INTRODUCTION
The world is currently experiencing record-breaking heat waves, with temperatures reaching unprecedented levels. These changes pose a danger to swine farming and demand immediate action. High ambient temperatures hamper productivity in the animal industry, negatively affect animal welfare, and reduce production efficiency [1,2]. In swine production, sows are greatly susceptible to the impact of high ambient temperatures, particularly during lactation [3]. The swine industry is challenged by environmental hyperthermia, resulting in decreased and variable growth rates, altered carcass quality, low milk yield, poor sow performance, increased mortality and morbidity, and reduced efficiency [4–6]. Management of sows during lactation calls for the consumption of the recommended voluntary feed and water intake to maintain health, prevent excessive loss of body weight (BW) at farrowing, achieve a timely weaning-to-estrus interval (WEI) [7], and improve milk production [8,9]. However, heat stress (HS) triggers alterations in metabolism, behavior, and physiology that involve amplified respiration and diminished average daily feed intake (ADFI), resulting in a decline in milk production which affects piglet growth [10–12] and seasonal infertility [13,14]. Lactating sows are prone to HS due to increased metabolic demands and heat production by the mammary glands. Similarly, pigs naturally have underdeveloped physiological control of body temperature, with inadequate sweating through the skin owing to keratinized sweat glands and a thick layer of subcutaneous fat [15] and, occasionally, compromised intestinal integrity [16]. Sows under HS are prone to compromised intestinal epithelial barrier, allowing pathogens to infiltrate the bloodstream leading to both local and systemic inflammatory responses [17]. The immune system is activated to combat infiltrating pathogens, diverting energy and nutrients away from vital functions and compromising the overall health and productivity of the animals [18].
β-Glucan is a functional polysaccharide found abundantly in the cell wall of yeast, it is rich in β-1,3-D-glucose side chains located at β-1, 6 branching sites, this structural uniqueness makes the polysaccharide easily recognizable and accepted by the immune system easily than β-glucan from other sources [19]. In monogastric animals, dietary yeast β-glucan has immunostimulatory and hypocholesterolemic effects that facilitate the alleviation of stress [20], promotion of immune function [21], and antioxidant activities [22] because of free radical degradation [23]. It also has a high molecular weight and a high viscosity in water, which can affect the digestion and absorption of nutrients in the gastrointestinal tract [24] development of enzyme production, that enhance the digestibility of fat and starch [25,26]. It modulates the intestinal microbiota by increasing beneficial bacteria thereby reducing pathogenic bacteria like Escherichia coli [27]. Due to its natural antioxidant properties, yeast β-glucan can inhibit methotrexate-induced leukocyte apoptosis [28], reduce plasma lipid peroxidation induced by haloperidol [29], and alleviate oxidative stress in macrophages through the Dectin-1/Nrf2/HO-1 signaling pathway [30]. The aims of this study were to explore the effects of dietary yeast β-glucan on lactating sows under HS and determine how its supplementation may relate to lactating sow performance, litter performance, oxidative stress, hair cortisol levels, and inflammation status.
MATERIALS AND METHODS
The experimental procedures’ protocol was performed and sanctioned in accordance with the guidelines of the Institutional Animal Care and Use Committee of Kangwon National University (KW-211022-2), Chuncheon, Korea.
The experiment was done during the summer, with an average environmental temperature of 37°C. Thirty multiparous crossbred (Landrace × Yorkshire) sows at day 100 of gestation with an initial BW of 216.2 ± 7 kg of average parity of 3 were randomly allotted to three blocks (10 sows/treatment) in a completely randomized experimental design to determine the effect of β-glucan from Saccharomyces cerevisiae (effective content 95%, Biorigin, Brazil). The treatments are composed of; control (CON), BG5 (CON + 0.05% β-glucan), and BG10 (CON + 0.10% β-glucan). Ten replicated pens with two sows from each parity (1, 2, 3, 4, and 5) were included in each treatment. During the gestation period, each pig was accommodated in a separate stall measuring 2.05×1.08 meters with a concrete slatted floor. The gestation barn’s ambient temperature was recorded to be around 32°C, comprising an average of 33.5°C during the day and 29.1°C at night. On day 100 of gestation, sows were moved to the farrowing room and individually placed in farrowing crates with slatted floors until their litter was weaned. Each farrowing crate was equipped with a single-space feeder and a nipple drinker, ensuring unlimited access to feed and water for the sows. The temperature was automatically controlled by a ventilation fan and an automatic sensor. Piglet crates were warmed with heating pads on either side of the crates. The experimental basal diets used were formulated based on the guidelines provided by the National Research Council [31] report and fed from day 100 of gestation until day 21 of lactation. The experimental basal diets were carefully designed to fulfill or exceed the nutritional requirements of both gestating and lactating sows. Table 1 shows the formula and chemical composition characteristics of these diets on an as-fed basis. The ADFI was calculated by subtracting the daily unconsumed feed from the daily feed allocation.
