INTRODUCTION
In the livestock industry, the in vitro production (IVP) of embryos stands as a pivotal technology with widespread applications in transgenic animals, particularly in the advancement of interspecies transplantation and the development of human disease models. However, embryos generated through IVP technology are susceptible to damage induced by reactive oxygen species (ROS) originating from the supplementation of culture medium and other factors [1,2]. Increased levels of ROS, including hydrogen peroxide and hydroxyl radicals, are prominently associated with oocyte senescence [3], a phenomenon that impedes the maturation of mammalian oocytes during the post-ovulation secondary meiosis (metaphase II [MII]). Oxidative stress triggered by ROS and senescence contributes to spindle abnormalities, disorders in chromosomal condensation, and mitochondrial alterations, culminating in aberrations in gene and protein expression. These anomalies, in turn, exert detrimental effects on fertilization and subsequent embryonic development [4].
The utilization of antioxidants in the culture medium represents an effective strategy for mitigating the detrimental effects of oxidative stress. Extensive research endeavors have been dedicated to identifying beneficial components, particularly antioxidants, aimed at enhancing assisted reproductive technology (ART) outcomes [5]. Notable findings have emerged from studies investigating various animals and plants, revealing the presence of antioxidants, anti-inflammatory agents, and anticancer agents [6,7]. Research has been conducted specifically on the antioxidative properties of fish eggs, including those from salmon, sardine, and carp [8,9]. In human dermal fibroblasts treated with salmon roe extract, differential regulation of TXNRD1, OXR1, and PRDX family genes, alongside collagen type I genes, were observed [10]. Additionally, several antioxidant genes were identified in large uniflagellar liposomes (LUVs) derived from sardine eggs [8]. Reports on defatted egg hydrolysate (CDRH) from Cyprinus carpio (carp) eggs indicated antioxidant and anti-inflammatory activities, as well as antioxidant and antibacterial activities in the lipidic fish roe extracts (designated fishroesomes) [9]. Among various fish eggs, our particular interest is in Paralichthys olivaceus eggs (POE), a prominent component of Jeju. Studies on POE have elucidated the role of germline alpha factor (FIGLA), a basic helix-loop-helix (bHLH) transcription factor, in the early stages of muscle ovarian development and overall ovarian differentiation in Paralichthys olivaceus [11, 12]. Notably, Paralichthys olivaceus stands as one of the best-selling fish species in Jeju Island, commanding 98% of the domestic aquaculture market with an annual production exceeding 25,000 tons and exports surpassing 4,000 tons [11]. Despite this, approximately 9,000 tons of POE are estimated to be wasted annually.
In a study focused on Paralichthys olivaceus, α-chymotrypsin hydrolysate of flounder fish muscle (FFM) was evaluated against 2,2-diphenyl-1-picrylhydrazyl (DPPH) and peroxyl radicals. Two novel protease-digested antioxidant peptides, Val-Cys-Ser-Val (VCSV) and Cys-Ala-Ala-Pro (CAAP), obtained from FFM hydrolysates, exhibited potent antioxidant activity [11]. Another study revealed a significant increase in the mRNA expression of antioxidant-related genes (SOD, CAT, and GST) in POE under conditions of seawater acidification and exposure to cadmium [13]. Despite Paralichthys olivaceus being recognized for its antioxidant properties, the direct effects of POE on mammalian oocytes or ce--lls have not been explored, although numerous experiments pertaining to its functions in Paralichthys olivaceus production have been conducted. Consequently, while POE’s antioxidant potential is well-established, its effects remain unexplored in the context of mammalian cells and oocytes.
This study aimed to assess the antioxidant efficacy of POE extract (POEE) components within a peroxidative environment during in vitro conditions. The investigation focused on evaluating the effectiveness of nuclear maturation, embryonic development, and the expression of development-related genes in porcine oocytes. The experimental outcomes substantiate the antioxidant potential of POE during in vitro maturation (IVM), indicating its potential influence on the utilization of POEE.
MATERIALS AND METHODS
All chemicals and reagents utilized in the study were procured from Sigma (St. Louis, MO, USA), unless explicitly mentioned otherwise.
An ethanol (EtOH) extract of POE was obtained by immersing 1 g of POE in either 100 mL of 30% ethanol (30E) or triple-distilled water (DW). The mixture was then subjected to agitation on a shaker at 25°C for 30 minutes. Subsequently, EtOH was removed using a rotary vacuum concentrator, and the resulting samples were filtered through 90 mm filter paper before undergoing freeze-drying.
To analyze the components of POEE, chromatography was conducted using a QP2010 instrument (Shimadzu, Kyoto, Japan) equipped for gas chromatography coupled with mass spectrometry (GC-MS). The chromatographic analysis employed an OV-5 analytical column (30 m × 0.25 mm internal diameter, 0.25 μm film thickness, comprising 5% phenyl and 95% dimethylpolysiloxane), with helium serving as the carrier gas at a flow rate of 1.19 mL/min. The injector and detector temperatures were set at 220°C. The initial column temperature was set at 40°C, with subsequent increments to 240°C at a rate of 6°C/min.
