INTRODUCTION
Increased temperatures and heat waves due to the changing climate have negatively affected the animal industry [1,2]. In such conditions, livestock can suffer heat stress (HS) that may severely reduce carcass quality via reduced lean growth and increased fat content [3,4]. The HS reduces the growth rate of livestock by lowering feed intake and it causes an imbalance in physiological responses such as blood metabolic indicators, respiration rate, nutrient metabolism, and rectal temperature [5]. Consequently, HS can induce enormous economic loss, which is increasing annually with global warming [1].
Swine are susceptible to HS because of their naturally nonfunctional sweat glands and high metabolic rate due to genetic selection for high performance (reviewed by Ross et al. [6]). Sows are more vulnerable to HS given the appropriate rearing temperature of approximate 20°C. HS can potentially lead to abortion or early death in pregnant sows [7]. Early or late gestating sows exposed to HS produce fewer embryos that show lower survival as well as decreased litter sizes [8]. These results may be due to negative energy balance by low feed intake, which influence quality or development of oocyte, follicle and embryos by previous review [9]. In particular, pre- and post-mating periods to sows are important because ovulation rates are positively affected in uterine viable embryos [10] and in both size and average weight of litter [11]. Furthermore, gastrointestinal microflora is affected by HS, which facilitates the growth of pathogenic bacteria due to damage of the intestinal epithelium [2]. Importantly, intestinal microbiota is associated with physiology and reproductive performance in prenatal sows [12].
Many researchers have investigated strategies for mitigating the negative effects of HS [13]. As a potential management strategy, cooling systems can be used to regulate the inner air temperature of feeding facilities [14]. Air cooling (AC) systems are most widely used in farms, while water-drip cooling (WC) systems are also widely used and effective in farrowing crates or individual stalls to cool sows given that they improving respiration rate, heart rate, and rectal and skin temperatures [15]. However, studies related to cooling facilities have largely been limited to sows housed under HS during gestation. Therefore, in the present study, early gestating sows exposed to high-temperature stress were investigated. Specifically, the effects of AC and WC on respiration rate, body temperature, blood metabolites, reproductive performance, and intestinal microflora were determined in these sows.
MATERIALS AND METHODS
Cooling systems were prepared and applied in the gestating housing facility (individual stalls: 2.20 × 0.65 m) of National Institute of Animal Science (NIAS). An AC system comprising an air conditioner and duct was designed and installed to supply cold air above the head of sows in an individual stall. A WC system equipped with a water cooler and circulator was designed and installed to drop cold-water droplets (approximate 8°C) on the cervical area of sows via adjustable flexible nozzles with a water-drip flow controlled at 20 mL/min. The AC and WC treatments were operated when the ambient temperature measured at shoulder height of the sows was > 27°C. A treatment group exposed to HS without any cooling (denoted as the “HS” group) was used as a positive control; in this group, temperature was maintained at ≥ 30°C by a heater. The experimental facility was divided into three to avoiding interference effects among the treatment groups. Structural drawings of the AC and WC group set-ups are shown in Fig. 1.
