INTRODUCTION
The ongoing threat of pathogenic bacteria has persisted throughout human history, and recent advancements in public health awareness and hygiene practices were expected to alleviate infections caused by these microbes [1]. However, the invasion of bacteria from the external environment, beyond human control, has remained challenging in terms of prevention [2-4]. In particular, damages caused by livestock (swine, chicken, and bovine) closely associated with human dietary habits and escalating issues involving companion animals (canines and felines) worldwide present an ongoing challenge that requires resolution [5-8].
The most effective and straightforward method for treating bacterial infections is the use of antibiotics specifically tailored to the bacteria in question [9,10]. However, since the initial discovery of antibiotics, their potent effects have led to global misuse, resulting in various side effects and contributing to substantial environmental pollution and public health concerns. Of these issues, the emergence of antibiotic-resistant bacteria poses the most serious threat [11,12]. In particular, multidrug-resistant bacteria, resistant to two or more antibiotics, require high antibiotic concentrations or additional administration of different antibiotics for treatment, exacerbating the situation [13]. Therefore, while proper antibiotic use to minimize the emergence of resistant strains and active infection management is crucial, the urgent development of antibiotic alternatives is paramount.
Among various alternatives to antibiotic substances, such as probiotics, organic acids, bacteriocins, and antimicrobial peptides, we have focused on the potential of bacteriophages as antimicrobial agents (Fig. 1) [9,14-17]. In contrast to indiscriminate bombardment by antibiotics, bacteriophages (phages) possess characteristics akin to guided missiles, selectively infecting and killing specific bacteria (Fig. 2). Some phages exhibit distinct specificity, infecting only a single species of bacteria, whereas others can infect multiple species. In either case, the bacteria serving as hosts are closely related taxonomically [18]. Virulent phages carry only a lytic life cycle, invading the host’s interior, replicating, assembling, and ultimately destroying host cells through release. It is estimated that there are approximately 1031 phages on earth, surpassing the number of bacteria. The specificity, prolificacy, and abundance of phages distinguish them from other antibiotic alternatives, garnering renewed attention as substitutes for antibiotics (Table 1) [19].
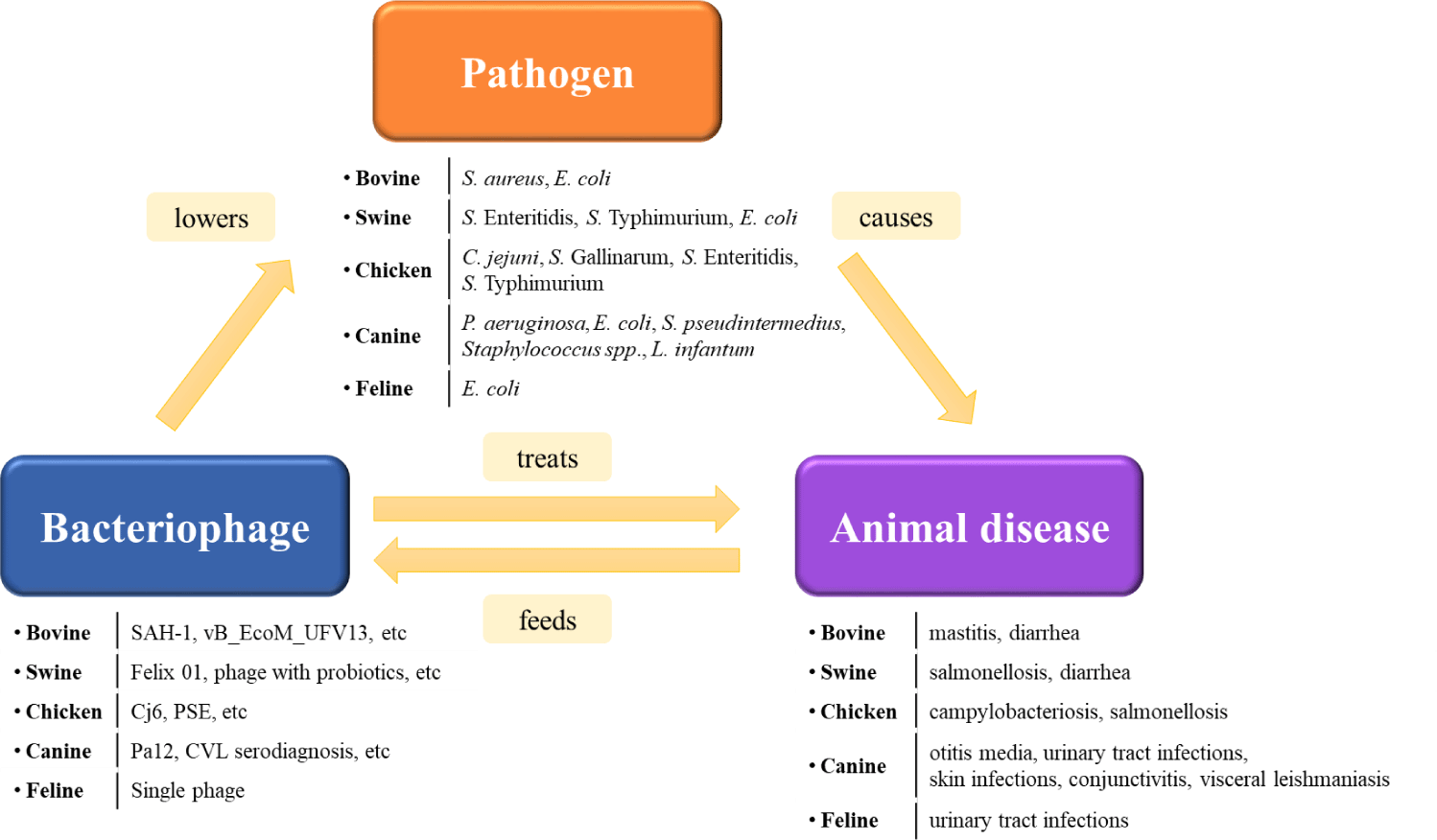
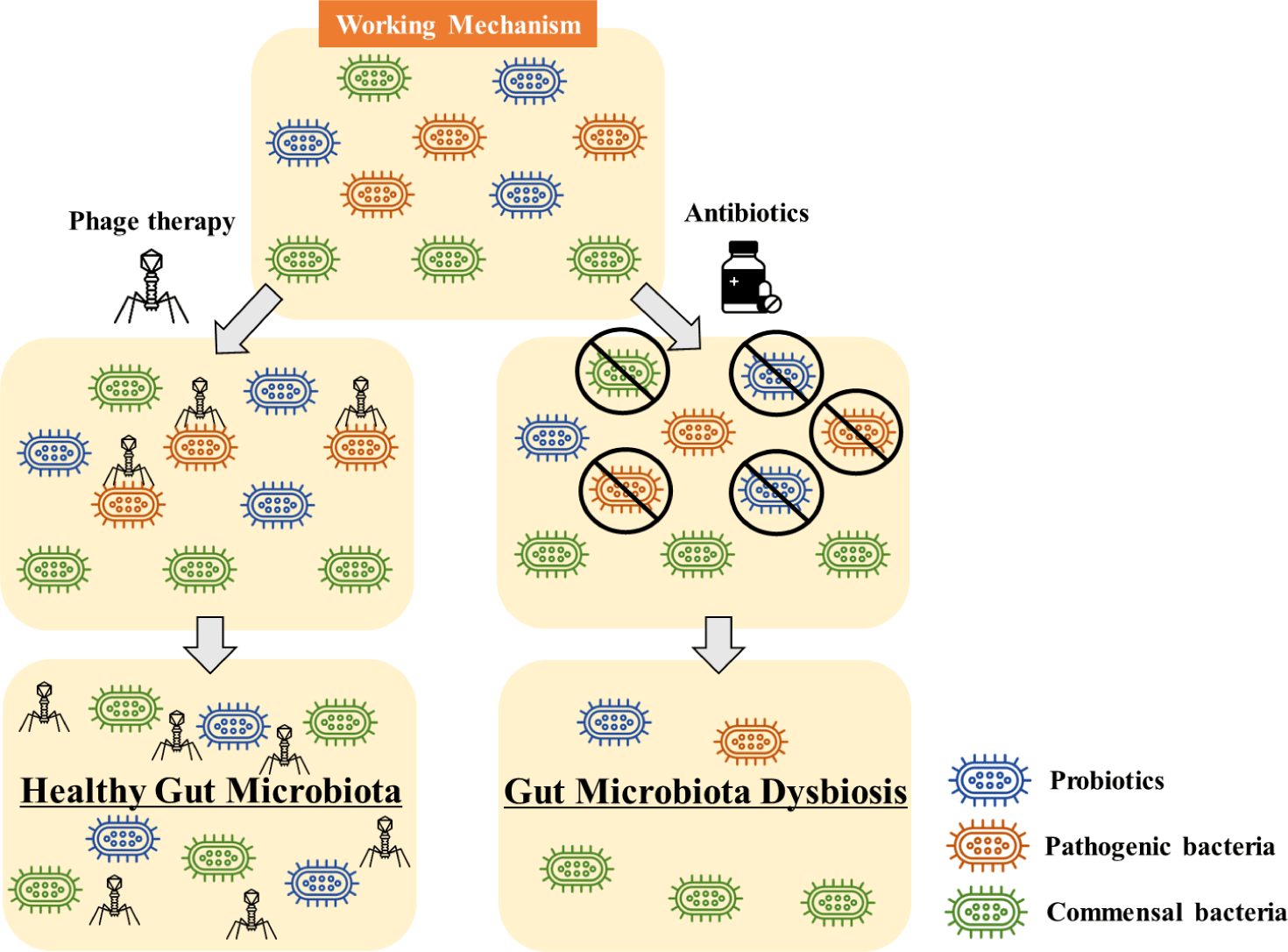
In an environment where pathogenic bacteria are prevalent, such as the livestock environment, the need for antibiotics is deemed critical [20]. However, there is a global trend toward the prohibition of antibiotic use in the livestock industry. Despite this trend, the prevalence of antibiotic-resistant strains continues to rise, attributed to the persistent use of antibiotics for purposes such as the treatment of bacterial diseases [21]. To address these challenges, experimental studies using phages for the inhibition of various pathogenic bacteria are underway (Table 2) [22]. On a national scale, legal permissions have been granted for the use of antibiotic alternatives within the livestock industry as veterinary drugs to mitigate these issues.