Supplied per kilogram of vitamin premix: 12,000,000 IU vitamin A, 2,400,000 IU vitamin D3, 132,000 IU vitamin E, 1,500 mg vitamin K3, 3,000 mg vitamin B1, 11,250 mg vitamin B2, 3,000 mg vitamin B6, 45 mg vitamin B12, 36,000 mg pantothenic acid, 30,000 mg niacin, 600 mg biotin, 4,000 mg folic acid.
Devices for monitoring temperature and humidity (Campbell Scientific, Shepshed, U.) elevated 1.2 meters above ground were used to measure temperatures and relative humidity and recorded every 10 minutes. With a precision of approximately ± 0.5°C and a resolution of 0.02%. Humidity and temperature were calculated as per the description by Dikmen et al. [32] using the temperature and humidity index (THI). The THI was calculated using a specific equation: THI = [(1.8 × T + 32) − (0.55 − 0.0055 × RH) × (1.8 × T − 26)], where RH represents the relative humidity expressed as a percentage (%) and T is the temperature in degrees. The temperature and THI during the 21-day experimental period are shown in Fig. 1.
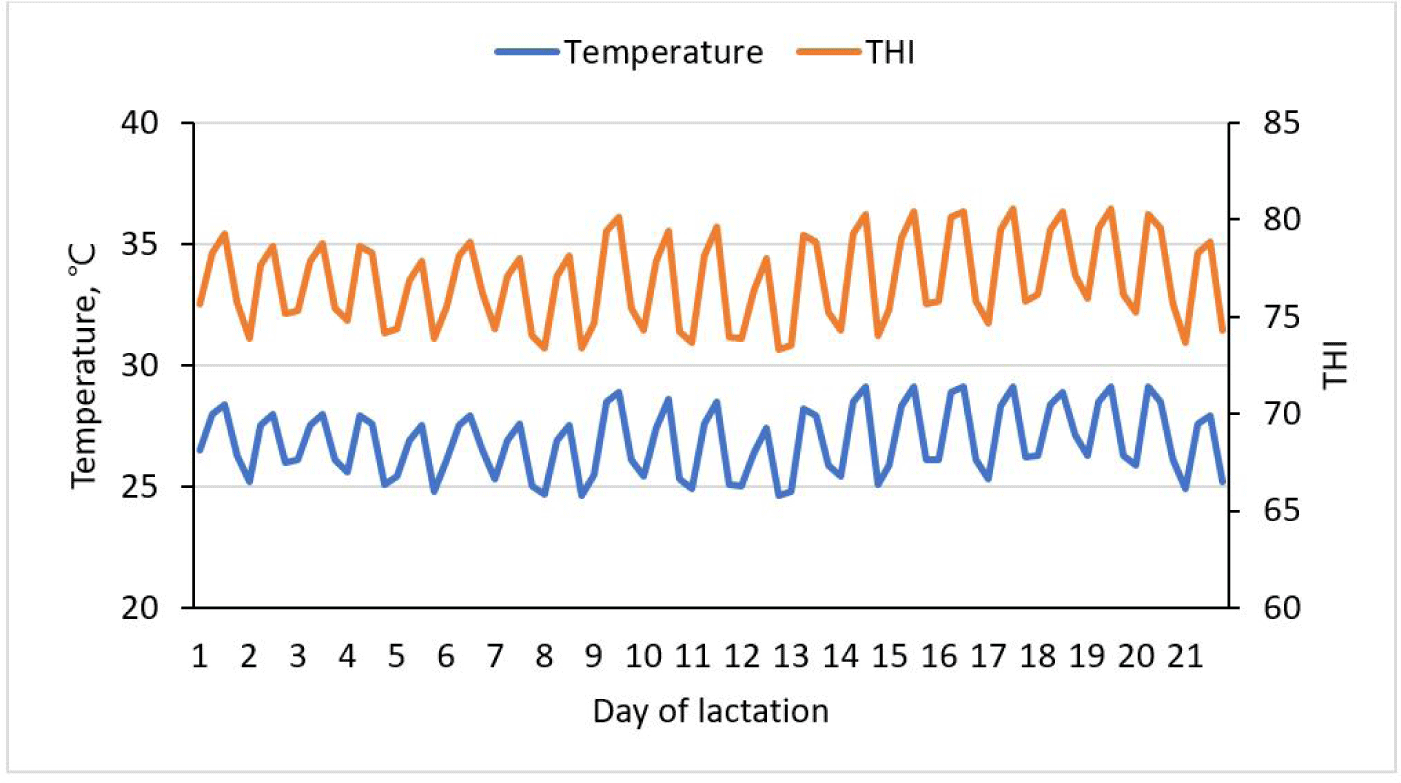
The respiratory rate (RR) was recorded in breaths per minute, they were determined by observing the rate of flank movement during a 60-second interval daily at 13:00 hrs, as described by Ghassemi Nejad and Sung [33]. Rectal temperature was measured using a digitally calibrated electronic thermometer (SK-1260, SATO, Tokyo, Japan) with accuracy 0.1°C, inserted 2 cm into the rectum every morning (07:30) and evening (18:00).