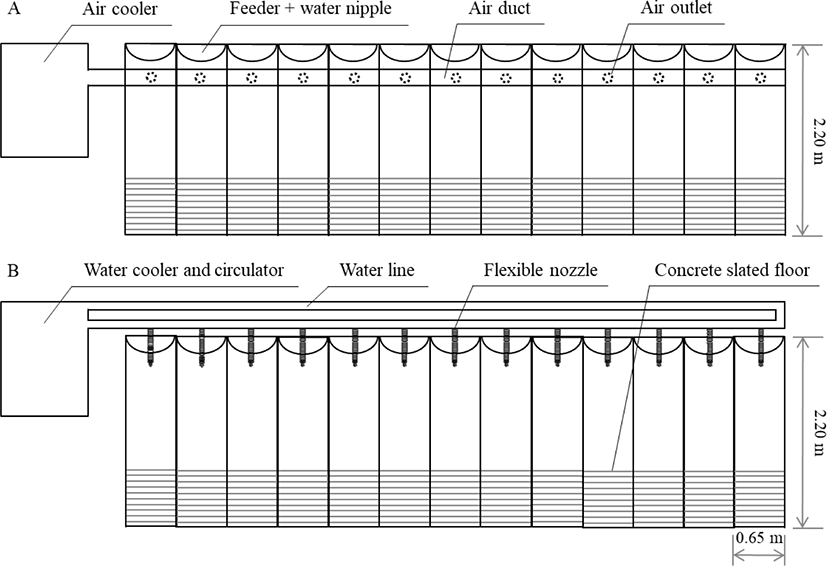
Thirty-nine sows (Landrace × Yorkshire) with two-parity were selected from the experimental farm of NIAS. These animals were randomly assigned to the three treatment groups as HS, AC, and WC and housed in individual stalls with 13 sows used per group. All sows were synchronized for estrus using altrenogest (Regu-Mate; Intervet America, Inc., Millsboro, DE, USA) for 18 days and maintained at ~25°C. When the onset of estrus was observed, the sows were artificially inseminated twice and then each cooler was operated from mating to day 35 of the early gestation stage (which corresponded to the summer season from June 25, 2019, to August 29, 2019). An experimental diet was prepared as Table 1. The allowance of the diet was 2.5 kg/d from day 0 to day 90 of pregnancy and 2.8 kg/d from day 91 to the farrowing days. For parturition, sows that had gestated for 104 d were transferred into farrowing crates. Also, water was freely accessed throughout the experimental period. The trial procedures were scrutinized and approved by the Institutional Animal Care and Use Committee of the NIAS (No. 2019-7131).
Provided the following quantities per kg of complete diet: Cu, 87.5 mg as copper sulfate; Fe, 125 mg as iron sulfate; I, 1.0 mg as potassium iodate; Mn, 75 mg as manganese sulfate; Se, 0.25 mg as sodium selenite; and Zn, 60 mg as zinc oxide.
Provided the following quantities per kg of complete diet: vitamin A, 12,500 IU; vitamin D3, 1,000 IU; vitamin E, 125 IU; vitamin K3, 6.3 mg; thiamin, 6.3 mg; riboflavin, 25.0 mg; pyridoxine, 12.5 mg; vitamin B12, 0.1 mg; pantothenic acid, 100 mg; folic acid, 7.5 mg; niacin, 225 mg; and biotin, 0.5 mg.
During the trial, dry bulb temperature (Tab) and relative humidity (RH) were automatically recorded every 30 min using a data logger (174-H, Testo SE & CO. KGaA, Lenzkirch, Germany), which was installed at the shoulder height of sows. The temperature–humidity index (THI) was identified by the equation from the NRC [16] that THI = (1.8 × Tdb + 32) − [(0.55 − 0.0055 × RH) × (1.8 × Tdb − 26.8)].
Backfat thickness was measured at day 0 and day 35 of gestation at the P2 location, which is 6.5 cm to the right and left of the backbone at the tenth rib, using a ultrasonic imaging tester (Medicalexpo, Loveland, CO, USA). Rectal temperature was identified using a Testo 180 thermistor probe (Testo SE & CO. KGaA). Skin temperature was visualized using a portable thermal imager (Testo 890, Testo SE & CO. KGaA), which had an operating temperature range of −15°C to 50°C with an accuracy of ±2% and a resolution of 640 × 480 pixels for capturing images. These images were analyzed using IRSoft version 4.5 software (Testo SE & CO. KGaA) to obtain average temperatures behind the ear, at the neck, and at the rump using a method of Rocha et al. [17]. Respiration rate was determined by counting the movements of the abdomen of sows during 1 min. The temperatures and respiration rate were measured on the last day of the trial.
Pregnancy tests were conducted on day 35 of gestation using ultrasonography (AV 2100V, Ambisea Tech, Shenzhen, China). The fertility rate was calculated as the percentage of pregnant sows from the number of total mated sows. On the terminal day of the experiment, three gestating sows per group were randomly selected and euthanized according to the guidelines of the NIAS. Corpus lutea and embryos were counted at the uterus opening. The viable embryos were calculated by dividing the number of corpus lutea by the number of embryos. At farrowing, the piglet’s total born, and those alive and dead, was identified. Mortality was calculated as the percentage of live animals from the total number of animals born per sow.
