INTRODUCTION
The hormones cortisol and corticosterone have become increasingly important in animal research as key biological markers (biomarkers) of stress and distress. Selection and preparation of the appropriate biological matrix (biomatrix) is the first step in every analytical procedure in biological research and is especially important for evaluating response to chronic stress. Biomatrices can be divided into three types: fluid (e.g., blood, saliva, urine, milk), semi-solid (e.g., earwax, bone marrow, feces), and solid (e.g., hair, wool, feathers, nails, scales). Each of the aforementioned types has advantages and disadvantages, with its own specific properties. The aims of this review are the following: (1) to discuss the biomarkers of stress in different animal species; (2) to provide guidelines for selecting the appropriate biomatrix for studying chronic stress in animals according to composition, consistency, and preparation technique; and (3) to discuss the strengths and limitations of various laboratory techniques for analyzing stress hormone levels. In particular, the review considers the questions of which biomatrices most accurately represent stress levels and whether stress in animals can be evaluated accurately using only a single biomarker.
BIOMARKERS OF STRESS IN ANIMALS
Biomarkers are defined as biological molecules whose levels in various biomatrices are used to evaluate whether an organism’s condition is normal or abnormal [1]. When evaluating response to a particular stress, it is critical to identify the most reliable biomarker; its levels should be strongly correlated with the specific pathophysiological aspects of that stress [2]. In animals, stress causes the endocrine system [3] to release several hormones, such as epinephrine, norepinephrine, and cortisol [2,4,5]. In general, researchers selecting biomarkers should consider the following: (1) the ease of collecting and processing the required biological specimens; (2) the stability and durability of the marker throughout the storage and evaluation periods; and (3) the availability of assays with sufficient specificity and sensitivity to the markers selected [2]. Cortisol and corticosterone score well on these criteria and are considered the classical biomarkers of stress, used more widely in animal research than other biomarkers such as prolactin, serum amyloid A, and substance P.
In general, the term “stress” refers to a normal reaction of an animal’s body to any type of physiological change (e.g., fluctuations in heart or respiration rate), behavioral change (e.g., decreasing feed intake, changes in time spent standing or lying down), or stressor (e.g., changes in environment or nutrition) [6,7]. Once the animal’s central nervous system (CNS) processes the stress, four biological responses are triggered: (1) the autonomic nervous system response, (2) the neuroendocrine system response, (3) the immune system response, and (4) the behavioral response [8]. In this regard, a series of hormones are synthesized and then released into the bloodstream, influencing the sympathetic–adrenal–medullary axis, the hypothalamic–pituitary–adrenal (HPA) axis, inflammation, metabolism, and immune function [6,9]. The HPA axis is the primary system involved in the initiation and coordination of the stress response [6]. Activation of the HPA axis stimulates secretion of corticotropin-releasing hormone from the paraventricular nucleus, which then stimulates the anterior pituitary to release adrenocorticotropic hormone (ACTH) [6,10]. ACTH then stimulates the adrenal cortex to secrete cortisol, which is the principal glucocorticoid hormone in humans, non-human primates, and other large mammals [6,10]. In addition, the regulatory role of central HPA axis on the production of cortisol can be influenced by the peripheral HPA axis stimulation such as wound or dryness of skin [11,12]. Under normal body conditions, blood levels of both free and bound cortisol are subject to circadian rhythms, being highest in the early morning and decreasing throughout the day [13]. As cortisol is a biomarker of HPA axis activity and affects blood glucose levels, blood pressure, and other parameters, abnormally high cortisol is considered an indicator of physiological stress (acute or chronic). Many previous studies in different animal species have used cortisol as a biomarker of stress (Table 1).