Retention indices for each component were calculated, and the identification of compounds was accomplished by correlating their retention indices with those of hydrocarbon patterns analyzed under identical conditions. The similarity between the mass spectrum of each chemical component and the mass spectra of the NIST 14 library was assessed for identification purposes.
DPPH demonstrates pronounced absorption at 517 nm due to its possession of an odd number of stable radical electrons. When engaging with an electron donor, such as a polyphenol, that provides electrons to hydrogen, DPPH undergoes a reaction wherein the electrons receive hydrogen radicals, generating phenoxy groups. The resultant radicals amalgamate to form a stable molecule, leading to a reduction in absorbance at 517 nm. The donated electrons form irreversible bonds, causing the purple color of DPPH to diminish in correlation with the number of electrons, ultimately resulting in decreased absorbance.
POEE 30E and DW samples were stratified into 0–2, 24, 48, and 50–60 h post-fertilization groups. These samples were then diluted in dimethyl sulfoxide (DMSO), and dilutions (2,000, 3,000, 4,000, 5,000, 6,000, 7,000, 8,000, 9,000 ppm) were prepared from a 0.01 g/mL stock solution. Subsequently, 20 µL of each sample, diluted in DMSO, was introduced to 180 µL of a 167 µM DPPH working solution. The absorbance of the resultant solution was recorded at 517 nm, and the DPPH free radical scavenging activity was determined using the designated equation:
The DPPH free radical scavenging activity was quantified by determining the inhibitory concentration at which 50% activity was observed (IC50). This value was derived from the trend line, which was constructed using a specific equation.
Pre-adolescent porcine ovaries were procured from a local abattoir and promptly transported to the laboratory within a 2-hour timeframe at a temperature range of 33°C–35°C. During transit, a saline solution enriched with 75 μg/mL penicillin G and 50 μg/mL streptomycin sulfate was utilized. Cumulus-oocyte complexes (COCs) were retrieved from follicles with diameters ranging from 2 to 8 mm, employing an 18-gauge needle and a disposable 10 mL syringe. Subsequently, the COCs underwent a washing procedure in tissue culture medium (TCM)-199-hydroxyethyl piperazine ethane sulfonicacid–(HEPES), containing 0.1% (w/v) bovine serum albumin (BSA).
The maturation of COCs occurred in a 500 μL IVM media TCM-199 (Gibco, Waltham, MA, USA) comprising Earle’s salts, cysteine (0.57 mM), 10 ng/mL luteinizing hormone, and 10% (v/v) porcine follicular fluid, all conducted under mineral oil for a duration of 42–46 hours at 38.8°C in an environment of 95% air and 5% CO2.
To induce peroxidation in oocytes, treatment involved exposure to 200 μM H2O2 for a duration of 44 hours in TCM-199 (Gibco) – folic acid (FA) treatment. Prior to maturation, 100X FA stock concentrations, prepared in DMSO, were diluted in IVM media (final volume of 500 μL) for the treatment of 50 oocytes, all covered with mineral oil as previously described. Germinal vesicle (GV) stage oocytes were incubated in IVM media containing 0.1, 0.2, 0.3, or 0.4 mM FA. Control groups underwent concurrent treatment with H2O2, and all samples were incubated for 44 h as outlined above. Following the treatment period, oocytes were collected, and peroxidation was subsequently evaluated.
Preceding the maturation process, GV stage oocytes were transferred to TCM-199 containing varying concentrations of POEE - specifically, 600, 650, 700, or 750 ppm. Simultaneously, control groups were subjected to treatment with H2O2. The samples, encompassing both experimental and control groups, underwent an incubation period of 44 hours, following the previously described conditions. Subsequent to the treatment duration, oocytes were harvested, and peroxidation assessments were conducted.
After maturation, cumulus cell removal was performed by pipetting with 1 mg/mL hyaluronidase for 5 minutes. Parthenogenetic activation (PA) involved exposing oocytes to porcine zygote medium (PZM)-5 with 0.4% (w/v) BSA (IVC medium) and treating them with 5 μM Ca2+ ionomycin (Sigma) for 5 minutes. Subsequently, after a 3–6 hour culture in IVC medium containing 7.5 μg/mL cytochalasin B (Sigma), embryos underwent a washing step in the same medium and were cultured for 7 days at 38.8°C in a humidified atmosphere of 5% CO2 and 95% air.
And after incubation culture, both oocytes and embryos underwent washing in Dulbecco’s phosphate-buffered saline (DPBS) containing 0.1% (w/v) BSA (0.1% B-PBS). Subsequently, they were fixed in 4.0% (w/v) paraformaldehyde for a duration of 20 minutes. Depending on the experimental protocol, specimens were either stored at 4°C or subjected to snap-freezing in liquid nitrogen and stored at −70°C. The survival rate of oocytes was determined through the observation of denuded MII oocytes before activation under a microscope.