On the terminating day of the experiment, blood (n = 8 samples per group) was taken into serum tubes (SSTTM II advance, Becton Dickinson, Oxford, UK) from the jugular vein. For serum separation, the tube was centrifuged at 3,000×g and 4°C for 20 min. Serum aspartate aminotransferase (AST), alanine aminotransferase (ALT), total protein, blood urea nitrogen (BUN), and nonester fatty acids (NEFAs) were analyzed with a blood biochemical analyzer (ADVIA 120, Bayer,Leverkusen, Deutschland), and levels of thyroid hormones (T3 and T4) were measured using an electrochemiluminescent immunoassay method via a testing service from a clinical laboratory (Seegene Medical Foundation, Seoul, Korea).
Before and after HS treatments, fresh feces were collected from five animals per group by rectal massage and then rapidly frozen in liquid nitrogen. The samples were maintained at −80°C until further genomic DNA (gDNA) extraction. The gDNA from samples was extracted using G-spinTM Genomic DNA Extraction Kit (iNtRON Biotechnology, Seongnam, Korea).
A 16S rRNA sequencing targeting the V3 and V4 hypervariable regions were constructed according to a previous study [18]. The first PCR was performed using region-specific primers shown to have similarity with the Illumina index and sequencing adapters (forward primer:5′-TCGTCGGCAGCGTCAGATGTGTATAAGAGACAGCCTACGGGNGGCWGCAG-3′; reverse primer:5′-GTCTCGTGGGCTCGGAGATGTGTATAAGAGACAGGACTACHVGGGTATCTAATCC-3′). After magnetic bead-based purification of PCR products, a second PCR with limited cycles was performed using primers from a Nextera XT Index Kit (Illumina). The libraries were sequenced on the Miseq system (Illumina) with 300 bp paired-end reads. At the end of sequencing, the remaining reads were merged as paired-end reads using FLASH v 1.2.11. Merged reads containing more than two obscure nucleotides, those with a below of average score 20 (low-quality) or reads shorter than 300 bp, were filtered out. Potential chimeric sequences were detected using the Bellerophon method. The number of operational taxonomic units was calculated using the preprocessed reads from each sample and decided by clustering the sequences based on 97% sequence identities using QIIME software (v.1.8.0). Taxonomic abundance was identified according to RDP Classifier v1.1 with 0.8 of a confidence threshold in preprocessed reads from each sample. The composition of microorganism was normalized to the count of taxonomic abundance. Alpha diversity of each sample was estimated by the Shannon index. As beta diversity, the difference in microorganism composition was identified by Bray-Curtis distance. Principal coordinate analysis (PCoA) was then performed using the measured beta diversities.
Data were analyzed by using ANOVA or t-test of SPSS 20.0 (SPSS, Chicago, IL, USA). Animal was deemed as the experimental unit for reproductive performance, physiological parameters and microbial diversity. Statistical differences were determined with Turkey’s multiple range tests among treatment groups, whereas, in taxonomic abundances, pre- and post-HS exposition in each group were conducted by independent-sample t-test. Differences were considered significant when p was < 0.05.
RESULTS AND DISCUSSION
The average temperature, RH, and THI during the early gestation stage are shown in Table 2. Average temperature and THI were highest in the HS group (30.7°C and 83.9, respectively), but average RH was highest in the WC group (80.0%). Variation in daily temperature, RH, and THI during the experimental period is presented in Fig. 2. The daily temperature range in the HS and WC groups was 27.7°C–32.0°C, whereas that in the AC group was 27.1°C–29.0°C for 9 h from 10:30 to 19:30 and 25.6°C–27°C for 15 h (i.e., the remaining time). The daily variation in THI in the AC group was 76.1–80.1, whereas that in the WC and HS groups was 79.6–82.9 and 82.8–85.3, respectively. Temperature, RH, and THI are used as indexes of thermal comfort in livestock. HS is mainly caused by high ambient temperature but its effect are strengthened by RH [19]. The THI is widely used as indicator by which to estimate HS. According to the classification of Haeussermann et al. [20], THI can be graded in degrees such as normal (below 75), alert (75 to 78), dangerous (79 to 83), and emergency (above 84). Thus, according to this classification, the AC and WC treatments in the present study mitigated the stress caused by high ambient temperature to some extent.