Stress type | Species | Biomatrices |
---|---|---|
Acute | Cow | Blood [14], saliva [14,15], urine [16], milk [17], feces [18] |
Sheep | Blood [19], saliva [19], urine [20], feces [19] | |
Pig | Blood [21], saliva [22,23], urine [24], feces [25] | |
Fish | Blood [26], respiratory vapor [27] | |
Chronic | Cow | Hair [28], hooves and claws [29] |
Sheep | Wool [30] | |
Pig | Hair [31] | |
Fish | Scales [32], fins [33] |
Corticosterone is the main glucocorticoid hormone in birds and rodents, among others. Like cortisol, it is secreted by the adrenal cortex in response to stimulation by ACTH. Activation of the HPA axis in response to stressors results in increased blood corticosterone concentration, usually 1–2 min after exposure. Approximately 90%–95% of cortisol and corticosterone in blood is bound to protein (globulin or albumin) [34]; only the free steroids have ready access to the corticosteroid receptors in the target cells. Table 2 summarizes previous studies that measured corticosterone in different biomatrices as a biomarker of stress in different species. Measuring corticosterone levels can be useful for evaluating animal behavior and welfare, especially that of birds. For instance, rearing commercial broiler chickens under stressful conditions (e.g., high stock density) causes the secretion of corticosterone into the blood as an adaptive response [34,35], which can negatively impact their immune system, making them more prone to health risks such as severe infections; this can in turn cause health issues for consumers.
Multiple blood biomarkers respond to stress in different situations. Cortisol and corticosterone are the main stress hormones used to assess the level of stress in animals. However, according to some studies [42,43], prolactin can also be used as a biomarker of acute and chronic stress in fish, cattle, sheep, donkeys, rats, dogs, and humans. It is released from the pituitary gland in response to eating, mating, and other stimuli. In mammals, its main function is to stimulate the mammary gland to produce milk, and it also plays active roles in reproduction, homeostasis, and the immune response [42]. Serum levels of the protein amyloid A are elevated under stress, for instance in cattle [44,45], and can be used to study stress levels in early life. Other biomarkers that can be used as reliable stress indicators include lactate, glucose, and substance P [45].
STRESS AND COMMON STRESSORS
Both humans and animals may be subjected to acute or chronic stress by exposure to stressors. Stressors may be physical (e.g., fluctuations in air temperature and relative humidity, fluctuations in food and water availability, low blood sugar, injury, hemorrhage, transportation, painful procedures such as castration) or psychological (weaning, restraint, separation from peers and introduction to unknown animals, reproductive competition, nursing offspring) [10,34,46,47]. Thus, many different stressors may trigger acute or chronic stress reactions.
Acute stress reactions normally occur in response to a brief threat and are characterized by quick recovery of physiological balance, leading to complete adaptation [48–50]. During an acute stress response, the HPA axis is activated and hormones are secreted over a few seconds or minutes, resulting in physiological and metabolic changes such as increased heart rate, faster breathing, increased blood pressure, increased energy mobilization, stimulation of immune function, reduced appetite, reduced plasma potassium and magnesium, reduced digestive flow rate in the rumen and stomach, and increased digestive flow rate in the intestines [48].
In contrast to acute stress, chronic stress is a persistent physiological condition [51] that occurs when the body experiences several stressors or repeated acute stress, preventing the autonomic nervous system from activating normal physiological and behavioral adaptations [3,52]. Therefore, alteration of biological functions and induction of distress are expected during chronic stress. Such long-term over-stimulation of coping responses may result in direct effects, such as increased body heat, low energy, and anxiety, or indirect effects, such as functional changes in the endocrine, immune, and metabolic systems. These effects generally lead to prepathological or pathological consequences that reduce health and welfare [3,53].
The two types of stress are distinguished by duration and intensity. Stress lasting for a period of minutes to hours is classified as acute stress, whereas chronic stress persists for several hours per day, for weeks, or for months [54]. Previous animal studies evaluating responses to acute stress, such as castration and weaning, have measured cortisol concentration in various fluid biomatrices, such as plasma, serum, saliva, urine, and milk [34,55–57] (Table 1). However, evaluation of chronic stress (e.g., fluctuations in food and water availability, prolonged high temperature), especially in a single measurement, is a more complex process requiring careful assessment of the characteristics and limitations of the biomatrix from which stress biomarkers are to be extracted [58]. Measuring stress biomarkers in fluid biomatrices can only provide information about a single moment in time; however, this problem can be overcome by studying solid biomatrices such as hair, feathers, nails, and scales, which can provide information about changes in cortisol and corticosterone concentrations over time.