Animals | Target bacteria | Symptoms | Phage application | Therapeutic activity | References |
---|---|---|---|---|---|
Bovine | S. aureus (MDR) | Mastitis | SAH-1 | Significantly reduced bacterial growth at MOIs of 1–100 | [111] |
S. aureus (MRSA) | Six phage cocktails | Reduced S. aureus CFU counts by 64%–95% | [112] | ||
S. aureus (MSSA, MRSA VISA) | Five phage cocktails | Reduced colonization in the mouse mammary gland 8 h after treatment and prophylactically 4 h before challenge was most effective | [113] | ||
S. aureus (MRSA) | PhiSA012, PhiSA039 | Intravenous and intraperitoneal administration of SA012 reduced bacterial colonization and inflammation of the mammary gland. | [114-116] | ||
S. aureus (MRSA) | vB_SauM_SDQ | Lysed 20 of the 24 strains reduced established biofilms on polystyrene, milk, and mammary gland tissue after treatment. | [117] | ||
S. aureus (MRSA) | Phage 24 A2 | Lysed 19 of the 30 strains examined. Phage-cleared bacterial cultures on agar at a MOI of 10, supporting topical application for therapeutic use | [118] | ||
S. aureus (MRSA) | SLPW | Lysed 36 of the 40 isolates examined. Phage administration remedially reduced colonization and inflammation of cytokines in mice. | [119] | ||
E. coli (MDR) | Diarrhea | Three-phage cocktails | A cocktail of the phages reduced colonization, somatic cells, and inflammatory factors and alleviated symptoms of mastitis in cattle. | [42] | |
E. coli (MPEC) | vB_EcoM_UFV13 | A 10-fold reduction in bacterial load was observed at a MOI of 10 in mice. | [120] | ||
E. coli (ETEC, EPEC) | Three-phage cocktails | The probiotic-phage suppositories reduced the duration of diarrhea in calves, completely eliminating it within 24–48 h after use. | [42] | ||
E. coli (ETEC) | VTCCBPA9 | VTCCBPA9 showed bactericidal activity against 47.3% (62/131) E. coli isolates, including three ETEC strains. | [121] | ||
Swine | S. Enteritidis | Salmonellosis | Phage cocktail | 3.5 log CFU reduction of S. Enteritidis PT4 per gram of cecal content | [122] |
S. Enteritidis | Felix 01 | 100% efficacy in eliminating S. Enteritidis strains from tonsils 6 h after application of bacteriophage suspension | [123] | ||
S. Typhimurium | Phage with probiotics | Significant influence on the growth of weaned pigs in comparison with pigs not treated with phages | [124] | ||
S. Typhimurium | Single phage | Significant reduction (99%) or complete elimination (100%) of S. Typhimurium strains in ileum, tonsils, and cecum samples within 48 h after the first administration | [48] | ||
S. Typhimurium | Phage cocktail | Significant reduction in the concentration of 2 of 3 serovars (S. Enteritidis and Typhimurium) by 2–4 log CFU after administration of bacteriophage suspension at 1011 PFU | [125] | ||
S. Typhimurium | Phage cocktail | 100% reduction of the Salmonella ATCC 14028 reference strain and 92.5% of field isolates | [126] | ||
E. coli (ETEC) | Diarrhea | Phage cocktail | In comparison with the control group, the E. coli K88 CFUs in the duodenum, jejunum, ileum, cecum, colon, and mesenteric lymph nodes were lower in each phage-treated group, with differences at log levels of 0.83, 1.61, 1.67, 2.4, 1.47, and 1.65, respectively. | [58] | |
E. coli | A221 | With the treatment of phage A221, the body weight of piglets increased, and the percentage of Enterobacteriaceae in duodenum decreased to 0.64%. | [127] | ||
E. coli (MDR) | PT-10 | When comparing the E. coli CFU in the feces between the phage-treated group and the control group, a difference of 4 log levels was observed on the 10th day. | [103] | ||
E. coli (ETEC) | Single phage | When comparing the number of E. coli isolated from challenged pig feces to the control group, there was an average difference of 1 log level throughout the experimental period. | [128] | ||
Chicken | C. jejuni | Campylobacteriosis | Cj6 | The maximum (2 log10 levels) reduction was achieved in samples that were treated with high densities of C. jejuni and high MOI of the phage at both storage temperatures. | [66] |
C. jejuni | Three-phage cocktails | 2 log10 levels of reduction were achieved using the cocktail consisting of the three phages. | [129] | ||
C. jejuni | CP81 | No reduction was observed at 4°C in situ on meat or in vitro. 1 log10 reduction was observed in vitro at 37°C. | [67] | ||
C. jejuni | Phage cocktail | 3.2 log10 CFU/g lower C. jejuni counts than in the control until slaughter | [71] | ||
C. jejuni | Φ3, Φ15 | Modest reduction of 0.2 log10 level. 0.8 log10 at 4°C | [130] | ||
S. Gallinarum | Salmonellosis | CJø01 | Treatment using bacteriophages as a feed additive for chickens having contact with infected individuals led to a mortality rate of only 5%, as compared with 30% in the group that did not receive phage therapy. | [75] | |
S. Enteritidis | PSE | 100% efficacy in eliminating S. Enteritidis strains from the tonsils, 6 h after application of bacteriophage suspension | [131] | ||
S. Enteritidis | CJ07 | The highest doses of bacteriophage significantly inhibited the replication of pathogens in the digestive tract of the chickens. | [132] | ||
S. Typhimurium | Three-phage cocktails | 10-fold reduction in bacteria in the chicken ileum, cecum, liver, and spleen | [76] |
In the livestock industry, various studies have been conducted to address the prevalent issue of pathogenic bacteria and bacterial diseases. This summary compiles research efforts aimed at preventing bacterial diseases in livestock through the application of bacteriophages. Numerous studies have been conducted, and further advancements in research are anticipated. The “Phage application” section provides information on the names or numbers of phages used in the treatment.