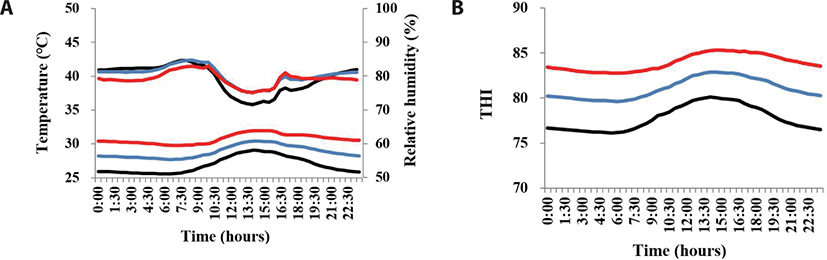
Sows in the AC group showed significantly lower respiration rates and rectal temperatures than were shown by the HS group (36 vs. 102 breaths/min and 38.2°C vs. 38.5°C, respectively; p < 0.05; Table 3). Similarly, skin temperatures for the ear, neck, and rump areas were significantly lower in the AC group than they were in either the HS or WC groups (p < 0.05). In addition, the WC group had lower neck temperatures than those recorded in the HS group (38.2°C vs. 38.9°C; p < 0.05). However, backfat thickness did not differ significantly among the groups at days 0 and 35 of the trial (data not shown). Previous researches are reported that hyperventilation and hyperthermia occur in pigs during heat waves. Heitman Jr et al. [7] reported a respiration rate of 157 breaths/min and rectal temperature of 40.6℃ in late pregnant sows kept with a maximum temperature of 35.8℃. Lactating sows reared with HS at 32°C showed increased respiration rates and rectal temperatures compared with pigs reared under thermoneutral (TN) conditions (21°C) [21]. Similarly, Zhao et al. [22] reported lower respiration rates (23 vs. 101 breaths/min) and skin temperatures (30.3°C vs. 36.6°C) in early-to-mid gestating sows kept at TN conditions of 20℃ compared with sows subjected to cyclic HS at 28°C–33℃. Another study reports lower respiration rate and temperature of ear and shoulder in pregnant sows under TN compared with HS [4]. These previous reports are similar to the results from the AC and HC groups in the present study. WC is often used in farrowing crates or individual stall to improve the respiration rate, heart rate, and rectal/skin temperatures of sows [23]. Murphy et al. [15] reported that the respiration rate was reduced by WC (63.6 vs. 28.5 breaths/min) in lactating sows housed with HS. Although there was no significant difference between the WC and HS groups in the current study, WC tended to improve the body responses of sows slightly. Furthermore, other cooling facilities as fogging, cooling pads and evaporative cooler show decrease of respiration rate and skin temperatures in lactating sows subjected to HS according to recent review [14]. Thus, the cooling systems seem to provide some thermal comfort to early gestating sows kept in high-temperature conditions.
The effect of the cooling systems on blood metabolites in early gestating sows exposed to high temperature are shown in Table 4. Total protein, ALT, AST, and NEFA in serum were not significantly affected by cooling systems. However, BUN was significantly increased in the WC group compared with its levels in the HS and AC groups (p < 0.05). In a previous study, growing pigs housed at 33°C showed decreased ALT or dynamic alteration of lipids in serum when compared with pigs housed at TN conditions of 23℃, but total protein and BUN are not differed between the treatments [24]. Another study reported that absorptive metabolism for energy and nitrogen was reduced in gilt pigs exposed to cyclic or constant HS [25]. Sanz Fermandez et al. [26] reported that NEFA immediately increased in pigs under HS (31.6°C) and paired-fed TN (PFTN, 21°C) conditions 1 day into a trial, before reaching a peak at 3 days, and then acutely decreasing; overall, however, pigs under HS showed 50% lower NEFA . In contrast, there are not different to NEFA in growing pigs [3] and gestating sows [4]. Previous results for lipid metabolism suggest that it is differentially controlled according HS and TN conditions [24]. Therefore, given the range of results reported, further study is required to clarify the metabolic mechanisms underlying the effects of HS in pigs. In the present study, triiodothyronine (T3) was significantly higher in the AC group than that in the HS group (p < 0.05; Table 4), whereas thyroxine (T4) levels only slightly increased in the AC and WC groups compared to in the HS group. Thyroid hormones are known to stimulate heat production via energy and lipid metabolism [3,27]. Indeed, pigs exposed to HS show a remarkable reduction in the hormones required to ameliorate heat stroke according to previous study [28] and review [29].
