INTRODUCTION
L-Tryptophan (TRP) is an essential amino acid (AA) which requires to be supplemented in diets to meet the maximum production quality [1–3]. It is while most AAs are commonly assumed not to be deficient in cattle. Ruminal fermentation produces the microbial protein, which is supplied to the small intestines and delivers a good quality pattern of both essential and non-essential AAs, about 34%–89% of total protein [4,5]. The amounts of essential AAs supplied by microbes are sufficient to support maintenance and normal physiological status, but not maximal growth, milk, and beef production [6–8]. Although the assumption is that cows produce sufficient AA through their diet; however, numerous common feed ingredients (average CP = 23% of dry matter [DM], minimum CP = 3%, maximum CP = 95.5% of DM basis) contain an average concentration of TRP at approximately 1% or less of total CP [5]. Therefore, the proteins supplied from the diet do not contain sufficient essential AAs to meet the animal’s needs. In addition, normal protein supply could not be used efficiently for muscle synthesis [9]. This experiment aimed to investigate the effect of additional TRP as an essential amino acid (block) for bundling protein of muscles as its amount is lacking when maximum production is targeted. Thus, we hypothesized that TRP supplementation may improve muscle development, and thus worth investigation in the present study.
Previous literature investigated the relationship between reducing stress and TRP addition using injection or infusion to horses [10], dairy cows [11], and beef cattle [2]. However, there is a paucity of information regarding its supplementation effect on longissimus dorsi muscle and the related gene expression in Korean beef cattle. Thus, we hypothesized that TRP might not only be a building block for muscle development in steers but also induce gene-expression related muscle development and adipose tissue catabolism and fatty acid transportation in longissimus dorsi muscle tissue.
MATERIALS AND METHODS
All experimental procedures were in accordance with the “Guidelines for Care and Use of Experimental Animals” of Konkuk University (Approval no: KU18178). Eight Hanwoo steers (initial body weigt [BW] = 424.6 kg [SD 42.3]; 477 days old [SD 4.8]; Castration age 189 days old [SD 4.8]) were randomly allocated to two groups of control and treatment (n = 4) and supplied with diets with the total mixed ration (TMR; Table 1). The 477 days old steers have muscle and intramuscular fat in longissimus dorsi muscle. Thus, we selected the period for analyzing adipose tissue and muscle metabolism in longissimus dorsi muscle. The treatment group was fed 15 g of rumen-protected TRP (0.1% of TMR as-fed basis equal to 43.4 mg of TRP kg−1 BW; CJ CheilJedang, Suwon, Korea) provided as top dressing once a day at 0800 h. The rumen-protected TRP was conjugated of acetate and TRP. The amount added was triple times higher than the concentration provided in the control diet (Table 1). The rate of by-pass was indicated above 95% [3]. TRP was included at 0.829 g per 1 g of rumen-protected TRP. The treatment group was supplied by oral administration for each steer for 10 weeks.
The animals were placed in 10 m2 (2 m [length] × 5 m [breadth]) with two steers per pen at Konkuk University farm (Chungju, Korea). All animals in the control and treatment groups were fed 15 kg of TMR feedstuff (as-fed basis) once a day at 0800 h. Water was available ad libitum. Residues of TMR were recorded for each pen every day prior to providing the fresh TMR and water. Then, we used the equation from pen data to individual data. Dry matter intake (DMI) equation was calculated as follows: DMI/BW % = 1.2425 + 1.9218 × net energy for maintenance (NEm) − 0.7259 × (NEm)2 [12].
Blood samples from each steer were obtained at 1400 h via jugular venipuncture into the non-heparinized vacutainers (20 mL; Becton-Dickinson, Belliver Industrial Estate, PL6 7BP, Plymouth, UK) to obtain serum for measuring biochemical parameters and the EDTA-treated vacutainers (Becton-Dickinson, Franklin Lakes, NJ, USA) to obtain whole blood for measuring hematological parameters at 0, 5, and 10 weeks. The collected blood samples were stored on ice before transferring to the laboratory. Then, blood in serum tubes was centrifuged at 2,740×g for 15 min at 4°C. The serum was transferred to a 1.5 mL tube and stored in a freezer (−80°C) for further analysis.
