INTRODUCTION
Since the industrial revolution, the rapid development of industry has caused various environmental problems that have global effects, including more intense El Nino and La Nina events, tsunamis, global warming, fine dust pollution, etc. Subsequently, developed countries have discussed on various environmental issues in the international conventions and made many rules. As a result of the London Convention Protocol 96, ocean dumping of all organic wastes have been banned and It took effect since 2009 in Korea. And animal manure ocean dumping has been banned from 2012 in Korea. For this reason, the demand for efficient methods of organic waste disposal has been steadily increasing.
Organic waste has been recycling through various technologies, it typically involves composting, anaerobic digestion (AD), biochar, and purification methods. These biomass recycling technologies break down organic matter or convert it into other forms and could reduce pathogens for biological safety [1,2]. Among them, bio-gasification converts organic matter, which consists of carbon, hydrogen, and oxygen, into biogas through the microorganisms under anaerobic conditions. The process of methane production classifies as hydrolysis, acidogenesis, acetogenesis, and methanogenesis [3] and they need organic substrates, which is consist of carbon and nitrogen, as a feedstock. Carbon type has an effect on microbiota during AD [4], it may lead to changes in the fermentation pattern. For this reason, the carbon type is very important in the methane generation process. Nitrogen must require for microbial growth, especially it is a basic component of amino acids, and it uses when synthesis for microbial protein. During the AD, carbon to nitrogen (C/N ratio) has effects on methane production yield and it is a very important factor for the stable operation. When C/N ratio is too high, biogas yield does not show the optimum due to acidogenic bacterium rapidly consume nitrogen compared with methanogenic bacteria. When C/N ratio is too low, most microbes rapidly consume nitrogen for growth. Although this has a positive effect on methane production rate. However, the lack of carbon type cause that decreases in acid forming, nitrogen accumulates in the form of ammonium ions (NH4) that increase the pH [5] which adversely affects biogas production.
In ruminant nutrition research, the concept of synchronization between the carbon and nitrogen sources has proposed to help stabilize rumen nutrient degradation by combining the different degradation rates of carbon and nitrogen [6]. Although the effect of synchronization seems to have little or no advantage for the aspect of nitrogen recycling in ruminants [7,8], this concept considered that might help control the shock by substrates due to no reabsorption of nitrogen in the AD, unlike rumen. However, in AD studies, there was a lack of research on the interaction between the carbon and nitrogen used as substrates.
Therefore, this study was conducted to evaluate the effects of different carbon types, and the interaction between carbon types and the C/N ratio, on methane production during the AD.
MATERIALS AND METHODS
The carbon types used in this study were selected starch (from corn, CAS Number 9005-25-8), cellulose (CAS Number 9004-34-6), and xylan (from beechwood, CAS Number 9014-63-5), and the nitrogen source was used urea (CAS Number 57-13-6) only, to regulate the ratio of C/N. Nitrogen source was fixed to evaluate the effect of carbon linkage characteristics and the interactions between carbon types and C/N ratios during the AD.
The experiment was performed using a completely randomized design; treatments were set to a 3 × 3 factorial design using three carbon types (starch, cellulose, and xylan) and three C/N ratios (10, 25, and 40) (Table 1). The AD was carried out using a 125-mL serum bottle at a constant mesophilic temperature (37°C) and it was performed as six replications to measure gas production, gas composition, and fermentation characteristics. A total of 132 bottles, which including 4 treatments × 3 replications × 11 sampling points, were prepared and the sample was collected gas and all inner material of bottles every sampling time. The inoculum, which was collected from the fed-batch type mesophilic anaerobic digester (37°C), was used in order to help with initial digestion. The digestor has produced about 200 mL-CH4/g-VS/d of biogas with an organic loading rate of 3 g-VS/L/d and a methane concentration of 60%. The chemical composition of inoculum on pH, total solids (TS) and volatile solids (VS) showed 7.86%, 12.31%, and 10.55% (of wet basis), respectively. Medium used according to the method of Chaney and Marbach [9] during the AD. The pH of the medium was adjusted to 7.1 using CO2 gas and stored at 37°C until performing the AD. The inoculum was injected 10% of the working volume into serum bottles according to the method of Healy and Young [10]. The sample was injected 0.25 g in each serum bottle, and the working volume was 50 mL. In BMP assay, calculated S/I ratio was 0.474 and the organic loading rate was 4.5 g-VS/L in each digestor. Nitrogen gas was flushed to make up anaerobic state in the digestors, AD was performed in a shaking incubator (IS-971R, Jeiotech Co., Korea) for 18 days, and it was shaken automatically at 0.336 ×g. Sampling was performed to measure gas production, gas composition, pH, and ammonia nitrogen content at 0, 1, 2, 3, 4, 5, 7, 9, 12, 15, and 18 days. Gas production was measured with a 50 and 100 mL glass syringe (Hypodermic Glass Syringe, DHS Medical Co., Korea) and gas samples were collected using gas tight syringe [Gastight model 1001 (22 gauge), Hamilton Co., Reno, USA] for analysis of gas composition.