S. aureus, Staphylococcus aureus; MDR, multi-drug resistance; MSSA, methicillin-sensitive Staphylococcus aureus; MRSA, methicillin-resistant Staphylococcus aureus; VISA, vancomycin Intermediate Staphylococcus aureus (VISA); E. coli, Escherichia coli; MPEC, mammary pathogenic E. coli; ETEC, enterotoxigenic Escherichia coli; EPEC, enteropathogenic E. coli; C. jejuni, Campylobacter jejuni.
The pet industry is continuously growing globally, and recently, the term “Petconomy” has emerged. According to the American Pet Products Association (APPA), the expenditure in the U.S. pet industry surpassed $95.7 billion in 2019 [23]. According to Euromonitor, the global pet food market size will reach $102.1 billion in 2020 [24]. With the increasing scale of the Petconomy, there has been a growing interest in the health and well-being of animals, leading to a rise in the use of antibiotics for treating animal diseases [25,26]. However, the use of antibiotics has raised health issues, particularly issues related to the reduction of probiotics in the gut microbiota, which is becoming a significant consideration for pet owners when addressing animal health [27]. As a response to preventing intestinal pathogen infections, various products using phages have been introduced, and research on phage-based treatments for pets has increased (Table 3) [28,29]. This study aims to introduce successful cases of treating bacterial diseases in animals using phages, emphasizing the potential utility of phages in treating pathogenic bacterial infections, considering the close connection between human life and animals, especially livestock and pets, where antibiotics are the most commonly used.
Animals | Target bacteria | Symptoms | Phage application | Therapeutic activity | References |
---|---|---|---|---|---|
Canine | P. aeruginosa | Otitis media | Pa12, Pa18 | This study explored two sewage-derived phages capable of lysing a variety of P. aeruginosa strains, even those resistant to fluoroquinolones. Notably, the phages showed effectiveness against isolates with high enrofloxacin and orbifloxacin resistance. | [78] |
P. aeruginosa | Phage cocktail | A bacteriophage treatment for chronic P. aeruginosa otitis in 10 dogs resulted in a significant improvement within 48 h, with a mean clinical score fall of 30.1% and a mean P. aeruginosa count fall of 67%. | [77] | ||
E. coli (UPEC) | Urinary tract infections | Single phage | Bacteriophages effectively lysed 94% of UPEC strains, with 10 of them individually lysing ⩾51% of the strains. | [79] | |
S. pseudintermedius (MRSP) | Skin infections | Single phage | All phages (n = 4) successfully lysed all MRSP isolates (n = 17); however, their lytic activity was restricted to S. pseudintermedius and S. schleiferi. | [80] | |
Staphylococcus spp. (MDR) | Conjunctivitis | Single phage | The phage eye drops tested in the experiment demonstrated 100% effectiveness in vitro against the Staphylococcus isolates under investigation. | [133] | |
L. infantum | Visceral leishmaniasis | CVL serodiagnosis | This study found eight mimotopes for accurate CVL serodiagnosis using a cost-effective and straightforward phage-ELISA assay. | [83] | |
Feline | E. coli (UPEC) | Urinary tract infections | Single phage | Bacteriophages effectively lysed 94% of UPEC strains, with 10 of them individually lysing ⩾51% of the strains. | [79] |
In the companion animal industry, various studies have been conducted to address the prevalent issue of pathogenic bacteria and bacterial diseases, using bacteriophages for prevention. The use of phages in the companion animal industry is relatively limited compared with livestock, but it is predicted that more research will be conducted in the future. The “Phage application” section provides information on the names or numbers of phages used in the treatment.
PHAGE THERAPY IN THE LIVESTOCK INDUSTRY
Bovine mastitis, characterized by inflammation of the mammary gland due to bacterial infection via the teat canal, stands out as one of the most crucial diseases [30]. The condition is classified into clinical and subclinical mastitis based on symptoms. Clinical mastitis presents visible abnormalities such as redness, udder swelling, and milk clot formation. In contrast, subclinical mastitis lacks visible abnormalities or milk clotting but can be confirmed by an increase in somatic cells [31]. Mastitis is further categorized as contagious or environmental depending on the pathogen involved. Contagious mastitis, caused by pathogens like Staphylococcus aureus, Streptococcus agalactiae, and Corynebacterium bovis, can transmit from one cattle to another. Environmental mastitis is instigated by pathogens such as Escherichia coli, Klebsiella spp., Streptococcus dysgalactiae, and Streptococcus uberis [8,32].
Among these pathogens, S. aureus, along with nonaureus staphylococci, is the most frequently detected contagious pathogen [33]. E. coli, a common Gram-negative bacterium, is also recognized as a frequent mastitis-causing pathogen [34]. Notably, while E. coli rapidly induces an immune response, S. aureus does not trigger a similar response. Consequently, S. aureus infections tend to be milder, leading to chronic mastitis lasting for months. In the previous study, the efficacy of a phage cocktail composed of two phages in inhibiting S. aureus N305, isolated from bovine mastitis, was verified through in vitro testing. Subsequently, an in vivo test was conducted, confirming the efficacy of the phage cocktail in a mouse mastitis model created by inoculating the S. aureus N305 strain into a lactating mouse [35]. Another research team isolated five S. aureus lytic phages from mastitis cow milk samples, belonging to the Podoviridae family. After in vitro characterization of the five phages, the efficacy of a phage cocktail was confirmed in a mouse mastitis model inoculated with S. aureus CVCC 546. Phage cocktail therapy alleviated the immune response, as evidenced by the reduction in proinflammatory cytokines tumor necrosis factor alpha (TNF-α) and interleukin 6 (IL-6), along with histopathological analysis [36]. Given that previous experiments utilized a mouse model, the actual application of bacteriophages to bovine mastitis remains uncertain. Prior study conducted an experiment with 24 lactating Holstein cows with preexisting asymptomatic S. aureus mastitis. Using a single bacteriophage (K - ATCC 19685-B1) for all experiments, intramammary infusions of either 1.25 × 1011 plaque forming unit (PFU) or saline were administered once per day for 5 days. Following bacteriophage infusion, mean milk production increased [37]. In prior study, the recombinant endolysin (Trx-SA1) from a novel bacteriophage (IME-SA1) was obtained for the experiment. The udder-infected group with S. aureus showed a decrease in both somatic cell count and pathogen level when treated with Trx-SA1, whereas the udder-infected group with E. coli did not exhibit the same response [38].