On days 112 (farrowing) and the 21st day of weaning, BW was measured as described by Kim et al. [34]. The back fat (BF) thickness was measured using an ultrasonic device (Anyscan BF, SONG KANG GLC, Seongnam, Korea) at the 10th rib on day 112 and at weaning. The litter total born, born alive, stillborn, BW at birth, and weaning, as well as the number of piglets weaned, were recorded. The ADFI of the sows was computed by taking the daily difference between the total feed allocated and the total feed left unconsumed for each sow. Additionally, WEI was calculated by recording the number of days between the weaning of piglets and the first observed signs of estrus in the sow.
Hair cortisol analysis was done by shaving the foreheads of lactating sows at farrowing and weaning [35]. The collected hair samples were washed with isopropanol (5 mL) afterward, the samples were subjected to drying in a vacuum dryer at 35°C and subsequently stored in aluminum foil within polypropylene tubes for the drying process (HM Hyundai Micro, Seoul, Korea). To determine the concentration of the extracted sample, cortisol extraction was performed by methanol dilution, and the samples were examined using an ELISA kit (ADI-900-071, Enzo Life Sciences, Farmingdale, NY, USA) following the provided instructions.
From each group, three sows were selected randomly, and blood samples were obtained from each sow through the jugular venipuncture using 10 mL disposable syringes (plasma [K2EDTA tube], serum [plastic tube], Becton Dickinson Vacutainers, Franklin Lakes, NJ, USA) 24 hours postpartum and at weaning. Serum and plasma samples were harvested by centrifugation at 3,000×g for 15 min at 4°C, afterward, the samples were divided into 2 ml microcentrifuge tubes and then kept at a temperature of −20°C for further analysis. Tumor necrosis factor-α (TNF-α), lipopolysaccharide (LPS), and lipopolysaccharide-binding factor (LBP) were measured using the commercially available kit: TNF-α (Fluorescent, Luminex, Millipore, Austin, TX, USA), LBP, and LPS (Fluorescent, Luminex, Millipore).
Antioxidant capacity was evaluated by the determination of superoxide dismutase (SOD), malondialdehyde (MDA), total antioxidant capacity (TAC), hydrogen peroxide (H2O2), catalase, and reactive oxygen species (ROS). 5 mL of blood was collected through an anterior vena cava puncture on the 21st day after overnight fasting using 3 healthy sows per treatment (n = 9) using disposable syringes with needles, the blood samples were promptly moved to a Vacutainer (Becton Dickinson Vacutainer) with a non-anticoagulant. All samples were kept at room temperature for 4 hours and subsequently centrifuged for 10 minutes at 3,000×g to separate the serum. The SOD, total TAC, H2O2, catalase, and the quantity of MDA were assessed using commercial kits (Nanjing Jiancheng Bioengineering Institute, Nanjing, China). The level of ROS the measurement of (the specific parameter) was also performed using commercial kits (Beijing Huaying Bioengineering, Beijing, China).
Data were analyzed using the general linear model procedure of SAS (SAS Institute, Cary, NC, USA), with sow as the experimental unit. The means were separated using the Tukey test. Data are presented as means ± SEM. Figures were created using GraphPad Prism (GraphPad Software, San Diego, CA, USA). The main effects of separating treatments are CON, BG5, and BG10. Probability was considered significant at p < 0.05.
RESULTS
During lactation, from day 17 to day 21, breaths per minute were significantly lower (p < 0.05) in the BG5 and BG10 than in the CON treatment. However, no significant differences in rectal temperature were noted between the three groups, indicating that different concentrations of yeast β-glucan did not have a discernible effect on rectal temperature (Figs. 2 and 3).