Protein metabolism was evaluated by measuring serum levels of total protein (TP), albumin (ALB), globulin (GLO), creatinine phosphokinase (CPK), and blood urea nitrogen (BUN). Energy metabolism was evaluated by measuring the serum level of glucose (GLU), triglyceride (TG), and nonesterified fatty acids (NEFA). These biomarkers were assayed by using a biochemical autoanalyzer (FUJI DRI-CHEM 7000; Fujifilm, Tokyo, Japan). Whole blood samples were used for analyzing complete blood-cell count, including granulocyte (GRA), lymphocyte (LYM), monocyte (MON), platelet (PLT), red blood cell (RBC), hematocrit (HCT), hemoglobin (HGB), and white blood cell (WBC) using VetScan HM2 (Diamond Diagnostics, Abaxix, Holliston, MA, USA).
Longissimus dorsi muscle samples (12 to 13 ribs, approximately 2 g) were collected from four steers in each group via surgery performed by a professional veterinarian at the end of the study (10 weeks). Briefly, local anesthetic agents were administered at 6 equidistant points around the resection area, namely, the longissimus dorsi muscle at position 12 to 13 of the ribs. The veterinarian resected 5 cm of skin in the direction of the muscle and then dissected the longissimus dorsi muscle tissue using surgical equipment. The collected longissimus dorsi muscle tissues were washed immediately into DEPC-treated distilled water (diethyl pyrocarbonate, Sigma-Aldrich, St. Louis, MO, USA) for protecting them from RNase contamination. The tissues were then preserved in 50-mL tubes after dipping into liquid nitrogen and placed in dry ice until transferring to the laboratory. The tissue samples were ground into powder in liquid nitrogen. The TRIzol was used to extract total RNA to follow up the method of [13]. The concentration of RNA was detected by spectrophotometric analysis (NanoDrop 1000, Thermo Scientific, Massachusetts, MA, USA). The RNA integrity was assessed using an RNA nano 6000 assay kit for an Agilent Bioanalyzer 2100 system (Agilent Technologies, Santa Clara, CA , USA). When the RNA integrity number (RIN) was 6 or higher, it was used for complementary DNA (cDNA) synthesis. The RNA used for this real-time polymerase chain reaction (PCR) analysis was RIN = 6.5 (SD = 0.27).
To synthesize cDNAs, 1 μg of RNA was reverse transcribed in a 100 μL reaction volume with an iScriptTM cDNA synthesis kit (Bio-Rad, Hercules, CA, USA) according to the manufacturer’s protocol. Quantitative real-time PCR (qRT-PCR) was performed on duplicate samples by using a CFX Connect TM Real-Time System (Bio-Rad) with IQTM SYBR Green Supermix reagents (Bio-Rad). The following PCR conditions were used: 95°C for 3 min and 40 cycles at 95°C for 10 s, 51°C to 61°C for 30 s and 72°C for 30 s. Expression of mRNA of gene involved in muscle development (myogenic factor 6 [MYF6], myoblast determination [MyoD], and myogenie [MyoG]), fat accumulation (CCAAT/enhancer-binding protein alpha [C/EBPα] and peroxisome proliferator-activated receptor gamma [PPARγ]), adipose tissue catabolism (lipoprotein lipase [LPL], stearoyl-CoA desaturase [SCD]), and fatty acid transportation (fatty acid binding protein 4 [FABP4]). 18S ribosomal RNA (18S), glyceraldehyde3-phosphate dehydrogenase (GAPDH), and ribosomal protein lateral stalk subunit P0 (RPLP0) primers were designed using the National Center for Biotechnology Information website. 18S,GAPDH, and RPLP0 were used as housekeeping genes [3,14] (Table 2).
The GLM procedure of SAS (SAS 9.4 Institute, Cary, NC, USA) was employed for two-way analysis of variance to analyze our data. Growth performance data (BW, average daily gain [ADG], and gain to feed ratio [G:F]), and mRNAs expression were assessed using Student’s t-tests. Data of blood biochemical and hematological parameters were used for mixed procedure of SAS using repeated measures. The model included the fixed effect of diet (control and 15 g rumen protected TRP group), and the random effects of blood sampling time. Blood was collected three times (0, 5, and 10 weeks). Data were presented as least-square means. Significance was set at p ≤ 0.05, and the tendency was declared at 0.05 < p ≤ 0.1.
