INTRODUCTION
Previous studies provide documentations of promising role of L-tryptophane (L-T) in improving performance, yields, and stress relief in both forms of rumen non-protected [1] and rumen protected L-T in beef [2–4] and in dairy cows [5]. The L-T supplementation in ruminants has been studied for its potential to improve growth performance, feed efficiency, and nitrogen utilization [1,2]. L-T is an essential amino acid (AA) that plays a role in protein synthesis and is a precursor to serotonin, which has been linked to various physiological functions, including appetite regulation and stress response [3,5]. Some studies suggest that L-T supplementation may improve nutrient digestibility, increase microbial protein synthesis, and reduce ammonia emissions from manure, which could have environmental benefits [4,5]. However, the exact mechanisms by which L-T supplementation affects ruminant metabolism are still not fully understood and require further research. Given these, supplementation of L-T at an optimum level could help the animals to outperform and to be cost-effective. In a recent in vitro investigation, Conejos et al. [6] concluded that L-T could increase protein synthesis in MAC-T cells by stimulating the genes, proteins, and protein synthesis-related pathways involved in energy and protein syntheses.
Given that 58 to 82% of starch is digested in the rumen by ruminant microorganisms, while 18% to 42% of undigested starch enters the small intestine [7]; induced starch digestion in rumen [2] and small intestine [1,4] is an aimed scenario to acquire high meat yield and quality particularly in the late stage of fattening period in steers. It is while the small intestine is capable of a 42% higher absorption rate than the rumen, the secretion and vitality of the starch-degrading enzyme α-amylase is low (35% to 60%) [8]. Owens et al., [7] suggested four possible reasons for this limitation; 1) restriction of enzyme activity for starch digestion, 2) limitation of the amount of glucose absorbed from the small intestine, 3) insufficient time to complete starch digestion, and 4) insufficient enzyme approach in starch granules. Tryptophan in form of rumen-protected has been practiced inducing starch digestion in small intestine in very few studies [2]. In a previous study [2], 191.1mg/kg body weight (BW) was added to improve the digestibility of starch in the small intestine. However, due to the cost of rumen-protected L-T (RPL-T), the optimum low dosage which can be beneficial for both purposes of being cost-effectively profitable and highly influential and possible physiological and hormonal reasons leading to the phenomenon requires further investigation.
L-T is known as a precursor or stimulator of serotonin, melatonin (MEL), and cholecystokinin (CCK) [1,2,4]. L-T is believed to induce gastro-intestinal hormones (GIH) and MEL in not only humans [9], but also chicken [10], rat [11], cow [5,12], and goat [13].
Hence, we aimed to find out the lowest optimum level of RPL-T showing the highest possible effectiveness in Holstein steers and for economical perspective. Therefore, this study designed to evaluate the different dosage of RPL-T which could induce starch digestion and stimulate gastro-intestinal tract (GIT) hormones in Holstein steers for the purpose of higher beef yield and quality.
MATERIALS AND METHODS
All experimental procedures were in accordance with the “Guidelines for Care and Use of Experimental Animals” of Pusan National University (approval no.: PNU-2010-000152).
The experimental animals used in this study were four Holstein steers (average BW = 201 ± 24 kg). The animals were housed in a 4 × 5 m fenced and concrete-floored barn where individual specifications were possible, and water was provided ad libitum throughout the experiment. In order to minimize the effect of light on the secretion of MEL, all passages in the barn were closed the day before each period to block the light. Animals were weighed before each treatment period, and the basic feed was fed by calculating the daily intake of steers according to the NRC [14] standard, which specifies the daily nutrient requirements according to the BW and daily gain. Feed amount by metabolic energy intake was determined to convert by dry matter (DM kg) value, crude protein content (%) and total digestible nutrients (TDN) content (%) of forage feed (timothy) and concentrated feed to meet the maintenance requirements for cattle [14]. The chemical composition of the basal feed is shown in Table 1, and the ratio of forage to concentrate feed was supplied at an average ratio of 41.8:58.2. The basal feed was fed twice a day at 9:30 am and 5:30 pm.