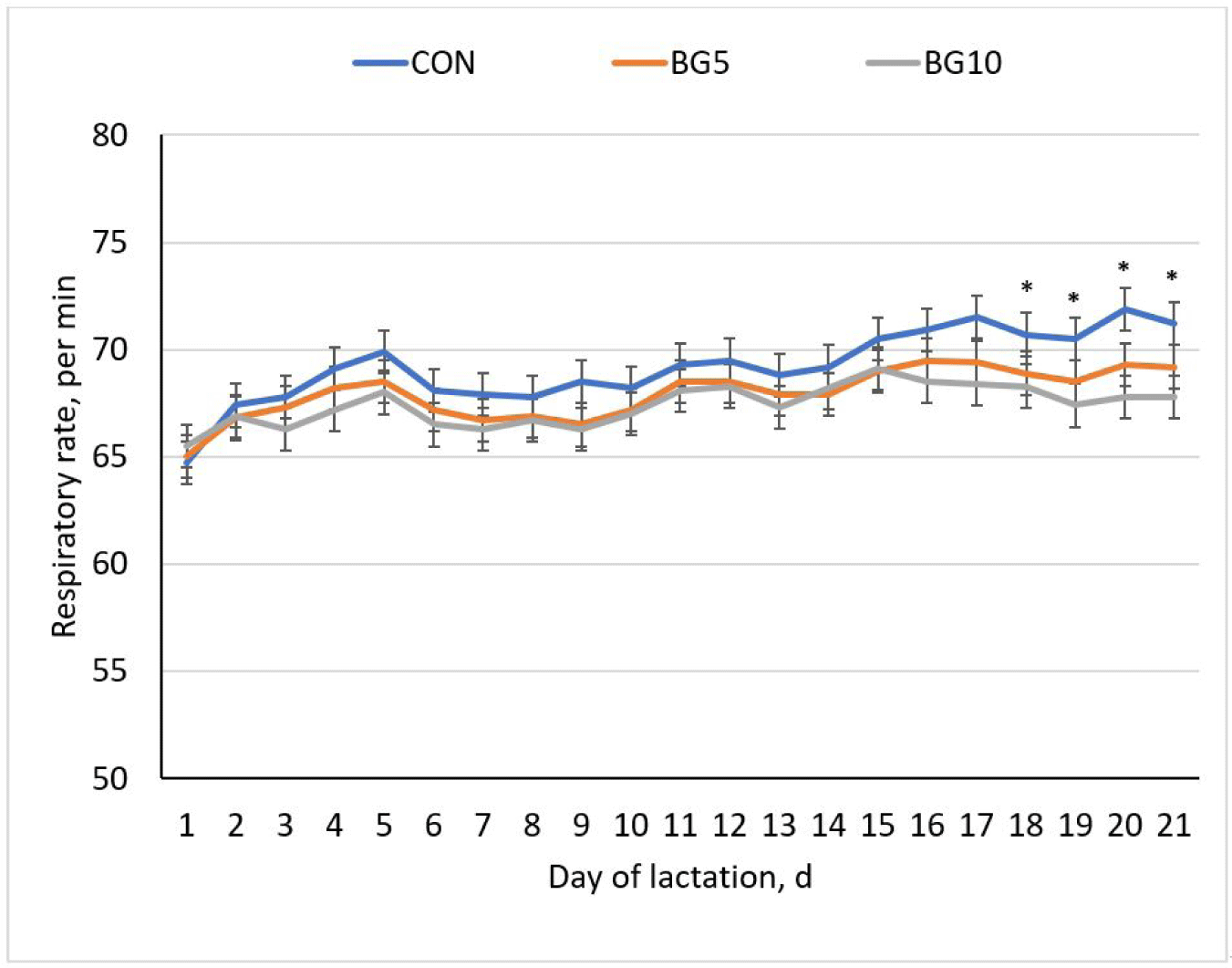
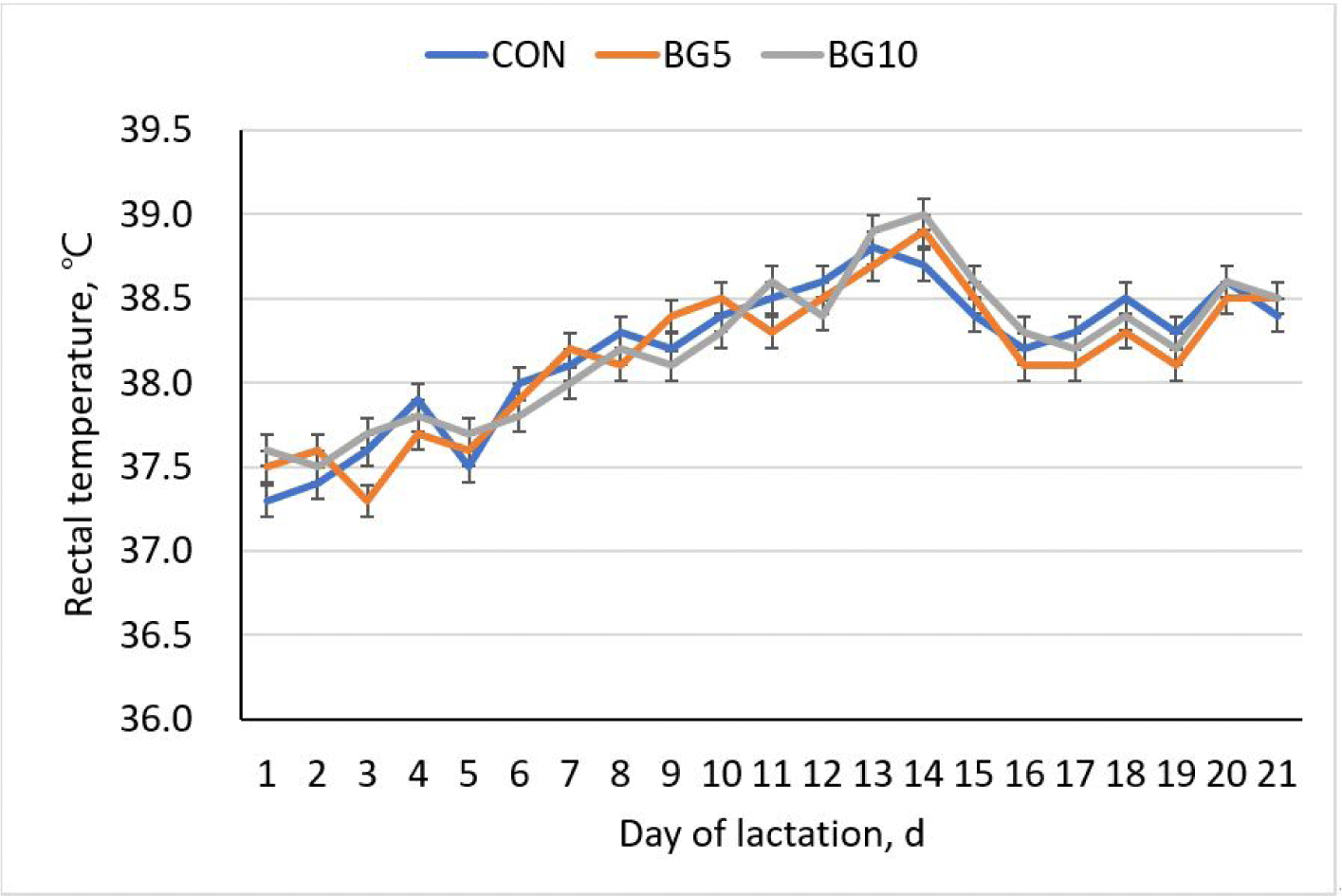
Administration of different concentrations of β-glucan did not have any significant effect on BW and BF thickness in sows, at 112 days and 24 hours after farrowing, as well as at the weaning stage. Nevertheless, no notable changes were observed in the interval between weaning and the return to the oestrus cycle across the three treatments. Moreover, BG10 resulted in higher (p < 0.05) ADFI compared to the CON and BG5 treatments (Table 2).