The cooling treatments used in this study did not altered in the fertility of sows at day 35 of gestation (Table 5). Similarly, as reproductive parameters, the number of corpus lutea and embryos, along with embryo survival rate, did not differ significantly among the treatment groups. Thus, reproductive performance at farrowing was not affected by the cooling systems. Similarly, Liao and Veum [25] reported that subjecting pigs to cyclic or constant HS in early pregnancy does not influence the parameters tested in our study. In addition, sows housed under TN conditions (20°C) rather than cyclic HS conditions (28°C–33°C) during 40–60 days of gestation were not affected in terms of corpus luteum number and embryo survival rate, but instead a numerical increase was observed in the TN group [22]. Omtvedt et al. [8] reported no effects on the performance of piglets produced from sows treated with HS during the mid gestating stage. However, HS is closely related to swine reproduction as it can (i) postpone the onset of puberty, (ii) compromise fertility, embryo development, and parturition rate, and (iii) reduce piglet performance [5,6,30]. This may be due to the insufficiency of the placenta according to results that show decreased placental nutrient transporter gene expression and epithelial layers [22]. Omtvedt et al. [8] also reported that HS at 32°C–38°C during days 0–8 or 8–16 of gestation can, in fact, reduce the number of corpus lutea and viable embryos in sows. In addition, these authors reported reduced litter performance in sows housed with HS during days 102–110 of gestation. The contrary results in these reports may have arisen for two reasons. First, HS sensitivity may differ according to pregnancy stage, i.e., early or late gestation are important periods because of embryo implantation and acute fetus growth. Second, genetic potential may be a factor; global breeding strategies typically focus on economically efficient selection, which can affect heat production due to increased physiological demand as the reproductive and lean growth cycle are accelerated in selected swine [6,31].
In the current study, the Shannon index was slightly decreased after HS relative to the value before HS (Fig. 3). Similarly, a significant decrease was observed in the index after HS compared with the index before HS with WC (5.2 vs. 5.6; p <0.05). According to taxonomic classification, however, microbial diversity at the phylum level was not changed by HS and cooling (Table 6). In contrast, after HS with AC and WC, microbial diversity at the family and genus levels was greater than before HS and before HS with AC (p < 0.05), but it did not differ simply before and after HS. At the species levels, after HS with AC or WC, microbial diversity was higher than before HS, after HS, and before HS with AC (p < 0.05). Intestinal microbes are closely related to the health of the host and play an essential role in the digestion and absorption of nutrients as well as in the development of immunity [24]. Indeed, balanced intestinal microflora can have beneficial effects on gut health by preventing the invasion of pathogenic bacteria and enhancing the function of the intestinal barrier [32]. In particular, increasing the diversity of intestinal microbes has a significant effect on host physiology and immune response [33], whereas decreasing diversity can lead to digestive disease [34]. Thus, according the present results, cooling systems can positively alter the diversity of intestinal microorganisms in sows housed under HS conditions.
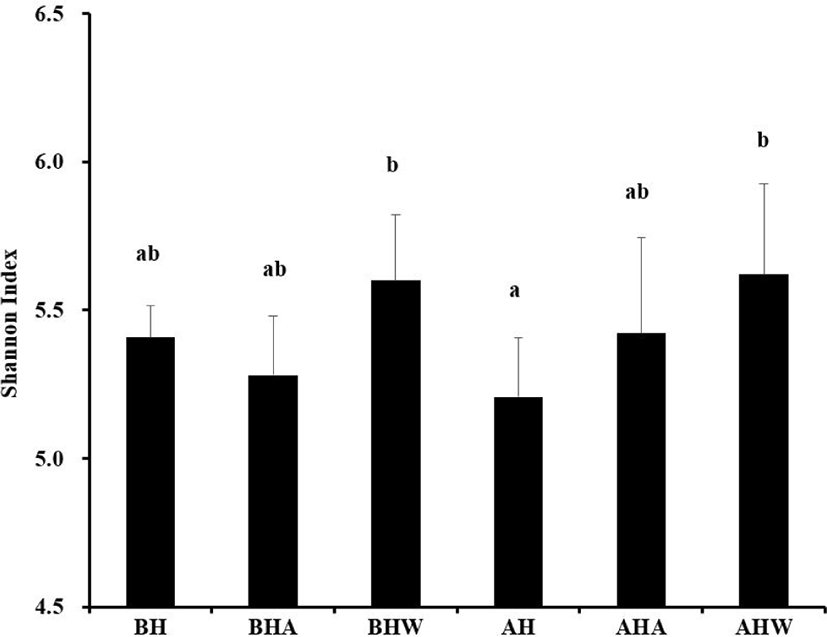
The overall structural changes in intestinal microbiota were analyzed using PCoA (Fig. 4). The PCoA plots for microbial communities before and after HS indicated that high-temperature conditions altered the intestinal microflora of sows. Specifically, after HS, patterns in the intestinal microbial community differed between the HS and WC groups. However, the intestinal microbial community in pigs from the AC group resembled those of the HS and WC groups. Certain PCoA plots in the WC group were also apparent in sows before HS, which may have arisen due to differences among sows rather than because of HS-related changes in intestinal microbial. As HS seemed to change the microbial community in the gut of sows, we further investigated the changes in microbial species.