Intracellular levels of glutathione (GSH) and ROS were assessed using CMF2HC and DCFHDA, respectively, as previously outlined with slight modifications [12,13]. Cumulus cells were removed from COCs by pipetting with 0.1% (w/v) hyaluronidase. Denuded oocytes were subsequently incubated in DPBS with either 100 μM CellTracker™ Blue CMF2HC or 50 μM DCFHDA in the dark for 20 minutes at 38.8°C. Following incubation, the oocytes underwent a washing step with DPBS containing 0.1% (w/v) BSA (0.1% B-PBS) to completely remove excess dye. Subsequently, analysis was performed via epifluorescence microscopy (Olympus IX71, Olympus, Tokyo, Japan). The excitation and emission wavelengths for CellTracker™ Blue CMF2HC were 371 and 464 nm, respectively, while ROS levels were assessed at excitation and emission wavelengths of 450–490 and 515-565 nm, respectively. Grayscale images were captured using a digital camera (Olympus DP71, Olympus IX71) attached to the microscope, and mean grayscale values were calculated using ImageJ software (NIH, Bethesda, MD, USA). The intensities of the control group were standardized to 1. The experiment was independently replicated eight times, each involving 10–12 oocytes per iteration.
The visualization of meiotic spindles and oocyte nuclei post-maturation was performed. Cumulus cells were extracted from porcine COCs matured for 42–46 hours, and oocytes were fixed overnight at 4°C with 4.0% (w/v) paraformaldehyde in PBS. Subsequently, the fixed oocytes were incubated with 0.5% (v/v) Triton X-100 at 38.8°C for 30 minutes. After blocking for 1 hour with 1% BSA (w/v) in PBS (blocking solution I), the oocytes were cultured overnight with an Alexa Fluor 488-conjugated anti-α-tubulin antibody (Sigma), diluted at 1:200 in blocking solution I, at 4°C. Oocyte nuclei were stained with Hoechst 33342 (1 μg/mL) for 30 minutes. Finally, the oocytes were washed with PBS containing 0.1% (w/v) BSA, mounted onto glass slides, and examined under an inverted Olympus IX-71 microscope (Olympus). Each group comprised a minimum of 20 examined oocytes.
On the seventh day following PA, blastocysts were fixed in DPBS containing 4% paraformaldehyde (4% PFA) at 38.8°C overnight (O/N). Fixed blastocysts were washed three times with 0.1% B-PBS and then incubated with 0.1% Triton X-100 at 38.8°C for 30 minutes. Subsequently, blastocysts were incubated with fluorescein-conjugated dUTP and terminal deoxynucleotidyl transferase, using an In situ Cell Death Detection Kit (Roche, Manheim, Germany), in the dark at 38.8°C for 1 hour. The scoring of mitotic and apoptotic cells was performed. Nuclei were stained with Hoechst 33342 (1 μg/mL) at 38.8°C for 30 minutes, and blastocysts underwent three washes with 0.1% B-PBS. Finally, blastocysts were mounted on glass slides and examined under an inverted Olympus IX-71 fluorescence microscope (Olympus). The experimental procedure was independently repeated four times.
The real-time reverse-transcription polymerase chain reaction (RT-PCR) protocol closely followed the previously described method [14]. Real-time RT-PCR utilized primer sets listed in Table 1 and a Step One Plus Real-time PCR System (Applied Biosystems, Warrington, UK) with a final reaction volume of 20 µL containing SYBR Green PCR Master Mix (Applied Biosystems). Thermal cycling involved denaturation at 95°C for 10 min, followed by 39 cycles at 95°C for 15 s and 54°C or 60°C for 60 s, with subsequent cooling to 12°C. Relative gene expression levels were analyzed using the 2−ΔΔCt method [15], normalized against the expression level of the housekeeping gene ACTB. The experiment was independently replicated three times.
The data obtained from all experiments underwent analysis using the general linear model procedure within the Statistical Analysis System (SAS User’s Guide, Statistical Analysis System, Cary, NC, USA). To determine significant differences, Tukey’s multiple range test was utilized. The presentation of values in this study follows the convention of mean ± SEM for independent experiments, with statistical significance indicated by symbols (p < 0.05).
RESULTS
The analysis of the primary components of POEE revealed the presence of collagen (280 mg/100 g), lutein (9 μg/100 g), coenzyme Q10 (2 mg/100 g), vitamin E-α (26 mg/100 g), and vitamin B9 (FA, 330 mg/100 g) (Table 2). Notably, FA emerged as the predominant component of POEE, as confirmed by the analysis. Additionally, DPPH analysis demonstrated the DPPH radical scavenging capability of POEE. The radical scavenging rates at 9,000 ppm were determined as 31.9%, 33.3%, 27.0%, and 71.6% for the 30E samples in the order of 0–2, 24, 48, and 50–60 h, respectively (Table 3). Significantly, the rate at 50–60 h was notably higher than at other time points (p < 0.05). Correspondingly, for the DW samples, the values were 36.5%, 53.5%, 39.5%, and 67.3%, respectively, in the same order, indicating relatively high the radical scavenging rates, akin to the 30E samples. Based on these observations, the IC50 value for POEE hatching was calculated as 5,830 mg/mL for 30E (Fig. 1A) and 6,143 mg/mL for DW (Fig. 1B). Further, the sample was subjected to a 10-fold dilution with the addition of DPPH reagent, revealing POEE concentrations of 600, 650, 700, and 750 when diluted 10-fold from the IC50 value.