Moisture | Crude protein | Ether extract | Crude ash | TDN | |
---|---|---|---|---|---|
Timothy | 9.24 ± 0.09 | 7.74 ± 0.07 | 1.14 ± 0.01 | 5.66 ± 0.01 | 57.43 ± 0.07 |
Concentrate | 10.06 ± 0.07 | 15.77 ± 0.09 | 3.94 ± 0.02 | 7.46 ± 0.02 | 68.21 ± 0.13 |
RPL-T used in this study was RPL-T (42% L-Tryptophan, Beijing Feeding Feed Science Technology, Beijing, China), which is a rumen protection L-T preparation. The RPL-T was a granular product in the form of wrapped 42% AAs in a resin material, which 37.7% fat and 20.3% carbohydrates are composed of complex organic acids and their derivatives. In the control group, an excipient consisting of 65% fat and 35% carbohydrate was used to eliminate the effect of the remaining 37.7% fat and 20.3% carbohydrate, not 42% RPL-T in the treatment group. After applying the BW of 57.8 mg/kg BW [2], 42% of RPL-T was converted to 100%, and in addition the average absorption rate 72% [15] of AAs in the small intestine in ruminants was converted to 100%. Therefore, the amount of 42% RPL-T (treatment group) was added by calculating to the BW of 191.1 mg/kg BW. The amount of excipient (control group) added was calculated as 58% of the value obtained by converting 42% of RPL-T into 100% and was added after converting the average AA absorption rate of 72% in the small intestine in the same way as in the treatment group. Table 2 shows the chemical compositions of RPL-T used in this study.
Moisture | Crude protein | Ether extract | Crude ash | |
---|---|---|---|---|
RPL-T | 0.58 ± 0.00 | 35.81 ± 0.09 | 50.81 ± 0.02 | 0.37 ± 0.00 |
The design was 4×4 Latin square using four Holstein steers, and only basic feed was fed for 7 days before given treatment as a feeding adaptation period. After adaptation, the control was added to the basic feed (41.8% timothy + 58.2% concentrate) at excipient and the treatment (42% RPL-T) was added to the basic feed at 191.1 mg/kg BW (100%), and 95.6 mg/kg BW (50%), and 19.1 mg/kg BW (10%). The treatment groups were treated for 5 days in each period, and a total of 4 periods were performed.
Blood samples were taken from the jugular vein for analysis of serum intestinal hormones, synthetic hormones, and metabolite profile. The 0 days not processed for analysis, and 9:00 (~30 min), 11:00 (90 min), 13:00 (210 min), 15:00 (330 min) for each hour on days 1, 3, and 5 of treatment at 17:00 (450 min), sampling was performed 5 times at 2 h intervals to confirm the change pattern by date. Blood collection at 09:00 was performed 30 min before morning feed. Blood was collected and placed in the serum tube (BD Vacutainer, BD, Franklin Lakes, NJ, USA) and the serum was obtained by blood centrifuging at 2,700×g for 15 min. The serum supernatant was dispensed in 1.5 mL each tube and stored at −80°C until analysis.
Feces samplings were performed 5 times on the 5th d at 9:00 (feeding before 30 min), 11:00 (after feeding 90 min), 13:00 (after feeding 210 min), 15:00 (after feeding 330 min), 17:00 (after feeding 450 min), the same as blood collection; and to analyze the digestibility, the weight of the feces was measured for 12 h.
Serum concentrations of secretin and CCK-8 were determined by enzyme immunoassay of secretin (EK-067-05) and CCK-8 (EK-069-04) (Phoenix Pharmaceuticals, USA) using a plate with secondary antibody attached thereto. MEL analysis was performed using the MEL human ELISA Kit (RE54021) (IBL, Hamburg, Germany), which can be quantitatively measured on a plate by enzyme immunoassay.
For the analysis of the D-glucose content in the feces, the anthrone method of Roe [16] and McCready et al. [17], in which D-glucose is colored with anthrone using a standard reagent, were used.