The main stressors of animals can be classified as environmental (e.g., toxic metal pollution, chemical fertilizer use, pesticide contamination), physiological (e.g., heat stress, cold stress, dehydration, advanced pregnancy), nutritional (e.g., acidosis, ketosis, hypocalcemia, hypomagnesemia, mycotoxins, and plant toxins), or management-related (handling and transportation, seasonal changes) [59]. Recently, much attention has been focused on heat and cold stress related to climate change, as climate change is a stressor common to all animals worldwide, as opposed to more localized nutritional and environmental factors. Researchers have begun to explore the influence of climatic and meteorological factors on the health and welfare of both animals and humans, particularly in the context of warming due to climate change. In commercial animal farming, environmental factors such as radiation, air movement, precipitation, air temperature, and relative humidity should all be considered to minimize the risk of heat stress [60–62]. Heat stress occurs when an animal’s heat load is higher than its ability to dissipate heat, damaging its general health; immunity, productivity, and welfare can be severely affected [62–64]. Heat stress triggers the release of stress hormones such as cortisol and epinephrine, which cause the body core temperature to rise further, weakening the immune system.
BIOMATRICES
To ensure good study design, the biomatrix needs to be selected and prepared carefully, as each type of biomatrix has its own properties that may complicate the study. In general, animal biomatrices can be classified as (1) fluid (blood, including serum and plasma; urine; saliva; sweat; milk), (2) semi-solid (earwax, bone marrow, feces), or (3) dry and solid (hair, feathers, wool, scales, nails, claws, hooves, teeth, and bone). Studies have shown that cortisol and corticosterone can be measured in all of the aforementioned biomatrices. To ensure the highest reliability and accuracy, biomatrix selection for a study should be based on physicochemical properties, biomatrix type (fluid, semi-solid, or solid), ease of collection, and method of analysis.
Cortisol and corticosterone can be measured in all types of fluid biomatrices because their chemical properties permit them to pass through all membranes of the body without hindrance [65]. It is normal for blood levels of these biomarkers to fluctuate throughout the day, and the time of sampling (morning or afternoon) should be taken into account when interpreting the results. Table 3 compares the properties of various fluid biomatrices commonly used for analysis of stress biomarkers in animals.
Properties | Fluid biomatrix | |||
---|---|---|---|---|
Blood | Saliva | Urine | Milk | |
Invasiveness of sampling | High [57,66], painful | Low [66], painless | Low [66], painless | Low [67], painless |
Risk of contamination from external sources | Low | High [66] | High | Low |
Training required for sampling | Yes [57,66] | No | No [66] | No |
Possibility of repeated sampling | Yes | Yes [68] | Yes | Yes |
Ethical and legal licenses required | Yes | Yes | Yes | No |
Biosecurity concerns | Yes | Yes | Yes | Yes |
Results depend on time of sampling | Yes | Yes [68] | Yes | Yes |
Results depend on sampling location | Yes | No | No | No |
Steroid medications may confound results | Yes | Yes [68] | Yes | Yes |
Sampling requires live animals | Yes | Yes | Yes | Yes |
Sample preparation is laborious and time consuming | Yes | Yes | Yes | Yes |
Storage conditions | Refrigerator, freezer [69] | Refrigerator, freezer | Refrigerator, freezer | Refrigerator, freezer [70] |
Time range represented by biomarker measurement | Single point, hour | Single point, hour | 12–24 h | 8–12 h |
Biomarker forms represented | Bound, unbound [66] | Unbound [66] | Unbound | Bound, unbound |
Sampling procedure may confound results | Possibly | Possibly | No | No |
Stress type | Acute [71] | Acute [71] | Acute [71] | Acute [70] |
Analytical cost | Relatively expensive | Relatively expensive [72] | Relatively expensive | Relatively expensive |
Blood is the biomatrix most frequently used for monitoring stress biomarkers (cortisol, corticosterone, and others). Only 5%–10% of blood cortisol is free and biologically active; the rest is bound to proteins such as corticosteroid-binding globulin [73]. When analyzing levels of stress biomarkers in blood, one should consider the amount to be collected, the centrifugation procedure if applicable (whether to separate plasma or serum, which anticoagulant to use), and storage conditions (room temperature, refrigerator, freezer, or otherwise). Generally, blood and other fluid biomatrices should be frozen until analysis. Plasma is the yellow part obtained after centrifuging blood with an anticoagulant (10 min at 1,000×g), whereas serum is obtained by centrifuging blood that has coagulated (10 min at 1,000−2,000×g); both can be stored frozen, preferably at either −20°C or −80°C [74].