Ingredient | Content (per 100 g) |
---|---|
Collagen | 280 mg |
Lutein | 9 μg |
Coenzyme Q10 | 2 mg |
Vitamin E-α | 26 mg |
Vitamin B9 (folic acid) | 330 mg |
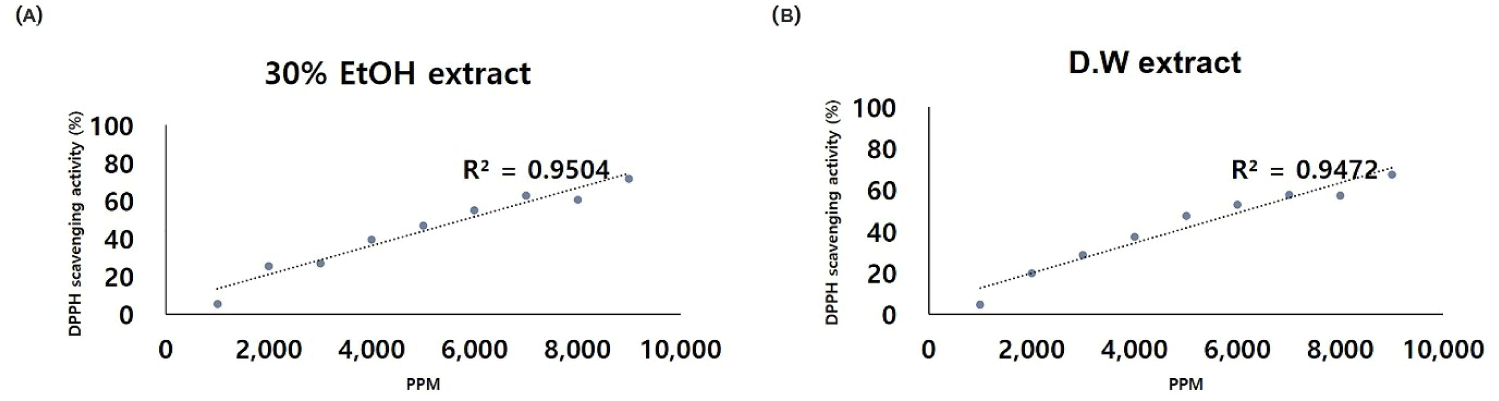
This investigation aimed to assess the impact of POEE treatment on porcine oocytes under conditions of oxidative stress induced by a peroxidative environment (200 μM H2O2) during IVM. The oocyte survival rate exhibited a significant increase in the 650 POEE treatment group compared to the control group (p < 0.05; Fig. 2A). Furthermore, the cleavage rate significantly improved in all treatment groups, except the control group (p < 0.05), with the highest rate (76%) observed in the 700 POEE group. Although the blastocyst rate did not show a significant increase compared to the control group, it was notably higher in the 700 POEE group (Figs. 2B and 2C). Consequently, the application of POEE demonstrated the restoration of both cleavage and blastocyst rates to levels comparable to those observed in the normal group.