The addition of either BG5 or BG10 did not affect the overall count of born piglets, number of weaned piglets, or mortality rate. Moreover, no differences were observed in terms of the overall weight of the litter between the different treatments. However, at weaning, piglets from the BG10 treatment groups exhibited a higher (p < 0.05) average weight than those from BG5, although the average weight was not different from that of CON group (Table 3).
Hair cortisol levels was greater (p < 0.05) in the BG10 group than in the CON and BG5 groups (Fig. 4).
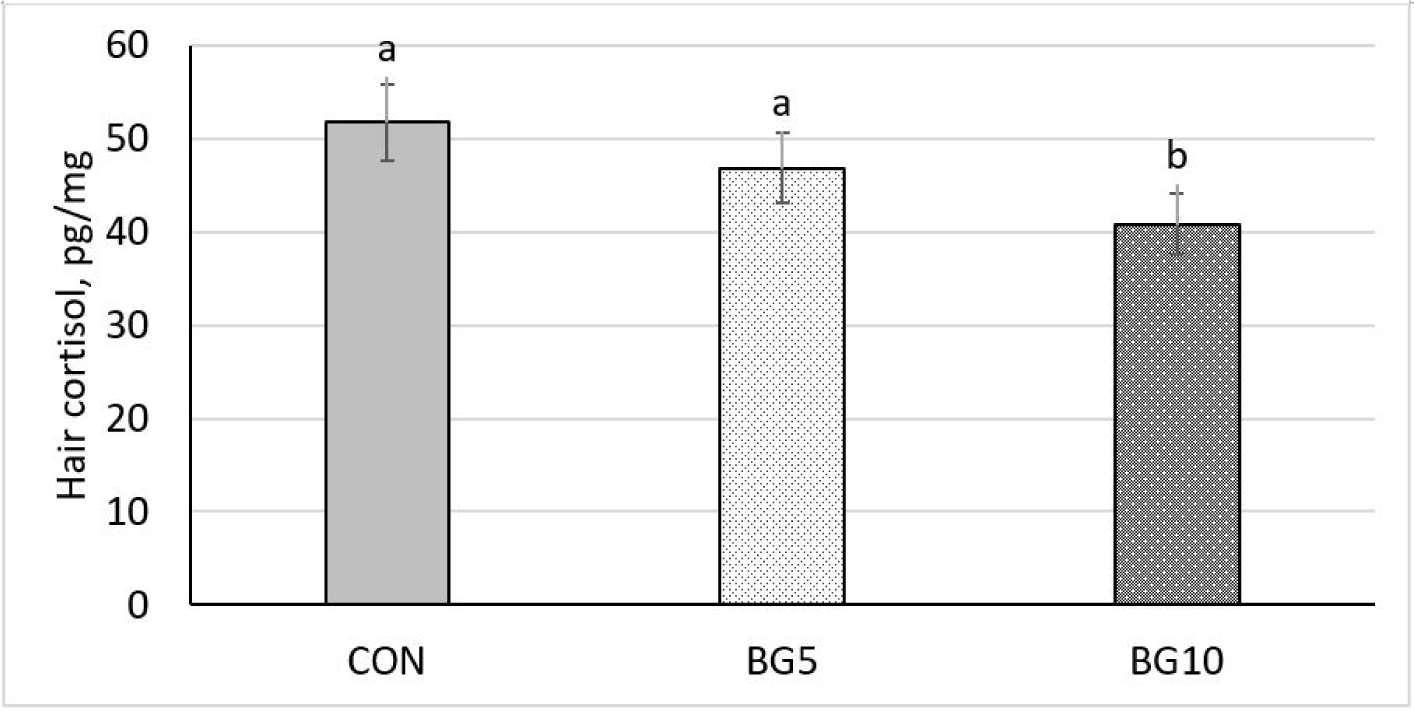