RESULTS AND DISCUSSION
The amount of TRP in the treatment group was about 3 times higher than the amount in the control group. However, no differences were observed in growth performance parameters including BW, ADG, and G:F (p > 0.05; Table 3). Ma et al. [15] supplied 3 doses of rumen-protected TRP (48.1, 96.2, and 144.2 mg kg−1 BW) to cashmere goats, and found increased BW and ADG by supplementation of 96.2 and 144.2 mg to the TRP group, but no difference by supplying 48.1 mg TRP. Henry et al. [16] examined dietary supplementation of 47.9 and 83.9 mg of TRP kg−1 BW in comparison with the control on pigs and found higher average daily feed intake (FI), ADG, G:F, muscle gain (g/d), and fat gain (g/d) in the 83.9 mg TRP kg−1 BW group than in the other groups. Newborn calves that were supplied with colostrum and milk plus 1.5 mg of 5-hydroxy-TRP kg−1 BW during 15 days after birth showed on increased immune factors, but no changes in growth performance and blood biochemical parameters including glucose, fatty acids, calcium, and magnesium [17]. Based on the results of the previous studies, we considered that the given 43.4 mg of TRP kg−1 BW might not be sufficient to improve the growth parameters in the current study. Another possible explanation could be the low sample size in this study. Further study with feeding higher amounts of TRP and larger sample size may warrant the obtained results or cause different result and thus advisable.
Hematological parameters GRA, LYM, MON, PLT, RBC, HCT, and WBC were examined and only HGB showed the higher value in the treatment group (p = 0.025; Table 4). Hemoglobin was known as an iron-containing biomolecule that transports oxygen and carbon dioxide in red blood cells [18]. Serum TRP is positively correlated with iron metabolites (0.100 ≤ r2 ≤ 0.305) including HGB (r2 = 0.301) in human. TRP is an important factor for erythropoiesis which produces red blood cells, which is the development of erythropoietic stem cells to mature red blood cells [19]. Similar with these complete blood count (CBC) results, Lee et al. [3] found no changes in WBC, LYM, GRA, RBC, HGB, HCT, mean corpuscular hemoglobin (MCH), and PLT of steers fed with 0.1% rumen-protected TRP.
GRA, granulocyte; LYM, lymphocyte; MON, monocyte; PLT, platelet; RBC, red blood cell; HCT, hematocrit; HGB, hemoglobin; WBC, white blood cell; TP, serum total protein; ALU, albumin; GLO, globulin; CPK, creatinine phosphokinase; BUN, blood urea nitrogen; GLU, glucose; NEFA, nonesterified fatty acids; TG, triglyceride.
All blood biochemical parameters including ALB, GLO, GLU, CPK, NEFA, BUN, TG, and TP were not changed by supplementation of TRP (p > 0.05; Table 4). ALB, CPK, GLO, BUN, and TP were indicators related to nitrogen utilization in blood [20]. Supplied 0.8 g TRP improved nitrogen utilization by lambs fed 2.7% non-protein nitrogen of DM [21]. Thus, we investigated effects of TRP on nitrogen utilization by using blood metabolism markers in cattle, but there was no difference between the two groups. TRP is also considered as both glucogenic and ketogenic amino acid, and thus, it is expected to see the reflected changes in some blood biochemical parameters. Additionally, since most of the factors affecting blood metabolites by supplementation of rumen-protected AAs are related to energy metabolism, protein metabolism, and DMI [22], therefore, changes in those factors could be expected due to feeding TRP in this study. However, we could not find any difference in nitrogen utilization and its index in the blood (BUN) nor in other biochemical parameters between two groups. One reason could be attributed to the similar DMI in both groups. Another possible explanation could be due to the low sample size (n = 4) and less blood sampling in this study. It should be noted that we collected blood samples prior to feeding, and thus due its half-life (1.8 h in in-vitro culture [23]) and also body homeostasis mechanism, rumen protected TRP may not alter blood metabolite parameters including TP, ALB, GLO, BUN, CPK, GLU, NEFA, and TG. Consistent with our results, in the previous study under cold stress conditions, Lee et al. [3] reported unchanged blood biochemical parameters by supplementation of 0.1% rumen-protected L-TRP in Hanwoo steers, when blood samples were collected prior to feeding. Further study with a larger sample size and with the addition of a higher amount of TRP supplementation may result in significant differences in blood biochemical values particularly when blood was collected after feeding.