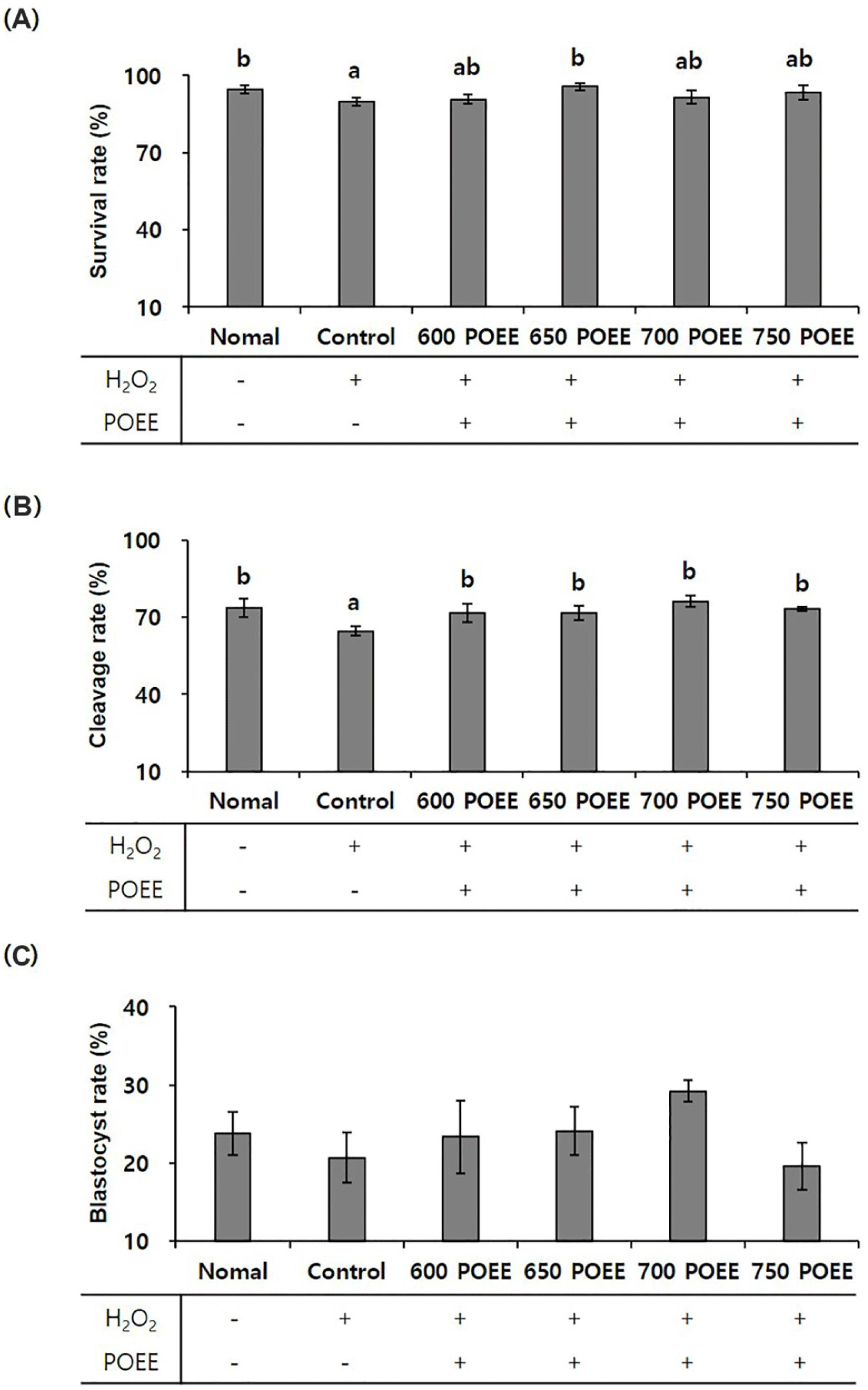
To validate the efficacy of FA, the primary component of POEE, we determined the optimal FA concentration. Groups treated with 0, 0.1, 0.2, 0.3, and 0.4 mM FA (0, 0.1, 0.2, 0.3, and 0.4 FA) in conjunction with POEE were established, and cleavage and blastocyst formation significantly increased at 0.3 FA (p < 0.05). Consequently, 0.3 FA was selected for subsequent experiments (Figs. 3A, 3B, and 3C).
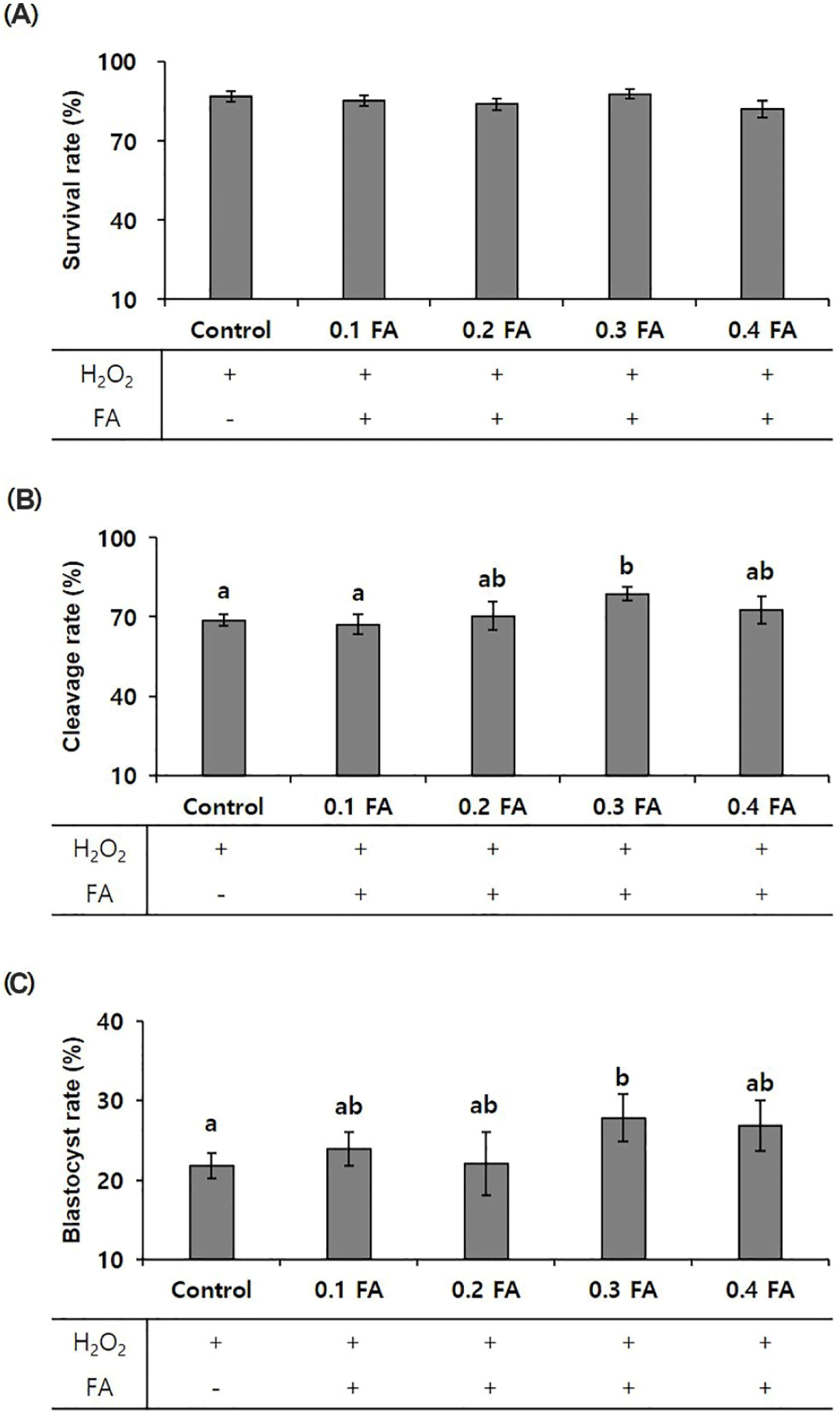
In this study, we conducted a comprehensive evaluation of the antioxidative effects of POEE and FA in a peroxidative environment, as illustrated in Fig. 4A. The fluorescence intensity of CMF2HC (measurement of glutathione) demonstrated a significant increase in the normal and 650 POEE groups compared to the control group (p < 0.05; normal = 57.0 ± 0.7 pixels/oocyte; control = 45.3 ± 4.1 pixels/oocyte; 650 POEE = 55.4 ± 1.0 pixels/oocyte; 0.3 FA = 53.3 ± 0.5 pixels/oocyte; Fig. 4B). Conversely, the