In order to check the amount excreted from the amount of feed consumed per day, the amount of excreted over 2 days was averaged and measured. Based on the NRC [14] standard, which specifies the daily nutrient requirements, the daily intake was calculated by calculating the coarse and concentrated feed.
Starch digestibility analysis for economic evaluation was analyzed by the following calculation formula:
(1) Starch (Fecal flow, g/d) = (D-glucose, μg/mL) × 1,000,000 (g/mL) / 1,000 (g/kg) × (Fecal flow, g/d)
(2) Starch (Disappearance, g/d total tract) = (Starch feed intake, g/d) – (1)
(3) Starch (Disappearance, % total tract, of intake) = (2) / (Starch feed intake, g/d) × 100
(4) Starch (Profit g/d) = (2) Treatment – (2) Control
(5) Starch (Profit won/d) = (4) × 0.5 won (Starch/g)
Data obtained through the experiment were analyzed for using ANOVA procedure by SPSS (version 16 SPSS, Chicago, IL, USA). In addition, Duncan’s New multiple range test was used to test the significance of multiple treatment intervals. The significance level was evaluated when p < 0.05.
RESULTS AND DISCUSSION
The GI hormones including CCK, secretin and MEL concentration by day (0, 1, 3, 5) and time (9:00, 11:00, 13:00, 15:00, 17:00) in serum of Holstein steers fed diets are presented in Fig. 1 and Table 3. The GI related hormones that stimulate the rate of feed digestion including CCK, MEL and secretin are known to be associated with L-T [1–3,5]. The CCK was the highest in the group treated with RPL-T 191.1 mg/kg BW on the 3rd d (p < 0.05), while the groups of 95.6 mg/kg and 19.1 mg/kg were equally significantly higher than the control group (p < 0.05). However, on the 5th d, 191.1 mg/kg treated with RPL-T showed a decreasing pattern, and with respect to the 95.6 mg/kg and 19.1 mg/kg treatment groups, the concentration continued to increase. Regarding secretin, the concentration was not changed according to the treatments. As a result of analyzing the pattern by time (Fig. 1 and Table 3), in the case of CCK, the group treated with 191.1 mg/kg was significantly higher at 11:00 and 15:00 than the control group (p < 0.05), and 95.6 mg The /kg treatment group showed a higher value than the control group at 13:00 (p < 0.05). However, secretin could not confirm the pattern by time and the difference between the treatment groups were not significant. According to Jaworek et al. [18], high-dose intraperitoneal injection of L-T in rats may play a protective role against severe pancreatic injury and significantly increased plasma MEL levels. The endocrine hormone CCK, which directly affects the secretion of pancreatic enzymes, is also related to the intake level of uncyclized AAs [19]. Leja-Szpak et al. [20], reported that L-T supplementation to rats led to an increased production of α-amylase and had an increased effect on the synthesis of MEL, a pineal hormone, and MEL. The endocrine hormone, CCK, reported to have a close relationship with L-T. In the pancreatic islet of Langerhans cells, the effect of MEL is regulated through a specific MEL MT2 receptor [21] and increases duodenal bicarbonate secretion by exogenous MEL produced endogenously from L-T [22], where it stimulates the secretion of pancreatic amylase. They suggested that the mechanism MEL function in the pancreas is indirect and dependent on the secretion of CCK and the activation of afferent vagus nerve initiating pancreatic reflexes in the intestine [1,10,11]. CCK appears to serve as a neurotransmitter and neuromodulator and is abundantly distributed throughout the mammalian brain [20]. CCK suppresses feeding behavior and stomach function by acting as a paracrine modulator of vagal afferents in the periphery, particularly in the duodenum [18,20]. CCK may function directly within the CNS to stimulate central vagal afferent terminal inputs to the solitary nucleus, according to current research [20]. CCK mediates pancreatic enzyme secretion via cholinergic pathways, according to recent experimental findings in animals and humans. These findings suggest that CCK’s ability to promote pancreatic secretion is dosage dependent. As a result of intestinal hormone analysis, there was no difference between RPT-L treatment groups. Therefore, from an economic point of view, it should be considered as a recommended level of 19.1 mg/kg BW addition.