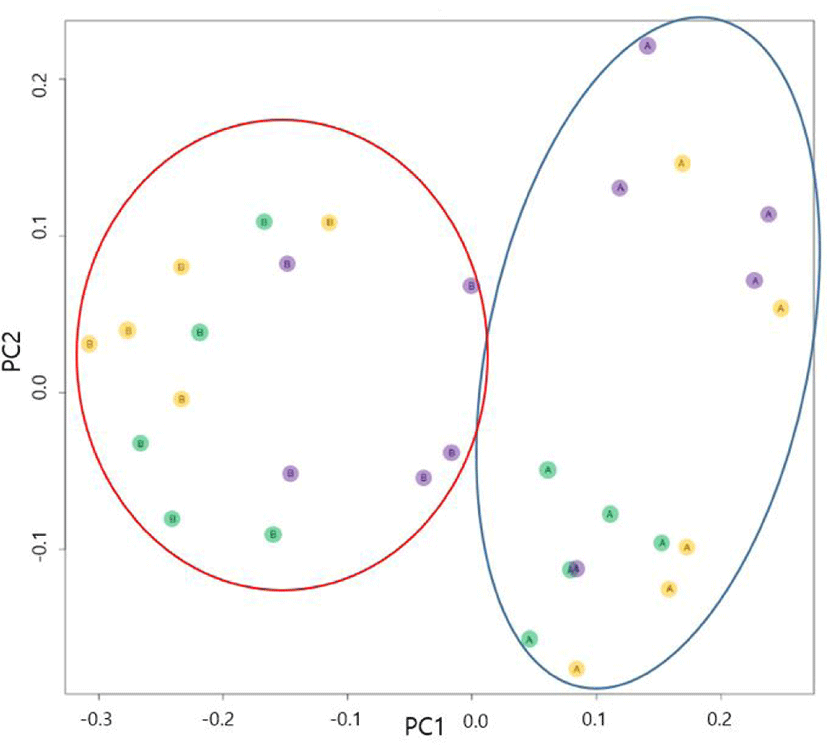
Specific HS-induced changes in intestinal microflora were identified in pregnant sows (Fig. 5): the distribution of intestinal microorganisms at the phylum level (Fig. 5A) and the proportion of different species in the intestinal microflora of pigs (Fig. 5B) were altered after HS. Levels of Bacteroidetes were not altered by HS, but those of Firmicutes decreased significantly after HS (54.15% of all phyla before HS vs. 37.16% after HS; p < 0.01). Compared with levels before HS, the AC and WC groups also showed significantly decreased proportions of Firmicutes after HS (p < 0.05 and p < 0.01, respectively). Levels of the harmful intestinal bacteria Spirochaetes increased significantly in all groups subjected to HS (p < 0.05). At the genus level (Fig. 5C), Treponema, a pathogenic microorganism, comprised 2.37% of the detected genera before HS but increased significantly to comprise 14.68 % of the detected genera after HS (p < 0.05). Similarly, the AC and WC groups showed a significant increase in Treponema after HS (p < 0.01). Another common genus, Prevotella was not affected by HS in sows nor by HS in the AC group; however, levels of Prevotella significantly decreased (p < 0.01) in the WC group. Notably, since the proportion of Prevotella did not differ significantly among groups after HS, it is presumed that Prevotella was more prevalent in groups other than WC before HS and that HS did not affect this genus.