fluorescence intensity of DCFHDA (measurement of ROS) exhibited a significant decrease in the normal and 0.3 FA groups compared to the control group (p < 0.05; normal group = 29.1 ± 0.4 pixels/oocyte; control group = 33.5 ± 1.4 pixels/oocyte; 650 POEE = 32.5 ± 0.9 pixels/oocyte; 0.3 FA = 29.9 ± 0.5 pixels/oocyte; Fig. 4C).
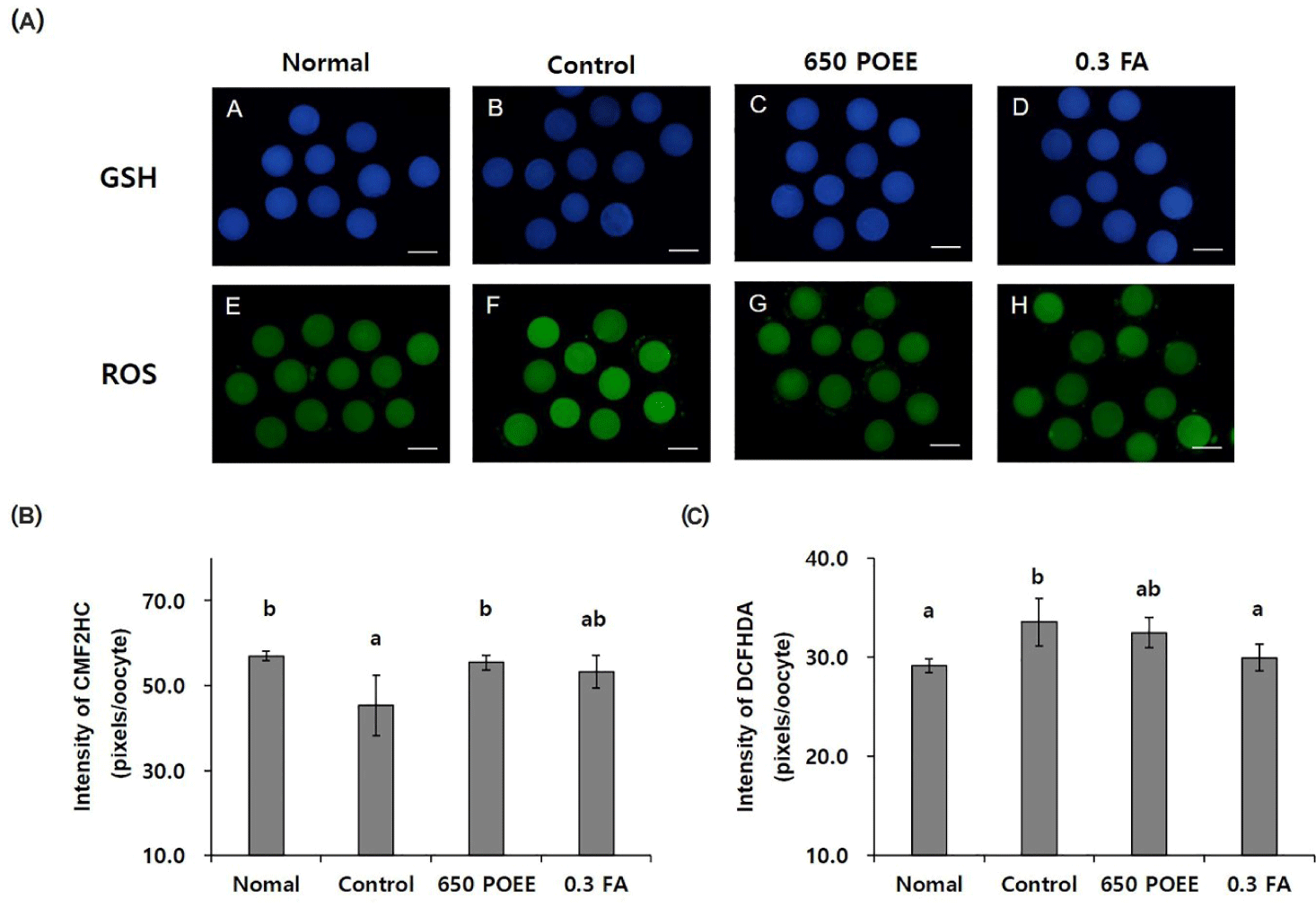
Furthermore, the impact of POEE on spindle morphology, a critical factor in nuclear maturation, was investigated. Spindles without abnormalities were classified as normal, and chromosomes not aligned in metaphase plates were considered abnormal [16]. The percentage of oocytes with normal meiotic spindles significantly increased in the 650 POEE and 0.3 FA groups compared to the control group (p < 0.05). Moreover, the proportions in the 650 POEE and 0.3 FA groups were similarly elevated compared to the normal group (normal group = 58.1 ± 1.0%; control group = 35.6 ± 4.4%; 650 POEE = 59.9 ± 1.5%; 0.3 FA = 59.4 ± 2.0%; Figs. 5A and 5B).