Total solids and VS were determined by AHPA standard methods [11]. Element analysis of C, H, N, S, and O was measured using an elemental analyzer (EA 1110, CE Instruments, Italy). The pH was determined using a pH meter (Orion 420A+, Thermo electron Co., USA).
Gas production was determined using a 50 and 100 mL glass syringe by the method of Owen et al. [12]. The measured gas production was calibrated to standard temperature and pressure (STP 0°C, 1 atm) considering the temperature-dependent volume using the following calibration equation (1):
where V is the gas production at 0°C and 1 atm, Vat T°C is the gas production at T°C, T is the temperature at the time of volume measurement, P is pressure at the time of volume measurement, and Pw is the saturated water vapor pressure at T°C. The gas composition was determined using a gas chromatography (HP 6890, Hewlett-Packard Co., USA) with a thermal conductivity detector (TCD). The gas sample was injected 0.2 mL into the gas chromatography with a column temperature of 60°C, using helium as the carrier gas with a flow rate of 1.5 mL/min. The sample gas concentration was calibrated using a standard gas mixture consisting of 40% CH4-60% CO2 and 60% CH4-40% CO2.
The amount of methane production from the serum bottle was calibrated using the calibration equation (2) [13]:
where CCH4is the calibrated methane concentration (%), CCH4is the measured methane concentration (%), and CCO2 is the measured carbon dioxide concentration (%).
Total volatile solids (TVS) was consist of biodegradable volatile solids (BVS) and non-BVS. The residue of TVS (TVSe) after degradation was calculated using Equation (3), (4), and (5). In Equation (4), V0 mean calibrated gas production using Equation (1). The Ultimated biodegradability was calculated that the ratio of TVSe and initial total total volatile solids before degradation (TVS0) was ploted on Y axis and the reciprocal of the operating time (1/time) on the X axis by method of [14].
Biogas yield were simulated using the Gaussian and Gompertz equation during the AD. The specific methane yield was simulated using the modified Gaussian equation. This equation describes the daily methane yield in the batch-type digesters assuming that destruction of methanogens and microbial kinetic growth follow a normal distribution over the AD process [15]. The Gaussian equation is presented in Equation (3):
where y is the methane production rate (N mL g/ VS/ d); t (d) is the time over the digestion period; a (N mL g / VS / d) and b (day) are constants; and t0 (d) is the time where the maximal methane production rates occurred. The parameters of a, b, and t0 were extimated by using the “Solver” in MS Excel. The modified Gompertz equation is prestented in equation (7) [16]:
where M is the cumulative methane yield (N ∙ mL/g VS); P is the methane yield potential (P, N ∙ mL/g VS); Rm is the maximum methane production (N · mL/g VS); λ is the lag phase and t is the time based on the cumulative methane production M is calculated. The parameters of P, l, and Rm were estimated by using the “Solver” feature in MS Excel.
Data were analyzed using a MIXED procedure of SAS package program (SAS Inst. Inc., Cary, NC, USA) as a complete randomized design. The model was,
where μ is an average value, Ci is carbon type value, CNj is C/N ratio value and Eij(t) is the error value. The fixed effects are carbon type and C/N ratio in the procedure. Orthogonal contrasts were used to determine carbon type, C/N ratio and its interaction using CONTRAST option. Statistical difference and tendency were accepted at p-value less than 0.05 and 0.10, respectively.
RESULTS AND DISCUSSION
In the graphical statistical analysis, as shown in Fig. 1, the result of refractory fraction showed a different pattern among carbon types. The VS of the starch group decomposed faster than those of other groups during the start-up phase, it considers that caused by more containing non-structural carbohydrates [17]. As the previous study reported that cellulase and xylanase were more quickly increased on the xylan treatment compared with the cellulose treatment [18], and a similar result was obtained in this experiment. However, cellulose was more quickly degraded among other treatments from 2 days (<0.5 operating times), xylan degraded most slow until the end of digestion.