Recent advances in technology have made it easier to measure cortisol from biological fluids other than blood, such as saliva. Measuring cortisol in saliva has numerous advantages over measuring it in blood. Like blood cortisol, salivary cortisol provides a measure of body cortisol levels within the past few minutes. However, unlike most cortisol in blood, salivary cortisol is free (not bound to other substances). The saliva matrix consists of 99.5% water, and sample collection with saliva-collecting devices is simple and non-invasive. Note that collection from rabid animals is inadvisable. Saliva samples can be stored at −20°C or −80°C [74].
Urine is another biomatrix commonly used to monitor stress biomarkers. Measuring urinary cortisol or corticosterone provides a measure of the secretion of these substances over 12–24 h. Urine is normally a clear or translucent fluid. It contains approximately 98% water [74], along with sodium, ammonia, phosphate, sulfate, urea, creatinine, proteins, and products processed by the kidney and liver, including drugs and metabolites [75,76]. Urinalysis is used in humans and animals to test for pregnancy, various disorders, and the presence of drugs in the body. The collection of urine from humans and most domestic animals is non-invasive and simple; however, collecting urine from wild animals may be difficult, as they are likely to be less cooperative. Under such circumstances, urine collection bags with catheters may be needed. Urine can be collected in plastic containers of a suitable volume, then stored at −20°C or −80°C [74].
Milk is a complex biomatrix to analyze due to its high content of nutrients, including proteins, and its variable composition, which may change throughout the nursing period. Mormède et al. [34] reported that cortisol levels in dairy milk are correlated with levels in blood; however, changes in milk composition could potentially affect the analysis results. Milk can only be collected from mature female animals, often only at a certain time of year, and cannot be collected from immature animals.
Generally, instruments for measuring stress biomarkers are designed to measure them in fluid biomatrices. Thus, semi-solid biomatrices may require dilution or dissolution before the measurement can be performed. Biomatrices consisting of soft to hard substances with high viscosity can be classified as semi-solid. Table 4 explains the properties of semi-solid biomatrices that can be used for measurement of stress biomarkers in animals.
Properties | Semi-solid biomatrix | |
---|---|---|
Feces | Earwax | |
Invasiveness of sampling | Low [77] | Low [77] |
Risk of contamination from external sources | Yes [78] | No |
Training required for sampling | No | Yes |
Sampling procedure may confound results | No | No |
Possibility of repeated sampling | Yes | No |
Ethical and legal licenses required | No [77] | Yes [79] |
Biosecurity concerns | Yes | Yes |
Results depend on time of sampling | Yes | No |
Results depend on sampling location | No | No |
Steroid medications may confound results | Yes | No |
Sampling requires live animals | Yes | Yes |
Sample preparation is laborious and time consuming | No | Yes |
Storage conditions | Refrigerator, freezer | Refrigerator, freezer |
Time range represented by biomarker measurement | 2–4 days | Week/month |
Biomarker forms represented | Unbound | Unbound |
Stress type | Acute | Chronic [77] |
Analytical cost | Relatively inexpensive | Relatively inexpensive |
Recent studies have introduced measurement of cortisol in earwax as a new method for monitoring chronic stress in humans [77] and whales [80]. Earwax collection is non-invasive and pain-free by comparison with the invasive sampling techniques required to collect many commonly used biomatrices [71,79].