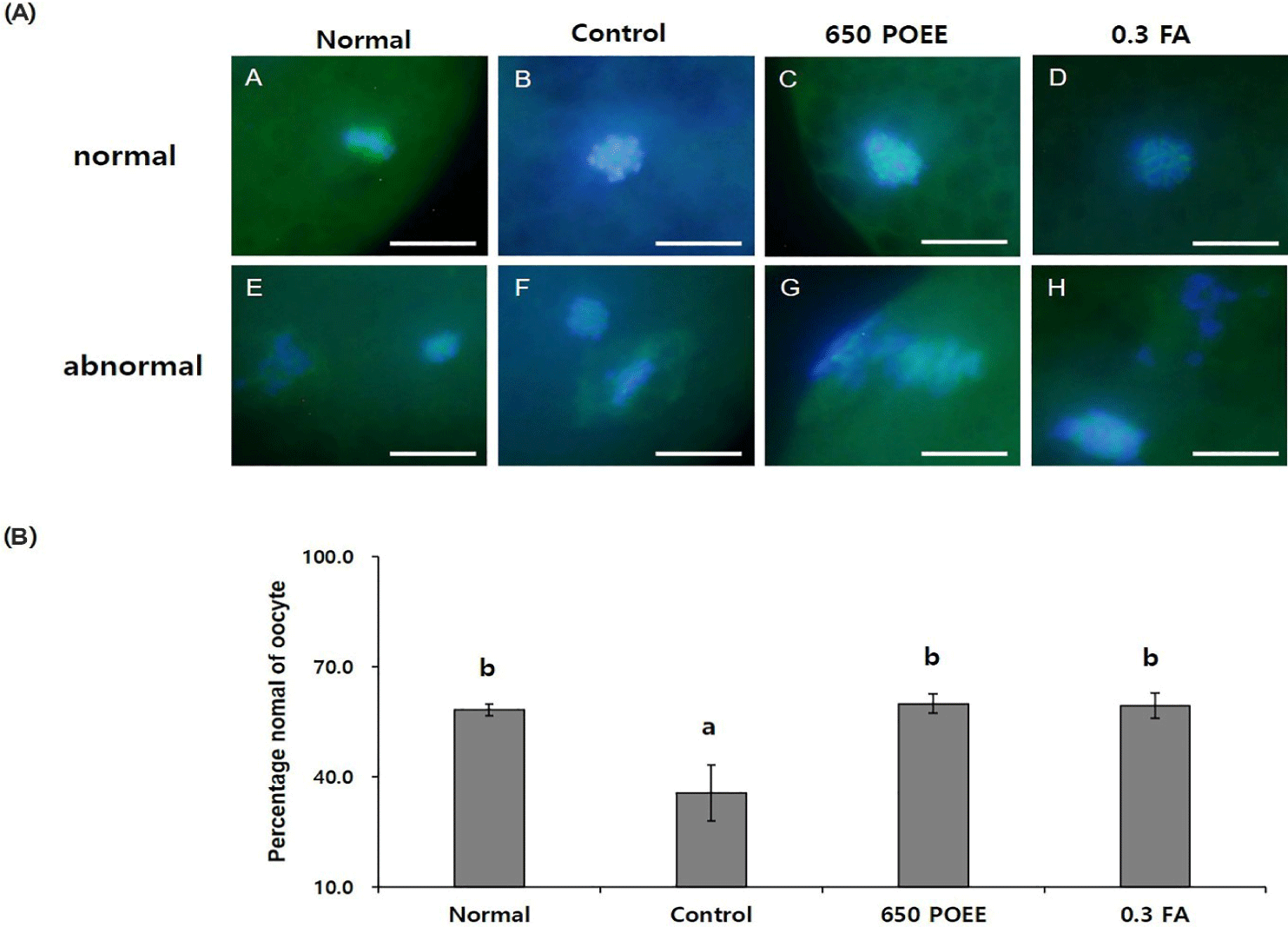
To elucidate the effects of POEE compared to FA during the IVM of porcine oocytes, we examined subsequent embryonic developmental parameters and the blastocyst quality derived from peroxidative oocytes (Fig. 6). The total cell counts per blastocyst significantly increased in the normal group and 650 POEE compared to the control group (p < 0.05; Fig. 6B). Genomic DNA fragmentation, assessed through a TUNEL assay to detect apoptotic cells in blastocysts, revealed a significantly lower proportion of apoptotic cells in the normal group and 650 POEE compared to the control group (p < 0.05; Fig. 6C). Furthermore, we measured the mRNA expression levels of development-related genes (POU5F1, SOX2, and NANOG) (Fig. 6D). The expression of POU5F1 and NANOG was significantly upregulated in the 650 POEE group compared to the control group (p < 0.05).