Neonatal calf diarrhea, defined as diarrhea occurring within the first month of life, is a common occurrence [39]. This condition can be caused by various factors, including viruses, bacteria, and stress. Antibiotics have historically been extensively used to treat bacterial-induced diarrhea in calves. However, the emergence of antibiotic-resistant bacteria and the presence of antibiotic residues have raised concerns [40]. In response to these issues, a study utilizing bacteriophages is currently underway.
Screening a cocktail of four unique bacteriophages based on 36 E. coli strains isolated from cattle with clinical mastitis, researchers observed that the bacteriophage cocktail significantly inhibited the growth of 58% of strains from Washington State and 54% of E. coli mastitis isolates from New York State. This suggests a relatively broad spectrum of action against related strains in two distinct regions [41]. In another study, three lytic phages—SYGD1, SYGE1, and SYGMH1—were isolated from the sewage of a dairy farm. These phages were administered in cocktail form to cattle with mastitis. Similar to antibiotic treatment, reductions in E. coli CFU and milk somatic cell count were noted, accompanied by a decrease in proinflammatory cytokines, IL-β and TNF-α [42]. In a related study, a combination of probiotics and bacteriophages was explored as an alternative to antibiotics for controlling E. coli, a common cause of neonatal calf diarrhea. The study comprised four groups: a healthy control group, a positive control group with diarrhea, a group treated with probiotics and bacteriophages in healthy calves, and a group treated with probiotics and bacteriophages in calves with diarrhea. Over an observation period of 11 days, the use of probiotic-phage suppositories led to a reduction in the duration of diarrhea in calves, with symptoms completely disappearing within 24–48 hours post-treatment. This therapeutic approach stimulated the activation of the calf’s immune system, enhancing both specific and nonspecific responses and increasing resistance to infection [43].
The domestic pig, Sus scrofa domesticus, holds significant economic and nutritional importance, being raised in various industrial systems and habitats. Susceptibility to emerging and re-emerging infections is common among swine [44]. The introduction of pigs into diverse environments by humans can lead to viral adaptability and genetic recombination within infectious agents. Specific circumstances, such as changes in land use, trade, and other human-induced occurrences, can promote the transmission and dissemination of infections, potentially resulting in the development of new viruses with zoonotic potential [45]. Swine health is a critical consideration for producers from farrowing through slaughter, impacting profitability through reduced weight gain or animal mortality in the event of herd infections [46]. Enteric viruses, especially highly contagious ones like the porcine epidemic diarrhea virus, pose significant economic challenges for farmers and the entire industry [47].
Examining phage dispersion tactics, researchers explored oral gavage and the addition of phages to drinking water and feed. Investigating the administration of phages through oral gavage, challenged pigs received phage cocktails every 2 hours for 6 hours. While this approach reduced Salmonella levels in cecal samples compared to a control, it is considered technically challenging for practical use on multiple infected pigs [48]. Instead, a delivery method enabling simultaneous treatment of several animals, such as in feed or water, becomes essential. One research was exemplified by the assessment of a three-phage cocktail in drinking water, revealing a noteworthy reduction in intestinal inflammation and Salmonella invasion. [49]. Although water-based phage dissemination is simple, it reduces product shelf life, and transporting large volumes of liquid phages is challenging [50]. Moreover, although high-titer phage stocks may be introduced to drinking water as a concentrate or powder, there is still the chance of human error [51]. Feed-based distribution offers various benefits, and recent advances in phage formulation studies demonstrated that phages can be transformed into a fine powder using excipients through lyophilizing, freeze-drying, or spray drying [52]. Excipients keep phages from drying out and act as agents that replace water to keep virion particles stable while they dry [53]. FDA-approved phage drying excipients, including trehalose, sucrose, mannitol, leucine, and the pH-sensitive polymer Eudragit S100, maintain phage stability during drying [54,55]. To date, no published study has documented improving phage drying at a commercial scale, but companies have filed patents based on drying phages, indicating a growing interest in developing dried phage products.
Administering a microencapsulated phage cocktail to young pigs challenged with Salmonella enterica serovar Typhimurium (S. Typhimurium) 2, 4, and 6 h later, researchers observed significantly lower ileal concentrations of S. Typhimurium in phage-treated pigs compared to untreated pigs. Moreover, the ileal S. Typhimurium concentrations were more frequently below the detectable limit in phage-treated pig samples than in untreated pig samples [56]. In two trials, researchers administered a microencapsulated phage cocktail to young pigs infected with S. Typhimurium. Concentrations of S. Typhimurium in ileal, cecal, and cecal tonsil samples were numerically lower (not statistically compared) in phage-treated pigs at necropsy (6 h post-challenge) than in untreated pigs. While some lymph node samples from both untreated and phage-treated pigs were positive for the challenge organism 6 h after exposure, all fecal samples were positive for the challenge organism. S. Typhimurium levels in phage-treated pigs were below the detection limit in several samples [48]. Using a phage cocktail suspended in sodium bicarbonate, researchers treated S. Typhimurium-challenged market-weight pigs. At necropsy, the quantities of S. Typhimurium in cecal and ileal samples were not significantly different (p > 0.05) between phage-treated and untreated pigs (18 ± 2 h post-treatment). Numerical variations in S. Typhimurium concentrations were most apparent between groups of pigs treated with 1010 PFU/pig and untreated pigs. Similarly, despite numerical changes in incidence, the incidence of S. Typhimurium in necropsy fecal samples was not significantly different (p > 0.05) in phage-treated versus untreated pigs [57].