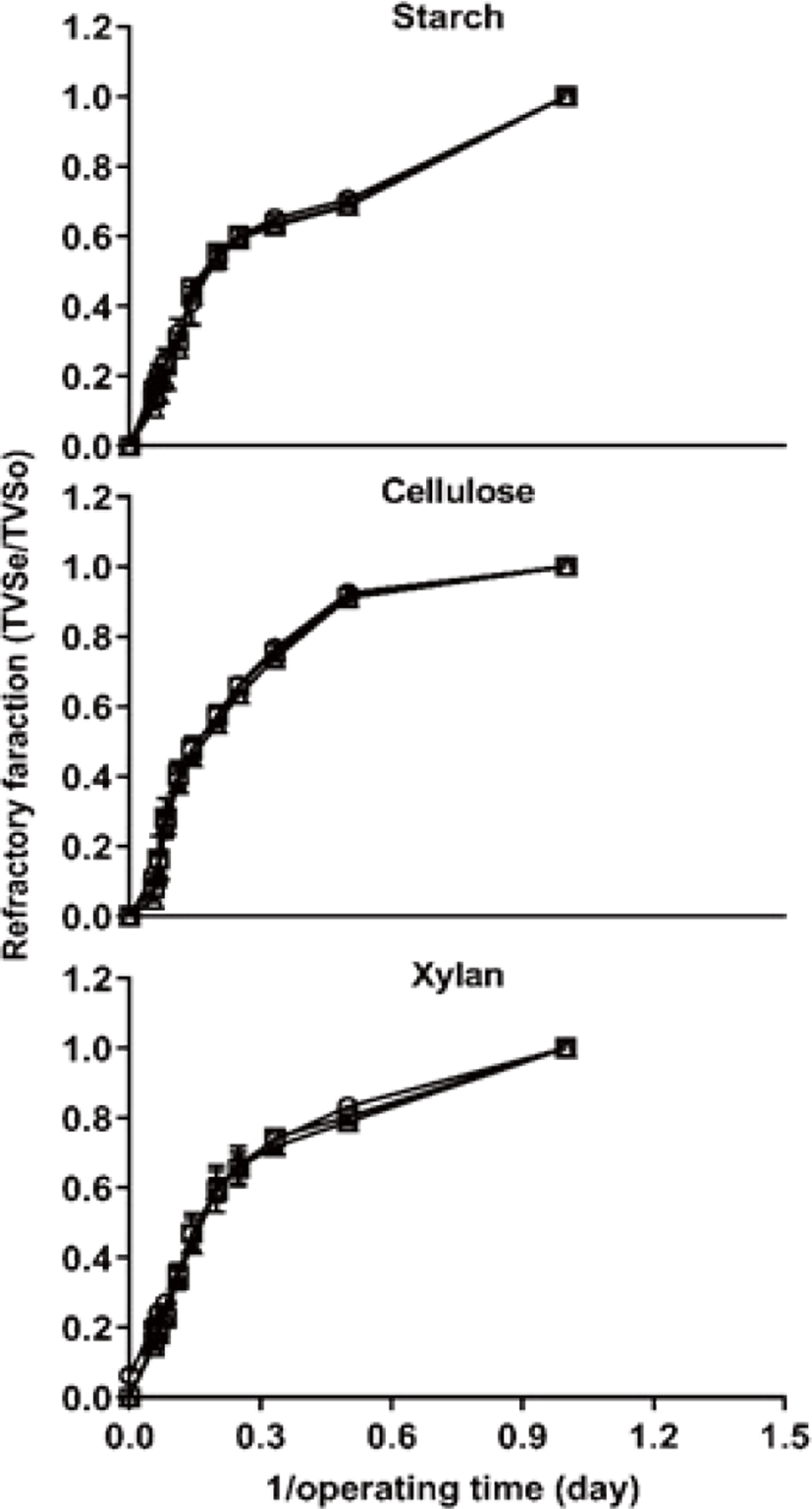
The degradation rate of cellulose has been reported as 89% [19] and 85% [20] at mesophilic temperatures, and the degradation rate of starch has been reported as 85% [20] at mesophilic temperatures. The degradation rate of xylan, conversely, has been reported as 65% [21] and 53% [22]. In this study, the degradation rate of starch, cellulose, and xylan showed greater than those of previous studies, it may be due to the refining substrate using in the experiments. And this suggests that the degradation of feedstock could be affected by processing, storage methods, and grain type [23]. The maximum degradation rate of cellulose was greater than the other treatments at 18 d (Table 2), which means that a sufficient pretreatment can improve the rate of cellulose degradation.