Collection of fecal samples has the advantages of being non-invasive and not requiring ethical approval. As animals drop feces naturally, they can be collected without disturbing the animal [81], making sample collection easier than for other biomatrices. However, fecal samples contain a mixture of many hormones and enzymes at different stages of metabolism, which can interfere with the results. Furthermore, they may contain zoonotic pathogens such as Salmonella, which can increase one’s risk of catching an illness. Analysis of feces from mammals (both ruminant and non-ruminant) and birds is useful for assessing a variety of parameters, including long-term stress, seasonal hormone patterns, and pregnancy status. Feces are typically inhomogeneous, with a composition depending on diet, microbial activity, and the presence of digestive disorders. Feces can easily be collected in suitable bags and stored at −20°C or −80°C [74].
Measurement of stress biomarkers in solid biomatrices, for instance keratinized tissues such as hair and wool, has been validated as a method of monitoring chronic stress in animals such as rhesus macaques and ewes [30,82]. However, there is room to improve the accuracy of this method by further research into factors that influence marker levels in these biomatrices over the long term. Table 5 presents the properties of solid biomatrices that can be used for stress biomarker measurement in animals.
Properties | Solid biomatrix | |||
---|---|---|---|---|
Hair | Feathers | Nails/claws/ hooves | Scales | |
Invasiveness of sampling | Low [82,83] | Low | Low [71,84] | Low [71,85] |
Risk of contamination from external sources | Yes | Yes | No | No |
Training required for sampling procedure | No [57] | No [40] | No | No |
Sampling procedure may confound results | No | No | No | No |
Possibility of repeated sampling | Yes | Yes | Yes | No |
Ethical and legal licenses required | Yes | Yes [40] | No | Yes [85] |
Biosecurity concerns | Low | Low [86] | Low | Low |
Results depend on time of sampling | No [87] | No [40] | No | No |
Results depend on sampling location | Yes [57] | Yes | No | No |
Steroid medications may confound results | No | No | No | No |
Sampling requires live animals | No | No [86] | No | No |
Sample preparation is laborious and time consuming | Yes | Yes | Yes | Yes |
Storage conditions | Room temperature [87] | Room temperature [40] | Room temperature [84] | Refrigerator, freezer [85] |
Time range represented by biomarker measurement | Week, month, year | Week, month, year | Week, month, year | Week, month, year |
Biomarker forms represented | Unbound [88] | Unbound [88] | Unbound [88] | Unbound [88] |
Stress type | Chronic [82,83] | Chronic [67,86] | Chronic [71,84] | Chronic [71,85] |
Analytical cost | Relatively inexpensive [72] | Relatively inexpensive [40] | Relatively inexpensive | Relatively expensive |
Cirimele et al. [89] and Koren et al. [90] introduced methods for measuring cortisol in hair in order to monitor chronic stress response without the result being affected by sampling conditions, such as time of sampling, food intake, background stress levels at sampling time, and stress caused by sampling. Hair cortisol levels reflect blood levels during the period of the hair’s growth, enabling hair to capture information about how cortisol concentrations have changed over time and thus making it a useful biomatrix for monitoring animal welfare and health under exposure to environmental stressors [14].