In a study, 28-day-old pigs were infected with E. coli K88 and K99 using a phage cocktail. The phage treatment began on the day of the challenge and continued until the experiment concluded (7 days post-challenge). Fecal E. coli K99 concentrations did not differ significantly ( p > 0.05) between phage-treated and untreated pigs during the study (1, 3, and 7 days post-challenge). However, overall fecal E. coli K88 concentrations in phage-treated pigs were significantly lower ( p < 0.01) than those in untreated pigs. Adhesion of E. coli K88 to the ileum and cecum of phage-treated pigs was significantly lower ( p < 0.05) than that of untreated pigs. The adherence of E. coli K88 to the duodenum, jejunum, colon, and mesenteric lymph nodes did not differ significantly ( p > 0.05) between treatment groups. E. coli K99 adhesion did not differ significantly ( p > 0.05) between phage-treated and untreated pigs in any sample type [58].
Regarding another investigation, initial antibiotic resistance was found in 87.3% of E. coli strains isolated from healthy or diarrheal fecal samples from three pig farms. Using these 87 E. coli strains as indicator hosts, 45 coliphages were extracted, showing a higher abundance in the postweaning stage than in the preweaning stage (24 versus 17 in the Nanjing farm and 13 versus 4 in the Chuzhou farm). Furthermore, each farm included the most common coliphage strain. Pathogenic E. coli-specific bacteriophages were detected in the intestines of examined pigs (9/10 in Nanjing farm and 7/10 in Chuzhou farm), and the majority had significant bacteriostatic effects ( p < 0.05) on pathogenic E. coli strains. Polyvalent bacteriophages N24, N30, and C5 were found. With relatively few differences in infection characteristics, the N30 and C5 strains shared 89.67% genetic similarity. Pathogenic E. coli-specific bacteriophages and polyvalent bacteriophages are common in piglet stomachs, and weaning is an important event that determines coliphage abundance [59].
Campylobacter jejuni is a prevalent pathogenic bacterium globally and a leading cause of foodborne illness. Typically associated with poultry, C. jejuni naturally inhabits the digestive tracts of various bird species and is most commonly found in animal feces [60]. Fortunately, infections caused by C. jejuni can lead to severe gastroenteritis but are rarely life-threatening [61]. Nonetheless, in the United States alone, approximately 2 million cases of Campylobacter enteritis occur annually, constituting 5%–7% of gastroenteritis cases, with the majority attributed to poultry, including chickens, turkeys, and waterfowl [62]. In fact, the cornerstone of Campylobacter enteritis treatment lies in maintaining electrolyte balance rather than antibiotic therapy, as most patients with this infection experience self-limiting illnesses that do not necessitate antibiotics. However, antibiotics are warranted in specific clinical scenarios such as high fever, bloody stools, pregnancy, and human immunodeficiency virus infection [63]. To prevent such situations, minimizing bacterial infection during the early stages of poultry growth is crucial. Consequently, several studies exploring the inhibition of C. jejuni using bacteriophage, an antibiotic alternative, are underway. Still, there is a scarcity of patents related to phage products targeting this bacterium. In conclusion, as global chicken consumption continues to rise, ongoing research on Campylobacter phages is essential.
Until now, various in situ and in vivo studies related to C. jejuni-infecting phages have been conducted. Atterbury et al. preserved chicken skin sections inoculated with varying concentrations of C. jejuni and bacteriophages at 4°C and 20°C. At the maximum phage concentration (107), there was a reduction of bacteria by 1.1–1.3 log levels in the 4°C treatment setting and a 2.3–2.5-log reduction in the 20°C treatment setting compared with the control group [64]. Previous study observed a 1-log reduction in chicken skin treated with phages at a concentration of 106 PFU/cm2 compared with untreated control groups [65]. Another research team treated beef samples inoculated with C. jejuni with bacteriophages and stored them at 5°C and 24°C. High-density C. jejuni and phage-treated samples achieved a maximum reduction (2 log levels) at both storage temperatures [66]. The other study observed no reduction in situ or in vitro in meat at 4°C, whereas a 1-log reduction was observed in vitro within the test tube at 37°C [67].
In addition to the aforementioned in situ experiments, various in vivo experiments have been conducted. Previous study observed an initial 3-log reduction, followed by a resurgence in C. jejuni CFU counts within 5 days, which were 1 log lower than the control group [68]. Similarly, another research demonstrated reductions ranging from 0.5 to 5 log levels depending on the gut region and phage-host combinations, with the largest bacterial reduction achieved within 24–48 h [69]. Additionally, prior study achieved a 2-log reduction using a cocktail of three phages. Interestingly, phage delivery through food was more effective than oral gavage in nutrient broth [70]. The other research group administered a phage cocktail to birds through drinking water, resulting in C. jejuni counts 3.2 log CFU/g lower than the control group until slaughter [71].