Carbon type | C/N ratio | Ultimate biodegradability (%) | Volatile solid removal (%) | Biodegradable volatile solids removal (%) |
---|---|---|---|---|
Starch | 10 | 100.0 | 82.7 | 82.7 |
25 | 99.2 | 86.4 | 87.1 | |
40 | 99.5 | 89.3 | 89.8 | |
Cellulose | 10 | 99.5 | 88.3 | 88.3 |
25 | 99.5 | 91.9 | 92.3 | |
40 | 99.5 | 95.4 | 95.9 | |
Xylan | 10 | 93.7 | 79.3 | 84.7 |
25 | 99.7 | 84.1 | 84.3 | |
40 | 99.9 | 85.5 | 85.6 | |
SEM | 0.44 | 1.19 | 1.11 | |
p-value1) | C | 0.001 | <0.001 | <0.001 |
CN | <0.001 | <0.001 | <0.001 | |
C × CN | <0.001 | 0.935 | 0.048 |
Although, in graphical statistical analysis, the interaction between carbon type and C/N ratio not showed clearly, the result of BVS showed the interaction which as increase C/N ratio of 10 to 40, BVS removal were greater during same periods AD (Table 2).
In the specific methane yield using the Gaussian curve fitting, as shown in Fig. 2, methane production showed different patterns among carbon types. The maximum daily methane production rate was similar between starch and xylan (Fig. 2), those of cellulose was slower compared with other treatments. Generally, non-structural carbohydrates decomposed faster than structural carbohydrates by microbes. However, in this study, the methane production rate of xylan treatment was similar to those of starch treatment during AD start-up phase. Furthermore, the maximal methane production occurred time, which t0 in Gaussian curve fitting value, was showed that it was similar between starch and xylan treatment (Table 3). In starch treatment, methane production pattern was different according to the C/N ratio, starch feeding might mean the factor which threatens stable methane production during the AD process. Furthermore, the property with rapid degradation of non-structural carbohydrates causes pH decrease, it could cause easily shock the AD process [24], and it is difficult to use easily as a carbon feedstock in an AD plant due to the high cost. For these reasons, many biogas plants have been used structural carbohydrates as carbon feedstock. Comprehensively, it suggests that proper pretreated fibrous materials could be a useful feedstock that has a similar methane production rate and more stable compare with non-structural carbohydrates.
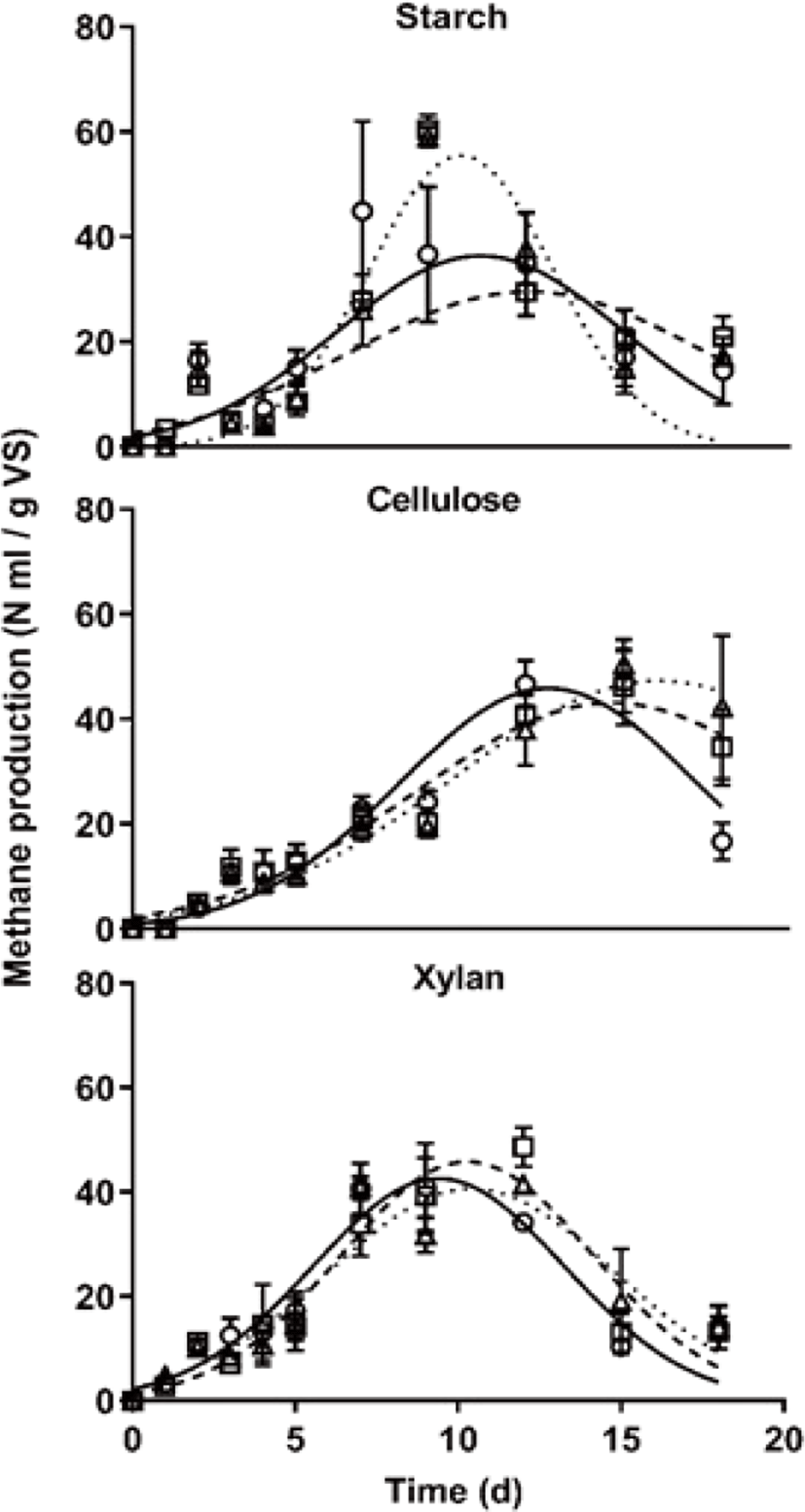
Carbon type | C/N ratio | Maximum specific methane yield (N mL/g VS/ day) | t0(days) | a (N mL/g VS day) | b (days) |
---|---|---|---|---|---|
Starch | 10 | 53.5 | 9.9 | 44.7 | 3.9 |