Relative mRNAs expression including MYF6, MyoG, FABP4, and LPL were higher in the treatment group than in the control (p < 0.05; Fig. 1). The MYF6 and MyoG are representative of muscle differentiation [24]. The MYF6 regulates the final differentiation of myotubes and is regarded as the principal factor influencing skeletal muscle phenotype. The MYF6 is expressed at a higher level in adult skeletal muscle than all of the other myogenic regulatory factors family genes including Myf5 and MyoD [25]. The MyoG is a critical transcription factor in myogenesis and is essential for the terminal differentiation of committed myoblasts [26]. FABP4 involves intracellular transport and fatty-acid metabolism [27]. The LPL has dual functions as a triglyceride hydrolase and a lipoprotein uptake [28]. Expression of LPL is recognized as a potentially important effector in lipoprotein metabolism, energy homeostasis, and body weight regulation [29]. Given the above review, dietary supplementation of TRP may be improved muscle differentiation, intracellular transportation of fatty acids, and catabolism of triglycerides in muscle by increasing the expression of MYF6, MyoG, LPL, and FABP4. The NEFA and TG showed no difference in serum between the control and treatment group (p > 0.05). However, the expression of LPL and FABP4 gene were increased. The reason could be assumed as the muscle tissues utilize free fatty acids to myocyte differentiation and thus resulted in higher expression of the aforementioned gene.
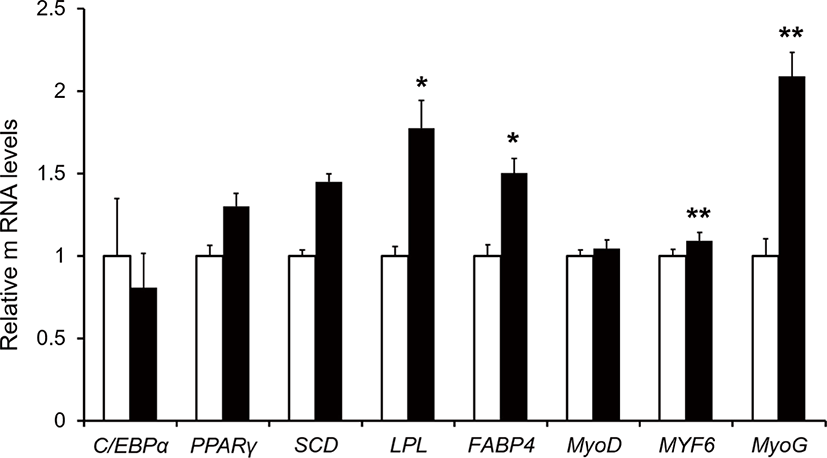
TRP is reported to be the limiting AA in growing lambs and cattle during non-protein N use. Thus, supplementation of TRP in a rumen-protected form had positive effects on growth [6,8]. We verified that 74 feed ingredients contained TRP (average CP = 23% of DM, minimum CP = 3%, maximum CP = 95.5% of DM basis), and that 52 feed ingredients contain the lowest TRP in the midst of total AA composition include average TRP approximately 0.98% of total CP (minimum TRP = 0.44%, maximum TRP = 1.55 % of total CP) [5]. Dietary supplementation of 43.4 mg TRP per 1 kg of BW improves to increase the expression of gene related to muscle development, but its potent influence on muscle mass production remained unclear. It is mainly because we did not observe any changes in BW gain (p > 0.05, Table 3) and also, the carcass traits were not examined in this study. This study showed the possibility of muscle growth through gene expression including MYF6, MyoG, FABP4, and LPL. Although, there was no difference in growth performance by supplied 43.4 mg TRP per 1 kg of BW (p > 0.05), an increase in genes related to muscle development can indirectly improve muscle growth. In the future experiment, with larger sample size, long-term effects of TRP supplementation, and experiments with higher levels of TRP may influence growth performance or improve carcass traits to which are related to increasing muscle mass.
CONCLUSION
Dietary supplementation of TRP (43.4 mg kg−1 BW and 65 days in this study) may promote muscle development as observed in this study by increasing the expression of MYF6, MyoG, FABP4, and LPL gene. Further study with a larger sample size and higher amount of TRP supplementation is required to investigate possible changes in growth performance including carcass traits and blood metabolites and also to warrant the obtained results.