All biomatrices have their own limitations with regard to sampling. Analysis of hair cortisol concentration is increasingly used to study HPA axis activity in response to prolonged stressful conditions and has proven to be a very informative tool for evaluating chronic stress and welfare in many animal species. However, the results can be influenced by numerous factors, such as genetic inheritance [91,92], species [93,94], physiological stage of development, life history, sex, age [94,95], environmental factors [67], pregnancy [95,96], and parturition [14], as well as methodological factors (storage time and conditions, localization of sample) and color [92]. Hair is a useful biomatrix for retrospective measurements of special analytes (e.g., cortisol) because small amounts of biological molecules present in blood can be deposited in it. Generally, there are two main possible pathways by which cortisol incorporation into hair shaft. One way is thought to be from the vascular system directly to the follicular cells that generate the hair shaft. Another way is through the diffusion of tissues surrounding the follicle of hair [55]. Due to lipophilic properties of cortisol, it is bound to carrier proteins to pass freely across the cellular membranes of target matrices. In humans, head hair grows at up to approximately 1.3 cm per month, whereas hair on other parts of the body grows very slowly. Hair samples can be stored at room temperature until analysis.
Like hair, feathers form a keratinized biomatrix. Previous studies have validated the measurement of stress biomarkers in feathers [35,40]. Bortolotti et al. [86,97] tracked long-term HPA axis activity in birds by measuring the corticosterone concentration in feathers. This method has the advantage of being able to determine current or past stress conditions, even in a dead bird [40].
In the majority of developed countries, human diets are undergoing a gradual shift from being primarily plant-based, with staples such as rice and other grains, to including significant amounts of animal products (e.g., meat, milk, eggs) [98]. Therefore, the demand for broiler chickens is expected to increase, and poultry farmers must prepare for possible challenges. For instance, feather cover plays a critical role in chicken health and welfare, particularly with regard to managing body temperature and protecting the body. Poor feather cover may lead to reduced mating drive and fertility, loss of body weight, and a poorer feed conversion ratio due to inability to regulate body temperature [99]. Feather loss and bald spots in chickens may be caused by environmental stressors (excessive heat or cold, ventilation problems) or imbalances in nutrition. In such cases, where stress threatens bird health, a method is needed to monitor stress-induced body changes in the birds. Measurement of corticosterone in feathers is a suitable alternative to commonly used techniques and can be used to evaluate stress over several weeks or months [86].
Taking feather samples from birds is quick and simple in comparison to blood sampling [40], with the advantages of not requiring expert training, reducing average handling time, and reducing stress on the birds during sampling. Furthermore, blood corticosterone can change quickly in response to environmental stressors or handling, whereas such variation does not occur as quickly in feathers. Another advantage is that feathers can be sampled from dead birds as well as live ones. Feathers can be stored at room temperature, and biomarker measurements taken from feathers are more accurate because of the reduced effect of conditions at the time of sampling [40,100]. A study on pigeons (Columba livia domestica) by Jenni‐Eiermann et al. [101] reported that the amount of corticosterone per millimeter length of feather varied between feather generations in interaction with segment, also finding that levels differed between washed and unwashed feather parts. In a study of broiler chickens, Carbajal et al. [102] found no significant relationship between feather corticosterone levels and physiological variables such as sex, weight, and the presence of fault bars. Lattin et al. [103] stated that the mechanism by which corticosterone is incorporated into feathers is not clear, suggesting that it may be deposited from blood during feather growth and keratinization. Another possibility is that it may be deposited via preen oil or diffusion from the skin surrounding the feather. Steroid hormones are lipophilic and low molecular weight substances that are synthesized by endocrine glands, and are then released into blood circulation. They act both on peripheral target matrices and the CNS. Because of lipophilic properties of steroids, up to approximately 90% of circulating cortisol or corticosterone is bound to carrier proteins such as albumin and corticosteroid binding globulin that can freely diffuse across the cellular membranes [34]. Thus, in birds, circulating corticosterone is incorporated into developing feathers via diffusion from the blood during cell differentiation.