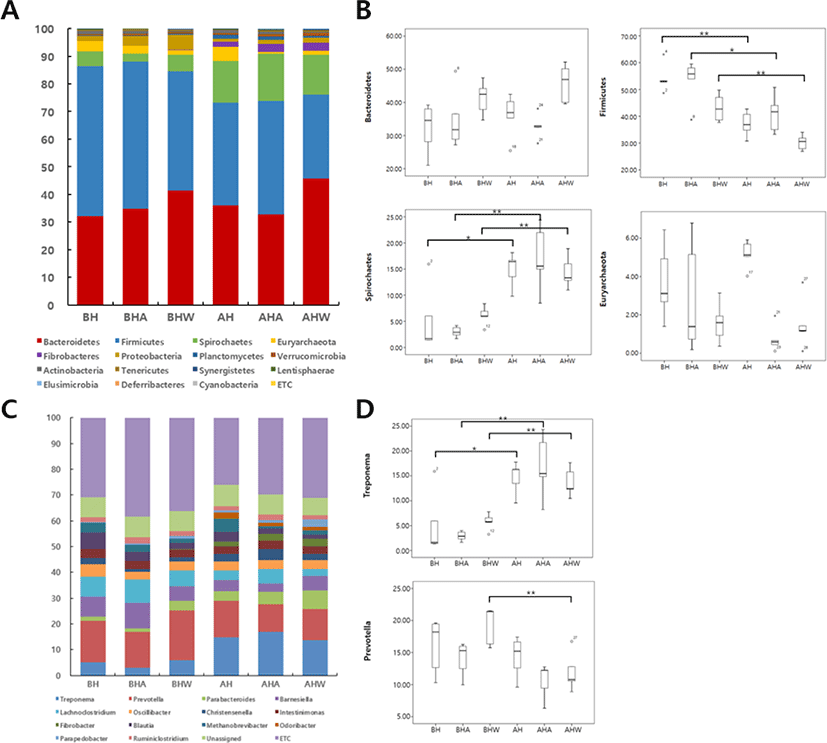
Firmicutes and Bacteroidetes are known to be dominant in the intestinal microflora of pigs and, phylogenetically, account for > 90% of the intestinal microflora in humans and mice [35]. In the present study, Firmicutes and Bacteroidetes were also dominant at the phylum level in sows. After Firmicutes and Bacteroidetes, the most dominant phylum was Spirochaetes, which increased in all groups subjected to HS. Spirochaetes found in pig intestines have long been recognized as disease-causing pathogens in humans and birds [36]. Euryarchaeota, another potentially harmful bacteria found in the intestines [37], was significantly reduced in the AC and WC groups compared with in the HS group. At the genus level, Prevotella species were dominant in the tested sows. Prevotella is commonly found in species that consume fiber diets [38] and is known to contribute to the breakdown of carbohydrates [39]. Also at the genus level, Treponema species increased to the greatest extent in the intestinal microflora of sows after HS. Treponema contains many species of pathogenic microorganisms [39]. Indeed, this genus is associated with various tumor system diseases related to syphilis in humans and dermatitis in cattle [40], and is known to cause dysentery in pigs [41]. In the current study, following HS, Treponema increased by 12% and 14% in the HS and AC groups, respectively, but increased by only 8% the WC group. In addition, the respiratory rate of sows was significantly reduced in the AC group, which may have had a minimal effect on intestinal microbial changes caused by heat shock. This is because the THI in the AC group was at a warning-risk level for 4 weeks and was only normal in the last week, which indicates that the recovery period was insufficient to restore the intestinal environment of sows exposed to HS. In comparison, the intestinal environment was not restored to normal levels (i.e., those before HS) in the WC group, although levels were still improved in relation to those in the HS group.
CONCLUSION
The swine industry is suffering substantial economic expenditure due to HS-related issues. Thus, using cooling systems on gestating sows with HS was evaluated here by measuring variables such as body (rectum and skin) temperature, respiration rate, thyroid hormone levels, reproductive performance, and the gut microbiome. Taken together, our results indicate that the AC treatment stabilized the biometric cycle of early pregnant sows exposed to HS but did not affect reproductive performance. In addition, the impact of HS on the diversity of gut microbiome was minimized by the use of cooling systems. Overall, our data suggest that AC or WC could ameliorate or mitigate, to some extent, the adverse effects of HS on various physiological parameters and the intestinal microbes of pregnant sows. However, current study may lack representation owing to relatively less animal number used. Therefore, further study can be needed to reevaluate effect of cooling system by using large scale or farm unit.