Salmonella is one of the most problematic pathogenic bacteria in poultry, along with C. jejuni [72]. There are two well-known species of Salmonella, Salmonella enterica and Salmonella bongori, with S. enterica further divided into six subspecies containing over 2,600 serotypes. In particular, the nontyphoidal serotypes, unlike typhoidal serovars that can only be transmitted among humans, pose a zoonotic threat, being capable of common infections between animals and humans, thereby giving rise to potential public health concerns [44]. In the poultry industry, the most frequently occurring serotypes implicated in the majority of Salmonella infection incidents are S. Enteritidis, S. Typhimurium, and S. Gallinarum [73]. Research on Salmonella phages, similar to the studies conducted on C. jejuni, has been extensively conducted to suppress this bacterium without relying on antibiotics.
In a previous study conducted to reduce contamination by foodborne pathogens in poultry products and decrease the incidence, severity, and mortality of diseases, a concentration of 108 CFU of S. Enteritidis PT4 culture in 100 μL was orally administered to chickens. Subsequently, a phage cocktail consisting of CNPSA1, CNPSA3, and CNPSA4 at a concentration of 1011 PFU was administered orally as a single dose. Thus, a significant reduction of pathogenic bacterial populations in the digestive tract was observed with the single administration of a high-titer bacteriophage mixture. Furthermore, a reduction of 3.5 log levels in colony-forming units of S. Enteritidis PT4 per gram of cecal content was observed [74]. In prior research, an oral challenge with S. Gallinarum at a concentration of 5 × 108 CFU/mL was conducted. Following the challenge, bacteriophage CJø01 was used as a feed additive at a concentration of 106 PFU/kg. The administration of these bacteriophages to chickens that had contact with infected individuals resulted in a mortality rate of only 5%, compared with 30% in the group that did not receive phage therapy [75]. Finally, an oral challenge with a suspension of S. Typhimurium at a concentration of approximately 106 CFU/mL, administered in a volume of 0.5 mL, was conducted concurrently with oral administration of a bacteriophage cocktail (S2a, S9, S11) at a concentration of 106 PFU to 4–6 and 8–10-day-old chickens. The results revealed a 10-fold reduction in bacterial counts in the chicken’s ileum, cecum, liver, and spleen. Furthermore, when a commercial probiotic supplement was administered orally along with the bacteriophage cocktail, a synergistic antibacterial effect was observed [76].
PHAGE THERAPY IN COMPANION ANIMALS
The first in vivo experimental investigation using phage therapy to treat external otitis caused by Pseudomonas aeruginosa in dogs was reported recently. In the experiment, 10 dogs with a preexisting case of P. aeruginosa-related external otitis were used. The researchers isolated phages and then selected which virus would be most effective in killing the strains often seen in canine otitis to create a phage cocktail for administration to the animals. For the in vivo trial, the researchers opted for the phage combination that killed 90% or more of the P. aeruginosa strains tested [77,78].
The effectiveness of 40 bacteriophages against 53 uropathogenic E. coli (UPEC) strains was tested in vitro by a New Zealand research team. Phage mixtures have been shown to efficiently lyse over 90% of bacterial strains, suggesting that such mixtures may constitute a legitimate alternative to traditional medicines in the case of urinary infections [79].
Methicillin-resistant S. pseudintermedius (MRSP) strains are becoming increasingly common and are limiting the effectiveness of antibiotic molecules in treating infections in dogs. Thus, a team of researchers has recently set out to isolate and characterize specific bacteriophages for MRSP strains. Dog feces bacteriophages were tested on 66 different strains of S. pseudintermedius to determine their host range (17 strains resistant to methicillin, 43 sensitive to methicillin, and 6 isolated directly from dogs). When tested against a panel of resistant bacteria, all phages demonstrated lytic activity; however, they killed only 16%–28% of susceptible strains [80-82].
This study reported that L. infantum did not use a serological panel that included samples from dogs infected with Leishmania braziliensis because the researchers collected dog serum samples only in the Belo Horizonte metropolitan area, where a comparatively low incidence of Leishmania infection in dogs has been recently described. Therefore, the findings of this study should be used as proof-of-concept of the ability of the suggested synthetic antigens to help in the serodiagnosis of canine visceral leishmaniasis (CVL) and may serve as a reference for future tests. However, owing to their simplicity, ease of use, repeatability, and cheap cost, these new extremely accurate phage-fused peptides and their application in phage-enzyme-linked immunosorbent assays (ELISAs) may be swiftly used in the serodiagnosis used in CVL-monitoring programs [83].
UPEC, the primary infectious cause of urinary tract infections in dogs, cats, and humans, is a serious issue. This study aimed to determine whether a large variety of phages capable of lysing canine and feline UPEC exist in nature. Using 53 UPEC strains and 7 E. coli strains obtained from dog and cat feces, the ability of phages to cause lysis in vitro was studied. Between January 2002 and April 2004, four New Zealand animal health laboratories were contacted to obtain a total of 31 canine UPEC strains and 22 feline UPEC strains. Once lysis profiles suggested that a specific phage may be a potential candidate for in vivo testing, electron microscopy and DNA sequencing were used to perform more morphological and genetic studies on the phage in issue.