25 | 60.5 | 9.9 | 54.4 | 2.9 | |
40 | 59.1 | 10.0 | 56.5 | 2.8 | |
Cellulose | 10 | 50.5 | 12.7 | 46.1 | 4.5 |
25 | 47.3 | 14.9 | 44.1 | 6.1 | |
40 | 52.7 | 16.3 | 49.1 | 6.2 | |
Xylan | 10 | 42.2 | 9.4 | 43.1 | 3.9 |
25 | 48.7 | 10.3 | 46.8 | 3.8 | |
40 | 42.0 | 10.4 | 41.1 | 4.3 | |
SEM | 2.14 | 0.82 | 2.87 | 0.57 | |
p-value1) | C | 0.001 | 0.001 | 0.008 | 0.000 |
CN | 0.158 | 0.096 | 0.160 | 0.777 | |
C × CN | 0.054 | 0.330 | 0.096 | 0.154 |
The profile of simulated cumulative methane yield using the Gompertz curve is shown in Fig. 3 and determination coefficients (R2) were 0.99 for all curves. Cumulative methane production (M), Cumulative methane production potential (P), maximum methane production rate (Rm), and lag phase (λ) are listed in Table 4. Maximum cumulative methane production did not differ among treatments at 18 d (Fig. 3 and Table 4). Cumulative methane production potential showed the greatest in the cellulose treatment compared with other treatments (p < 0.05) and the lag phase showed that was mainly affected by carbon type (p < 0.05). The interaction between carbon type and C/N ratio significantly did not show in the methane production. The reason why the cumulative methane potential of cellulose treatment showed greater than other treatments, it considers because carbon content differed among each treatment and the Gompertz parameters were affected by the result of simulated using its data (Table 1). As Raposo et al. [20] reported that methane production was similar for starch and cellulose in AD under mesophilic temperature conditions using various inoculum, the methane production is expected to be similar under the same carbon content. For this reason, the maximum methane production considers that it might not differ according to the carbon type, and it only affected by the carbon content. However, the daily methane production pattern showed that it was affected by carbon type and C/N ratio (p < 0.05) (Rm in Table 4) as similar to the result of specific methane yield (Table 3). Interestingly, the noteworthy is that the lag phase was only affected by the carbon type (p < 0.05). Nitrogen content generally has a great effect during the overall AD process, the cumulative methane production yield of this study significantly did not differ by the C/N ratio among the treatments. Conclusively, when minimum nitrogen exists to grow microbes, these mean that the effect of nitrogen content on the methane yield might be very small.