The sows in the CON group exhibited significantly higher (p < 0.05) levels of plasma TNF-α compared to both the BG5 and BG10 groups. Serum LPS levels were significantly lower (p < 0.05) compared to the CON and BG5 treatments. Nevertheless, PBP content was not statistically different across the three treatments (Fig. 5).
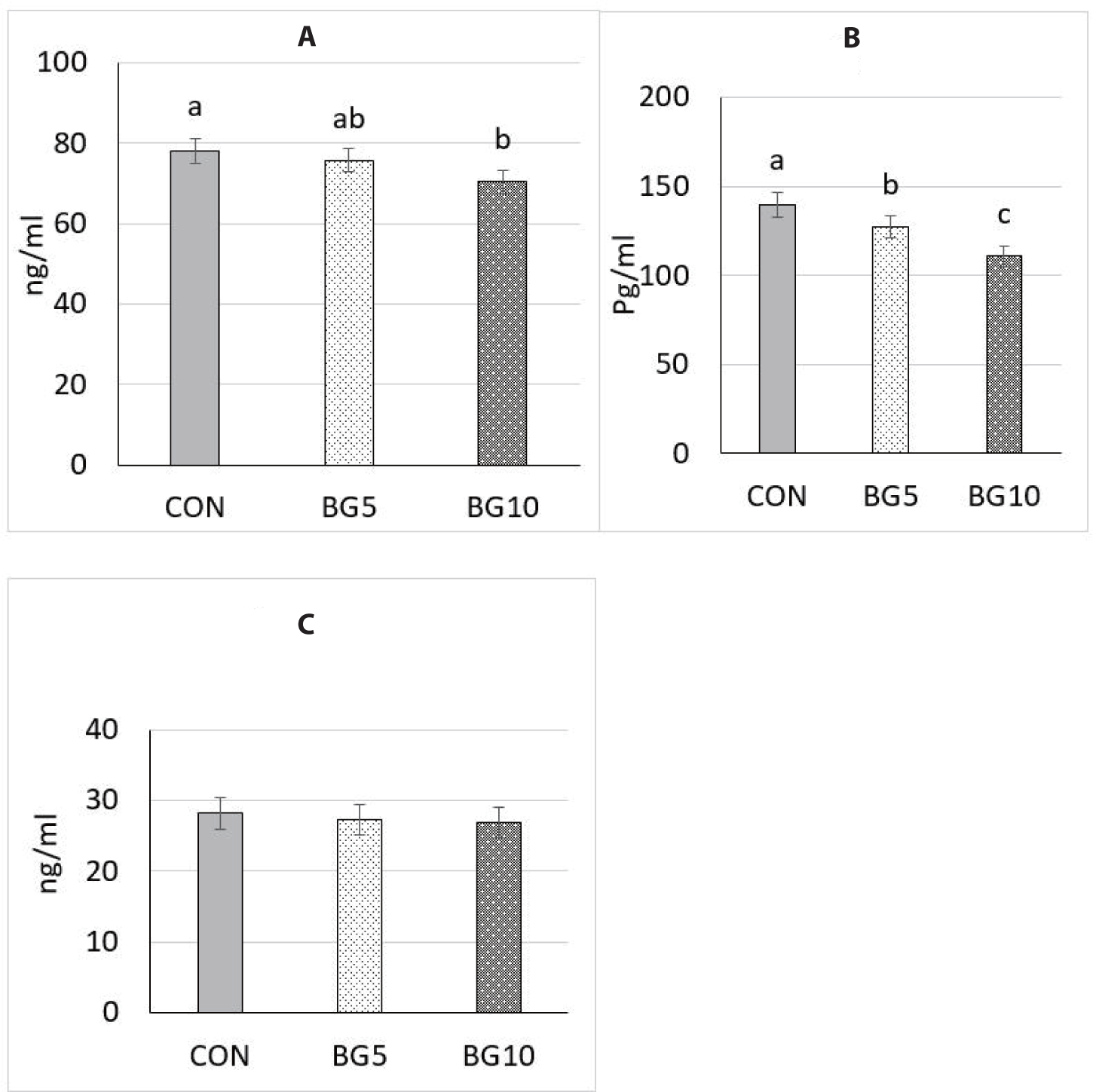
The levels of ROS, H2O2, TAC, MDA, and catalase did not change significantly throughout the experiment. However, the SOD levels were lower (p < 0.05) in the BG10 groups than in BG5, although they did not differ from the CON group (Table 4).