The majority of the canine and feline UPEC strains were lysed in vitro by naturally occurring phages that may be obtained by visiting a sewage treatment plant only once, according to the findings of the previous experiments. Of the UPEC strains that were examined, 17%–72% were eradicated by certain phages. More than half of all UPEC strains were lysed by the top 10 phages with the greatest host versatility. The phages were able to lyse 92% of the UPEC strains when combined, and each had a distinct lysis profile. In contrast to a previous study on E. coli urinary tract infections in children, however, 14 out of 44 phages lysed >15% of the UPEC strains; the present study demonstrates a far more positive result. Drulis-Kawa et al. discovered that only three of the phages they studied lysed >50% of the UPEC strains they analyzed [84]. This experiment used E. coli phages collected from a hospital collection. The phage propagation on UPEC may have contributed to the increased lysis success. The idea that UPEC isolated from cats and dogs is more susceptible to phage lysis than UPEC obtained from children cannot be eliminated at this time [79,85].
Most commonly found on the skin of animals, staphylococci are Gram-positive cocci that are asymptomatic, do not produce spores, and are facultative anaerobes. Two staphylococcal species, S. aureus and Staphylococcus schleiferi, are becoming more prevalent as they are harmful to rodents and birds. Staphylococcus intermedius has been linked to instances of superficial dermatitis, bacterial folliculitis, and superficial pyoderma in cats, although staphylococci do not appear to be a prominent cause of any particular illnesses in cats. An array of drugs used to treat staphylococcal infections in canines and felines has contributed to the development of drug-resistant forms of the bacteria. Penicillins, cephalosporins, macrolides, lincosamides, fusidic acid, tetracyclines, chloramphenicol, potentiated sulfonamides, aminoglycosides, and fluoroquinolones are all regularly used antibiotics in canine and feline treatment.
Nosocomial strains of staphylococci have exhibited rapid development of resistance to practically all the main types of antibiotics since their widespread usage became commercialized. Not much is understood about how staphylococci in cats and dogs become resistant to antibiotics. Conversely, plasmids are anticipated to play a crucial role in the dissemination of antibiotic resistance in these species. Either transposable elements or plasmids may serve as resistance gene vectors. Some studies have shown evidence of horizontal gene transfer between S. aureus and S. intermedius and between S. aureus and coagulase-negative staphylococci. Staphylococci are genetically diverse because of their interactions with a broad variety of other bacteria on the skin, mucosal surfaces, and respiratory tracts of mammals, all of which provide them with a rich source of genetic diversity. In a complex microbial community, resistance genes may be acquired rapidly. Staphylococci thrive on mammalian hosts, making them easily transmitted between animals and even to humans through cat and dog bites. There are extremely few accounts in the literature of staphylococci spreading from one species to another since staphylococci tend to be very species-specific. For example, cattle and cats are susceptible to infection from human S. aureus, presumably S. epidermidis strains and antibiotic-resistant staphylococci [86-89].
SUMMARY AND CONCLUSION
Since the discovery of antibiotics, phage therapy has not received considerable attention. However, the emergence of superbugs has emphasized the importance of bacteriophages in various industries. This study focuses on the use of phages in animal farming, specifically in the livestock and companion animal industries. In livestock farming, phages can be used throughout the entire production chain, with a particular emphasis on bacterial control in farms vulnerable to pathogenic bacteria. As animals in farming industries cannot manage hygiene on their own, external interventions are crucial to remove pathogenic factors [90]. Historically, antibiotics were efficient substances not only in eliminating bacteria but also in promoting animal growth [91,92]. Nevertheless, the rise of multidrug-resistant bacteria has prompted research into the potential of bacteriophages as an alternative to antibiotics [93-95]. Recent experiments have demonstrated the synergistic effect of bacteriophages and probiotics in controlling pathogenic bacteria and promoting animal growth [96-98]. Although the societal perception of phage use in humans remains negative, its application in animal farming is relatively acceptable, with some phage uses being legally permitted. In conclusion, research on phage therapy in animal farming is expected to become more active in the future.
Phage use in the livestock industry is strategically focused on vulnerable animals during their early stages. For instance, research indicates that a low concentration of Salmonella infection can be lethal to piglets but has a minimal impact on mature pigs [99]. Accordingly, in livestock, phage treatments primarily aim at preventing pathogenic bacterial infections and growth performance [100,101]. In contrast, with companion animals that have assured individual hygiene, the focus rests on bacterial diseases arising from their unique characteristics and lifestyle habits. This study observes a similar trend, where livestock research primarily centers around intestinal diseases directly linked to growth, such as Salmonella and E. coli [40,102-104]. In companion animals, studies extend beyond intestinal diseases to encompass conditions such as otitis and skin disorders [77,78]. Chronic illnesses, while quickly treatable with antibiotic products, pose the risk of developing antibiotic resistance with continuous use. Additionally, antibiotic treatments can disrupt the balance of symbiotic microorganisms by reducing beneficial bacteria [27,105]. Conversely, phage therapy alleviates concerns about antibiotic resistance, and according to the literature, phage resistance often lowers the pathogenicity of bacteria, significantly reducing the associated risks [106-108]. Consequently, while research in phage therapy for companion animals may be in its early stages, the growing societal recognition of pets as integral family members suggests that phage therapy holds potential as a research topic for treating various diseases in companion animals [109,110].
The extensive use of phage treatment, notwithstanding its benefits, raises new issues that extend beyond the scope of traditional medical practice and need new approaches. These include the need to increase phage strains in reference phage banks, the development of efficient phage screening techniques for the rapid identification of therapeutic phages, the design of effective treatment strategies that target infectious biofilms, the establishment of manufacturing procedures that ensure the quality and safety of preparations, and the assurance of the stability of preparations during storage and transportation. As infectious illnesses know no boundaries, a global action plan is required to make phage treatment accessible globally. This clearly necessitates active cooperation across nations to overcome logistical and regulatory obstacles, as well as between physicians and scientists to close the present knowledge gap and progress the area.