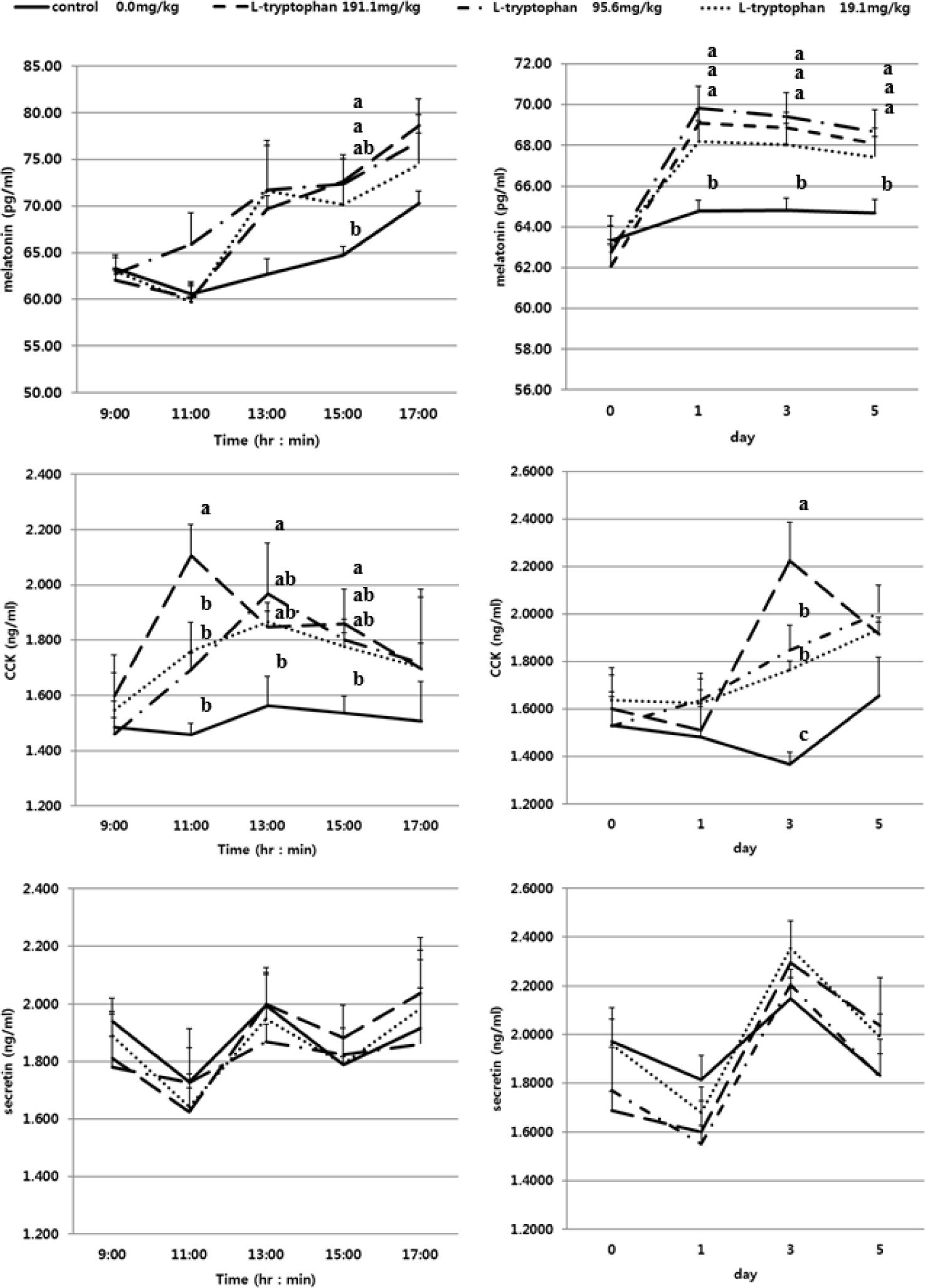
The D-glucose content in the RPL-T treatment groups was significantly reduced (p < 0.05) compared to the control group from the 3rd d of treatment, and there was no difference (p > 0.05) between the RPL-T treatment levels on the 3rd d (Fig, 2A). D-glucose content was significantly decreased at 191.1 mg/kg BW and 95.6 mg/kg BW compared to 19.1 mg/kg BW on the 5th d (Fig. 2A). As a result of examining the average D-glucose content in the feces after treatment with RPL-T for 5 d, the D-glucose content was significantly decreased by RPL-T treatment than the control (p < 0.05); however, no difference (p > 0.05) was observed by the treatment dosage. (Fig. 2B), which favors the lower level of supplementation for economical purposes. It has been reported that ghrelin, MEL, and tryptophan increase the secretion of CCK and starch in mono-gastric animals [18,20,23], and ruminants [2,3]. In general, MEL is endogenously produced from L-T and is known to strongly promote pancreatic secretion [11,20]. The following research in mono-gastric animals (dogs and rats) and ruminants (Hanwoo steers) is consistent with the obtained results in this study. In dogs, L-T strongly stimulates exocrine pancreatic secretion in most cases when jugular vein infusion or duodenum is supplied, whereas when this AA enters the ileum and when applied, pancreatic secretion is significantly inhibited [24]. In rats, dose-dependent administration of L-T significantly increased plasma MEL concentrations and pancreatic enzyme secretion [11,23], suggesting that endogenous MEL produced from L-T in the intestine may also promote pancreatic exocrine function [11,18]. In ruminants, administration of L-T significantly increased serum MEL, CCK concentrations [2,3] and the digestibility of starch in the small intestine was improved [2]. Secretion of intestinal CCK which observed in this study following RPL-T supplementation, eventually enhanced the activity of pancreatic α-amylase and decreased fecal D-glucose contents [20]. Li and Owyang [25] showed that CCK secretion can stimulate acinar cells in the pancreas by the afferent pathway of the pneumogastric nerves to stimulate the secretion of pancreatic fluids which results in higher digestion of peptides, AAs and starch in small intestine. As a result of the starch degradation analysis in Fig. 2B, there was no difference between the RPT-L treatment sections. Therefore, from an economic point of view, it should be considered as a recommended level of 19.1 mg/kg BW addition.
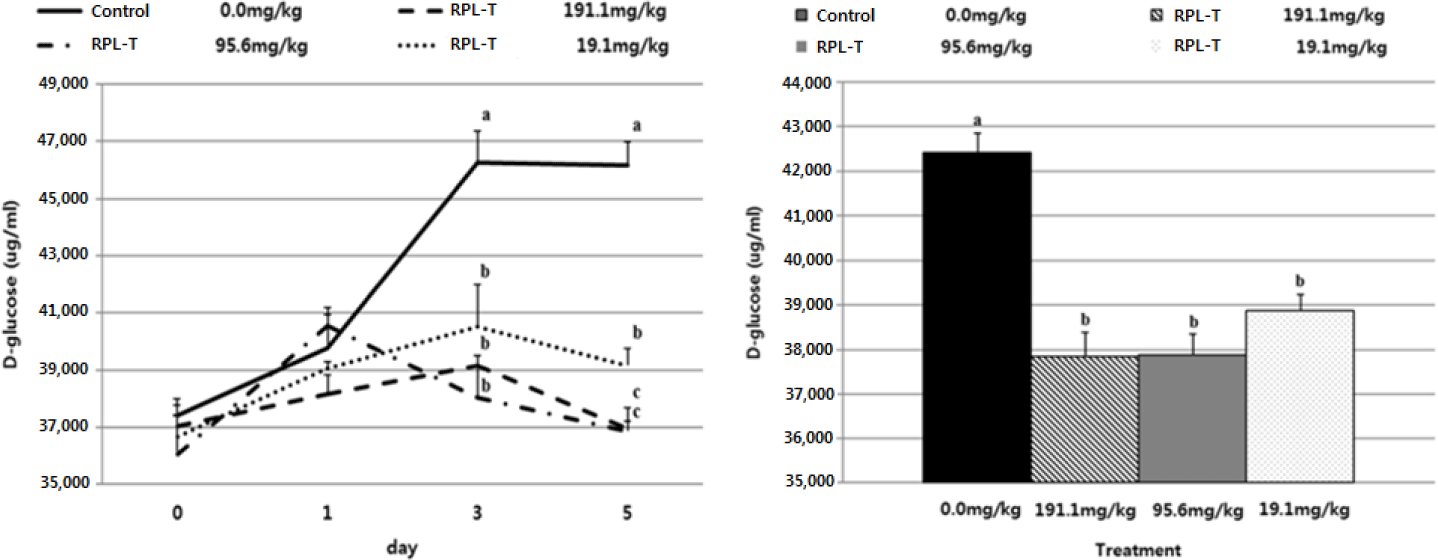
Table 4 shows the apparent starch disappearance rates in GI track in Holstein steers fed basal diet and different concentrations of RPL-T. The control group was fed 6,940 ± 199.1 g/d (DM) and excreted a total of 7,075 ± 462.1 g/d (wet matter). RPL-T 191.1 mg/kg fed 7,660 ± 141.6 g/d of feed and excreted 5,600 ± 556.5 g/d, so the proportional value was 0.73 ± 0.076%, which was significantly lower than that of the control group (p < 0.05). However, for RPL-T 95.6 mg/kg and 19.1 mg/kg, the feed intake-proportional excretion values were 0.89 ± 0.034% and 0.91 ± 0.056%, respectively which were tended to decrease compared to the control group. The total daily intake of starch was 4,164 ± 119.5 in the control group, while in RPL-T 191.1 mg/kg was 4,596 ± 85.0, in 95.6 mg/kg was 4,686 ± 53.9, and in 19.1 mg/kg was 4,476 ± 77.1 found to be ingested (p < 0.05). However, the excreted total daily starch content was the highest in the control group at 313 ± 48.9 g/d, and in the RPL-T 191.1 mg/kg was 160 ± 30.7, which was significantly decreased compared to the control group (p < 0.05). As a