DISCUSSION
Breathing frequency is an important parameter for evaluating stress, and a reduced RR indicates that the muscle movements of the pigs are relaxed, reducing their heat-generation process. Heightened heat sensitivity in sows results from a lower lung-to-body size ratio, coupled with the inability of animals to sweat, and leads to increased vulnerability at high temperatures [5]. In addition, sows produce more CO2, which leads to a drop in blood pH levels [14]. The RR and vasodilation form part of a thermoregulatory strategy in sows to enhance surplus heat released into the surroundings during periods of elevated temperatures [1,3]. Increased RR increases lung evaporation and heat loss [36,37]; these processes cause pigs to cease eating, considering that feed intake contributes to further heat production via energy metabolism [9]. Other obvious signs of HS in pigs are divisions within the pen, as pigs try to increase contact with the floor to cool down, as well as splashing water or fighting for drinkers. In this study, on days 18–21 of lactation, the average RR of sows in the control diet was around 71 mov/min; however, in the BG5 and BG10 groups, RR was ~68 mov/min, indicating reduced RR by supplementing β-glucan. Yeast β-glucan is a prebiotic, therefore it was hypothesized to influence gut microbiota in the digestive tract stimulating the growth of beneficial bacteria [24,38]. These bacteria can produce short chain fatty acids (SCFAs) such as butyrate that have inflammatory and immunomodulatory impact [20]. These SCFAs can enter the bloodstream and affect the systemic immune response [39,40] thereby improving the intestinal barrier function and preventing the translocation of harmful bacteria or toxins to the lungs [17], resulting to the reduced inflammation and oxidative stress in the respiratory tract which enhances lung function and preservation of energy [15, 38].
Rectal temperature in sows is a measure of core body temperature and is influenced by various factors, including metabolic rate, ambient temperature, and the body thermoregulatory mechanisms [36]. However, in this study, differences were not significant across all treatments, indicating lack of direct involvement of β-glucan in the regulation of rectal temperature. This result could be attributed to inability of β-glucan to influence thermoregulation process [34], a mechanism by toll-like receptors that are stimulated to produce pro-inflammatory cytokines, such as interleukin-1 beta (IL-1β) and tumour necrosis factor alpha [27]. These cytokines can act on the hypothalamus to regulates body temperature [41].
BW enhances the overall health and reproductive performance of lactating sows, their energy reserves, as well as milk output. WEI signals the sow readiness for breeding after giving birth, which influences the herd reproductive efficiency and litter size. BF thickness provides insight into the physical state of lactating sows, influencing their ability to sustain breastfeeding, conceive, and maintain good reproductive outcomes [7]. In this study, BW and WEI tended to be higher (p = 0.091 and p = 0.077, respectively) in BG10, while BF was not significantly different across all three treatments. Similar to our result, Goh et al. [42] conducted experiments on 50 multiparous sows and observed comparable outcomes when the animal diets were supplemented with 0.1% or 0.2% β-glucan. Additionally, the BW result aligned with the results obtained by Szuba-Trznadel et al. [43] who conducted a similar experiment using varying levels of β-glucan, ranging from 0.01% to 0.03%, on sows and their litters. WEI is influenced by factors such as the duration of lactation, weight loss experienced during lactation, and number of piglets [18,44]. Notably, all treatments that incorporated β-glucan resulted in noticeably greater ADFI compared to the CON group. This result could be attributed to immune-stimulating effects of yeast β-glucan that contributed to the augmented release of gut hormones, such as cholecystokinin and glucagon-like peptide-1; these hormones regulate appetite and ADFI, hence boosting gut health, body immunity, intestinal structure, overall health, and growth performance of sows by enhancing non-specific immune functions [45,46].