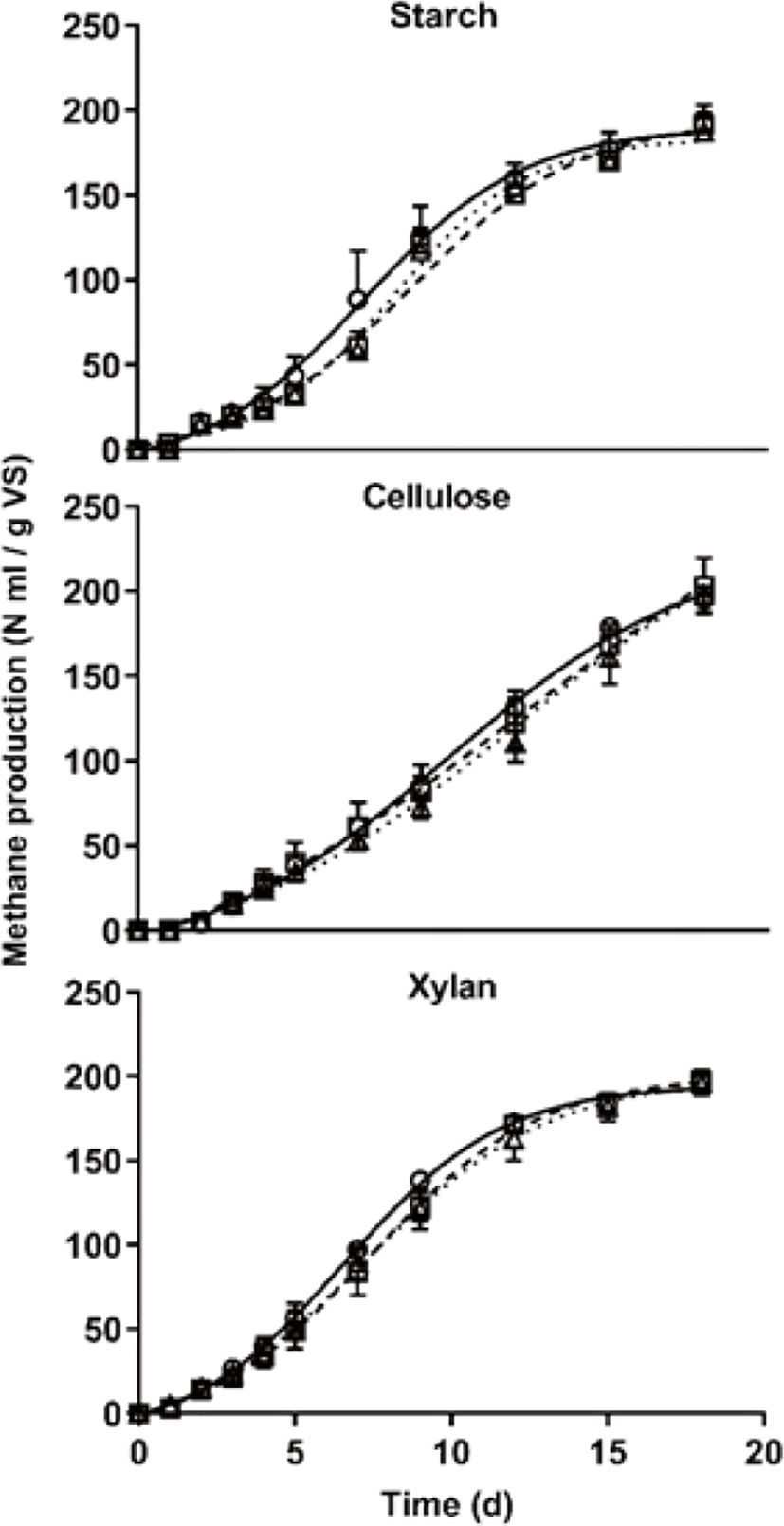
Carbon source | C/N ratio | M (N mL / g VS) | P (N mL / g VS) | Rm (N mL / g VS / day) | λ (days) |
---|---|---|---|---|---|
Starch | 10 | 191.3 | 198.4 | 20.1 | 2.7 |
25 | 191.8 | 205.2 | 18.1 | 3.0 | |
40 | 187.1 | 199.2 | 18.8 | 3.2 | |
Cellulose | 10 | 195.3 | 217.0 | 16.3 | 3.3 |
25 | 203.1 | 237.5 | 15.2 | 3.3 | |
40 | 233.0 | 223.2 | 14.5 | 3.5 | |
Xylan | 10 | 196.7 | 201.3 | 20.9 | 2.2 |
25 | 197.6 | 210.4 | 19.5 | 2.5 | |
40 | 195.8 | 208.5 | 18.6 | 2.3 | |
SEM | 5.12 | 5.61 | 0.77 | 0.23 | |
p-value1) | C | 0.123 | 0.001 | 0.001 | 0.000 |
CN | 0.627 | 0.022 | 0.020 | 0.304 | |
C × CN | 0.932 | 0.600 | 0.815 | 0.762 |
The pH content is shown in Fig. 4. during the AD start-up phase. During the experimental period, the minimum pH showed 6.85 and those of maximum pH showed 7.83. The pH is one of the important parameters to indicate stability during AD and methanogenesis is most active near pH 7 [25], On the other hand, it is inhibited in the condition of over pH 8 [26]. In this study, the pH change seems that it did not inhibit methane production process in all treatments. The pattern of pH change showed that has differed by carbon type, and it showed that did not significantly differ by the C/N ratio. Furthermore, as the pH of xylan treatment is less change than those of other treatments in the condition of a similar methane yield, it suggests that xylan is more stable and useful than starch and cellulose as feedstock.