result, the total daily starch digestibility was 3,851 ± 90.3 g/d for the control, RPL-T 191.1 mg/kg 4,436 ± 95.2, 95.6 mg/kg 4,449 ± 73.0, and 19.1 mg/kg BW 4.232 ± 71.3 g/d. Digestion was increased in all treatment groups compared to the control group (p < 0.05), and the total daily starch degradability was significantly increased (p < 0.05) in RPL-T 191.1 mg/kg and 95.6 mg/kg compared to the control group. RPL-T 19.1 mg/kg did not increase significantly, but it was 94.58%, which showed higher starch degradability than the control (92.53%). As a result, the RPL-T treated group showed higher starch degradability than the control group (p < 0.05), so it was confirmed that the starch utilization and digestibility were improved by the RPL-T treatment. In agreement with the above-mentioned mechanism, Richards et al. [26] suggested that protein infusing when accompanied with starch into small intestine enhances starch disappearance in small intestinal. This could be a phenomenon observed in this study by addition of RPL-T in the treatment groups. Additionally, dietary supply of AAs can lead to influx in lower intestines resulting in higher growth in ruminants [27].
CONCLUSION
In summary, while the RPL-T 191.1 mg/kg BW feeding level showed significant influence in a previous Hanwoo study, our Holstein study indicates that a reduced dosage of 19.1 mg/kg BW, representing 1/10th of the previous level, remains impactful. This reduced dosage promotes the secretion of MEL and CCK in the blood, resulting in comparable starch degradability. Through analysis of intestinal hormones and starch degradation, no significant differences were observed among the RPL-T treatment groups. Consequently, from both a biological and economic perspective, we recommend the supplementation of RPL-T at 19.1 mg/kg BW as an optimal and sustainable level.