Nails, claws, and hooves are keratinized tissues like hair, and they can similarly be used to assess the level of biological compounds such as cortisol [104]. Biological molecules present in blood can be deposited in these tissues, and their slow growth makes them useful for tracking changes in biomarker levels over month-long periods. Nails grow 3 mm per month in humans [104], whereas Contreras et al. [84] reported that over a three-week period, the front claws of cats grow by 2.4 mm and the hind claws by 1.7 mm. Binz et al. [105] reported different levels of cortisol in the nails of the left and right hands in humans; however, Higashi et al. [106] reported no significant differences between the left and right hands. The advantage of measuring cortisol in fingernails or toenails is that samples can be collected easily and with minimal stress using nail clippers and hoof cutters for humans, chickens, cats, dogs, cattle, sheep, goats, and pigs, then stored for a long time at room temperature.
Most fish species have scales distributed over their skin. Analysis of biomarkers in scales has been used to evaluate the activity of the hypothalamic–pituitary–interrenal axis in fish [85,107]. Scales can be easily and quickly sampled with negligible injury and stress to the fish, minimizing changes in cortisol concentration due to sampling stress [85,108]. Measuring cortisol in scales is a suitable method for evaluating chronic stress in fish, preferable to measuring it in other matrices such as feces, mucus, gut contents, and fins.
In animal research, samples should be collected and prepared in a manner that ensures data reliability. Before the experiment, animal names, identification numbers, the sampling location, the sampling facility, and the date should be properly recorded. When collecting multiple samples of fluid biomatrices per day, it is important to record the time of sampling due to daily variations in the concentrations of hormones (e.g., cortisol). To minimize risk to researchers, proper health and safety guidelines should be followed, and blood and other biomatrices should be handled properly during collection and storage.
In order to accurately determine levels of stress biomarkers in biomatrices, extraction procedures should be performed prior to detection by enzyme immunoassay (EIA). Extraction is a way to remove or concentrate the components of interest (e.g., cortisol and corticosterone) from the other components of the biomatrix (e.g., blood, urine, milk, hair, feathers) by applying appropriate solvents. For extracting stress biomarkers from fluid and solid biomatrices, the most widely used techniques are liquid–liquid and solid–liquid extraction with typical solvents such as diethyl ether and methanol. In the standard protocol for biomarker extraction from fluid biomatrices, the sample is diluted with ether (1:10 v/v) and vortexed for 1 min, followed by freezing. The ether supernatant (top layer) is then separated into a new container and saved. This procedure may need to be repeated multiple times for full extraction; each time, the top layers should be combined, and then the collected supernatant should be evaporated to dryness. To ensure reconstitution, the dissolved dried extract should be vortexed well together with an appropriate amount of the assay buffer provided in the EIA kit. Next, the EIA procedure may be continued according to protocol, or the samples may be stored at −20°C until analysis. Finally, the samples should be transferred to a 96-well microtiter plate, and the optical density at 450 nm can be determined using a standard plate reader (the wavelength may differ depending on the EIA kit). Curves for optical standards and experimental samples should be calculated with the analysis software included with the plate reader; alternatively, they can be calculated directly from raw plate data using free data analysis software that can be found online at www.MyAssay.com or other immunoassay analysis websites.