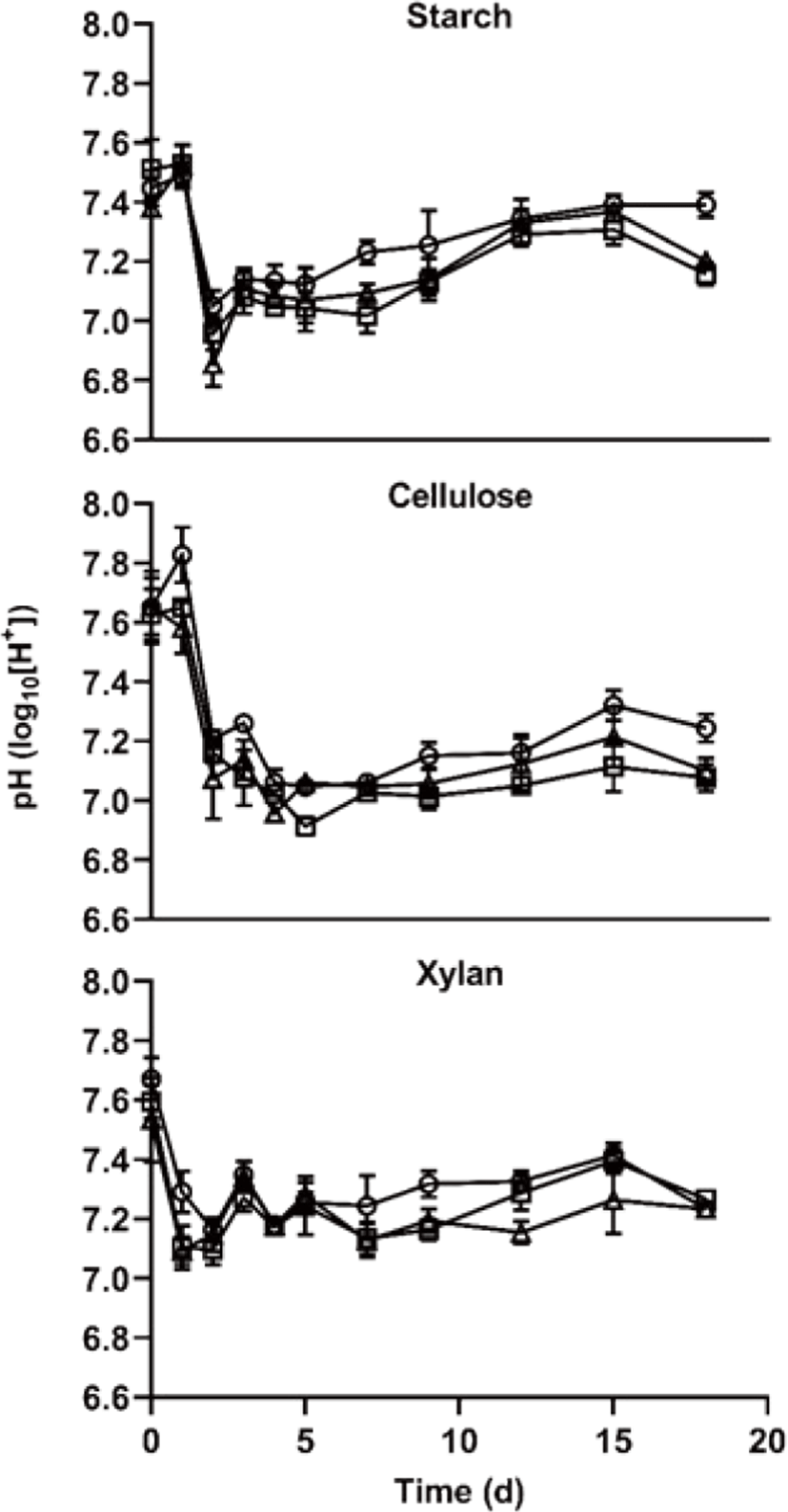
The ammonia nitrogen is shown in Fig. 5. during the AD start-up phase. High ammonia nitrogen concentration cause increasing pH content, it showed that inhibited AD process in the those of over 3,000 mg/L [27]. In this study, the ammonia nitrogen concentration was not enough to inhibit the AD process. A noteworthy phenomenon is that the ammonia nitrogen concentration was significantly higher in C/N 10 treatment than those of other treatments (p < 0.05). In this study, the nitrogen amount of C/N 10 treatment was higher 2.5 and 4 times than those of C/N 25 and C/N 40 treatment, respectively (Table 1). And, the carbon amount of C/N 10 was lower 5% and 6% than those of C/N 25 and C/N 40 treatment, respectively (Table 1), it differs very small amount compared with a difference of nitrogen amount. These results suggest that ammonia nitrogen concentration more depends on the amount of nitrogen, the carbon amount few effects on the ammonia nitrogen concentration. Furthermore, ammonia nitrogen concentration did not differ among the carbon type treatments of the same C/N ratio during the entire experimental period.