Higher piglet weight at weaning was observed with the administration of 0.01% yeast β-glucan. The polysaccharide had the ability to regulate both the immune system and hypocholesterolemic response in sows [20], thereby reducing stress levels, increased ADFI, providing sufficient nutrients and fostering high milk production, translating to better nourishment of piglets and enhanced growth rates, and consequently contributing to an optimal weight at weaning in the BG10 group. These results are in agreement with a previous study by Li et al. [47] in weaned piglets, whereby the addition of 0.005% β-glucan to the diet resulted in an increase in the average daily gain of piglets. Likewise, Hiss and Sauerwein [48] reported improved average daily gain and weaning weight with the addition of 0.015%–0.03% β-glucan to the diet of piglets. Nevertheless, no significant differences in litter size or litter weight were recorded across treatments. We hypothesised that β-glucan alone does not provide considerable nutritional value in terms of essential nutrients required for growth, and the duration of this study was too short for β-glucan to exert marked effects on these parameters.
Assessment of the level of hair cortisol, a steroid hormone, in pigs serves as a stress indicator, providing information about the activity in the hypothalamic–pituitary–adrenal axis [49–51]. Hair cortisol analysis has been widely used recently because it allows the assessment of cortisol levels over long periods. Cortisol is synthesised and discharged by the adrenal glands into the circulatory system and its release follows stimulation by adrenocorticotropic hormone. Activation of hypothalamic–pituitary–adrenal axis prompts the secretion of adrenocorticotropic hormone [52]. This release is triggered when an animal experiences a stress stimulus [53]. In the current study, we observed a significant decrease in hair cortisol in the group administered the polysaccharide at 0.01% concentration. Yeast β-glucan supplementation in the diet of lactating sows reduces hair cortisol levels by modulating immunity responses, regulating hormones such as cortisol, countering inflammation, shaping gut microbiota, and improving metabolic health [16]. This multi-faceted approach fosters a low-stress physiological state and promotes the overall well-being of sows.
Inflammation in lactating sows affects nutrient utilisation, impairs milk production, and hampers piglet growth [21]. Increased levels of stress hormones and reduced feed intake are commonly observed in sows, negatively affecting overall health and productivity. In this study, β-glucan administration resulted in lower levels of inflammation markers, evidenced in downregulation of the production of TNF-α and LPS, leading to reduced stimulation of the immune system. Yeast β-glucan has been found to modulate inflammatory activity by stimulating immune cells. It enhances immune responses and promotes anti-inflammatory effects by interacting with immune cell receptors [18], thereby regulating the immune system and potentially reducing inflammation. Our results suggest that β-glucan supplementation contributed to the inhibition of the production of TNF-α and LPS in HS sows, thereby contributing to a more balanced immune system function and potentially reducing HS. Previous studies on pigs by Vetvicka et al. [54] and Eicher et al. [55], β-glucan diet had a critical role in amplifying the cellular arm of the immune system, promoting an overall biological and immunological effect. Moreover, elevated levels of TNF-α have been found to influence the allocation of nutrients to the immune system rather than promote growth [17]. This result implies that when TNF-α concentration decreased, in groups BG5 and BG10, a greater amount of nutrients could be made available for animal growth. This finding is supported by the significantly higher ADFI and improved overall health and growth outcomes among the litters.
The HS triggers oxidative stress when ROS generation exceeds the capacity of antioxidants in the body to counteract them [56]. It encompasses the production of ROS, such as superoxide and H2O2, produced by the placenta and mammary glands, which adversely affects reproductive and lactation functions [23,57]. Zhang et al. [58] conducted a study on multiparous sows and highlighted that oxidative stress leads to decreased total litter size, live litter size, and litter weight gain. Moreover, a previous study on lactating sows by Black et al. [59] found that oxidative stress causes reduced ADFI during lactation, prolonged negative energy balance, great loss of body condition, and decreased milk production in sows, ultimately affecting the piglets. Sows subjected to high-temperature stress during lactation experience oxidative stress until weaning [49]. Oxidative stress levels can be assessed using indicators such as MDA and SOD. In this study, serum SOD levels were higher in the groups with β-glucan treatments, which indicated improved antioxidant capacity and reduced stress. This finding suggests that supplementation of β-glucan modulates the antioxidant defence system in HS animals and eventually increases ADFI, which is a sign of reduced oxidative stress. This effect is likely due to the capacity of yeast β-glucan to inhibit the proliferation of detrimental microorganisms in the intestine, fostering improved intestinal well-being, increased populations of beneficial microbes, and diminished presence of opportunistic pathogens [53].
CONCLUSION
Our study showed the effectiveness of yeast β-glucan in ameliorating the adverse effects of HS due to lower RR and hair cortisol. We found that β-glucan lowered TNF-α, LPS, and SOD levels and increased ADFI and piglets weight gain. Therefore, the supplementation of yeast β-glucan at 0.01% in lactating sows diet is recommended during high ambient temperature.