Commonly, the choice of techniques for screening stress biomarkers are not specific matter due to all well-known assays can apply to determine analyte purpose. Accordingly, Meyer et al. [55] mentioned a similar conclusion that the choice of assay is not particularly important as long as it has the requisite specificity for cortisol and a degree of sensitivity appropriate for the size of the samples being analyzed. Various assays can be used for evaluating cortisol and corticosterone concentrations in all types of biomatrices (e.g., high-performance liquid chromatography with mass spectrometry, fluorescence detection, immunoassays); however, immunoassays are the most commonly used method, based on binding between an analyte and an antibody. There are a variety of immunoassay methods, such as enzyme-linked immunosorbent assay (ELISA), radioimmunoassay (RIA), EIA, and the multiplex immunoassay. Each of these has limitations. For instance, RIA is an old assay now rarely used due to the risk posed by radioactive substances, the high level of skill and knowledge required compared with ELISA, and government restrictions due to environmental and safety concerns [33,109]. ELISA is designed to detect a single analyte (e.g., cortisol) in a single biomatrix; thus, for parallel analysis, several experiments are required, which is time- and cost-inefficient and can magnify errors in the results. The multiplex immunoassay can measure multiple biomarkers simultaneously in a single experiment [110] and may utilize flow cytometry, chemiluminescence, or electrochemiluminiscence [111]. The traditional EIA, involving an enzyme attached to an antibody, is capable of measuring one analyte (e.g., cortisol) in one biomatrix (e.g., hair) at a time; however, the multiplex method permits measurement of multiple analytes (e.g., cortisol, estrogen, oxytocin) in the same biomatrix (e.g., hair) at the same time. With traditional singleplex ELISA, it can be complicated to determine how different biomarkers, such as cortisol, cortisone, and estrogen, interact with each other; in comparison, the multiplex immunoassay saves time and expense and produces more biologically informative results. The current review is summarized with regard to measurement of stress hormones in several types of biomatrices (Fig. 1).
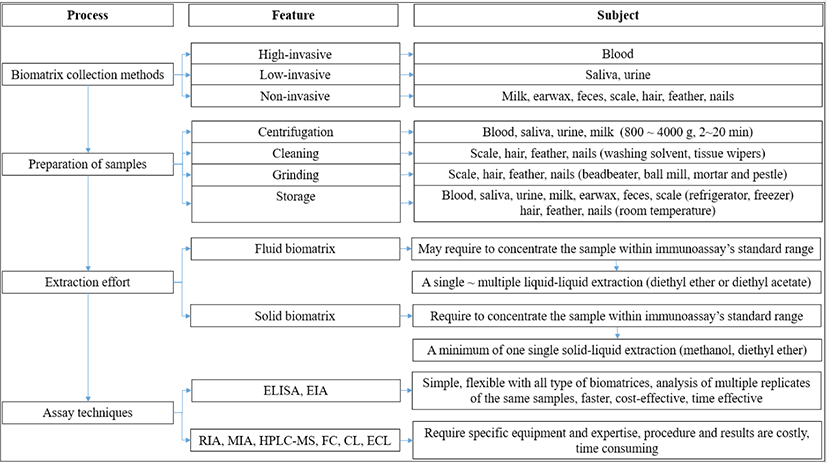
CONCLUSION
Measurement of stress hormones is an efficient method for evaluating stress, as it enables accurate information about physiological conditions to be obtained for living and even dead animals. In studies of chronic stress, the proper selection of biomatrices and biomarkers depends on the type of information required. Solid biomatrices can provide information about how concentrations of major biomarkers of stress (cortisol or corticosterone) have changed over time, making them more suitable than fluid biomatrices for this purpose. Commonly, cortisol or corticosterone extraction from solid biomatrices such as hair and feather, using methanol or diethyl ether that usually used for fluid biomatrices such as plasma and milk. Although, saliva, urine can be assayed after diluting them with sample preparation buffer, the extraction should be considered for accurate screening of cortisol or corticosterone via immunoassay methods. A single solid-liquid extraction procedure by methanol is sufficient to extract cortisol or corticosterone from solid biomatrices. Ideally, multiple (at least 3 times) extractions with diethyl ether are required to maximize the extraction of cortisol or corticosterone from biomatrices. Furthermore, solid biomatrices require less frequent sampling than fluid and semi-solid biomatrices, which saves time and expense and reduces error. At the analysis stage, among a variety of analytical methods used to detect and quantify cortisol and corticosterone in biomatrices, ELISA method is more applicable, highly sensitive, and reliable for screening those analytes than other analytical methods due to the simple procedure (requires no special expertise), analysis of multiple replicates of the same samples on one microtiter plate and faster with results in up to 3 hours, cost-effective, and flexible with biomatrix types. However, the use of a multiplex immunoassay can provide comprehensive details regarding interactions and correlations between various stress biomarkers in a single biomatrix.