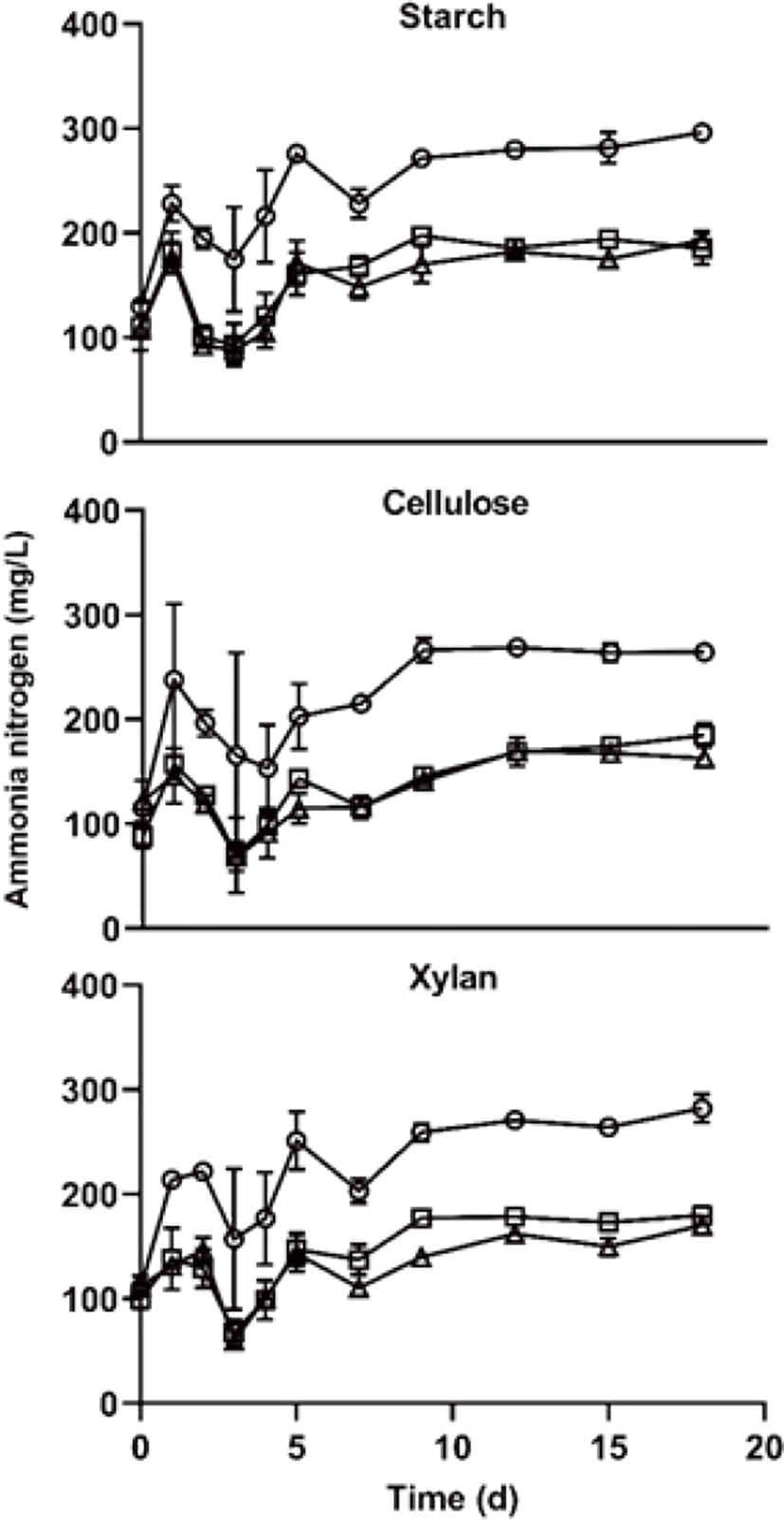
CONCLUSION
Carbon type affects various characteristics of AD during the startup phase. Maximum methane production was only affected by carbon content and the methane production rate was affected by both of carbon type and C/N ratio. Interestingly, the lag phase was only affected by the carbon type. However, the interaction between carbon type and the C/N ratio did not show during the experiment in all results. This research into carbon type characteristics is useful for the pretreatment and formulation of the substrate during the AD process as basic information and will contribute to improving the methane production rate of the start-up phase.