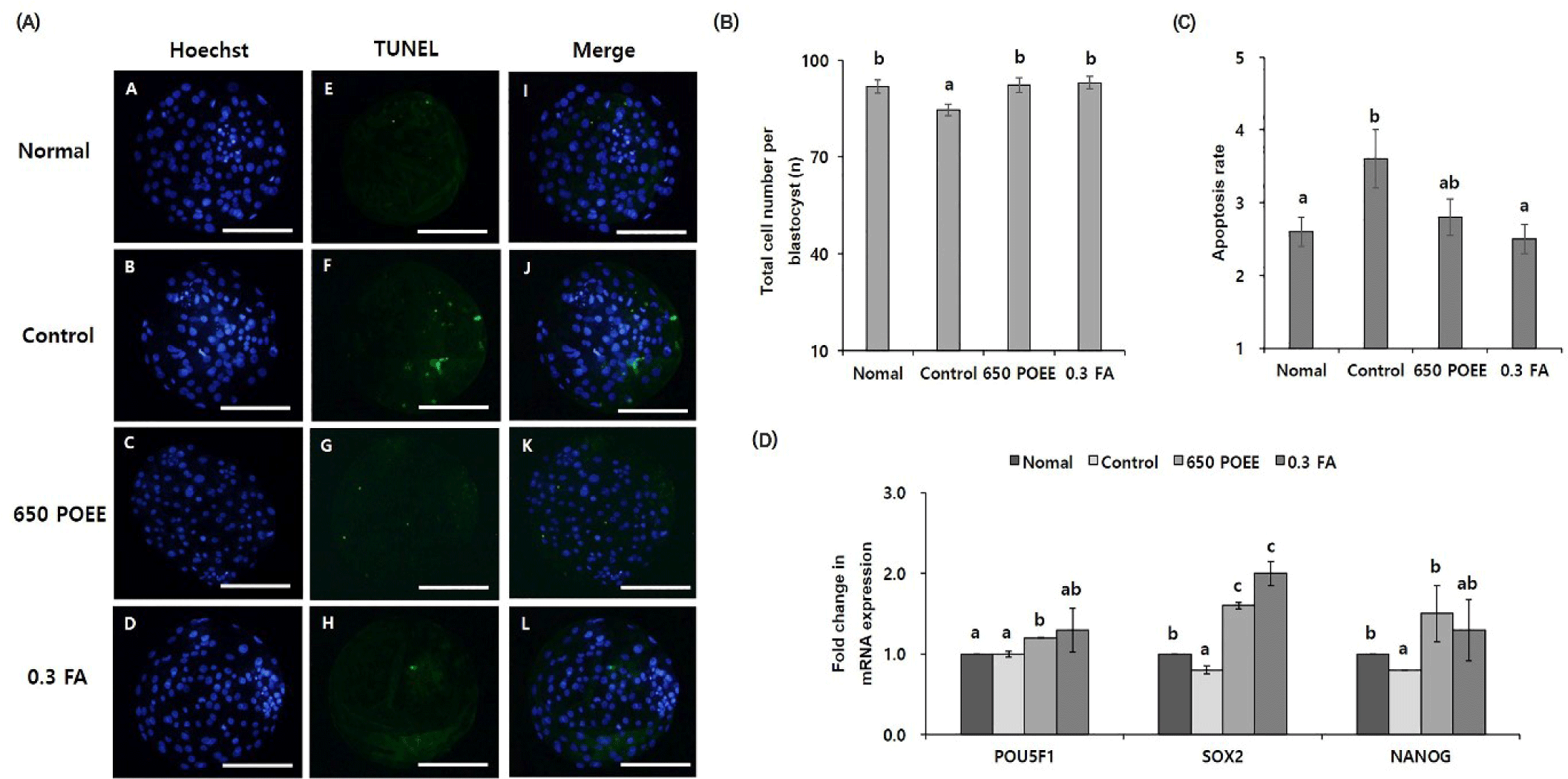
DISCUSSION
This study aimed to explore the influence of POEE on the IVM of porcine oocytes subjected to oxidative stress and its subsequent effect on the developmental capacity of embryos derived from these oocytes. Notably, POEE demonstrated an augmentation of GSH levels to a comparable extent, the prevention of chromosomal misalignment, heightened mRNA expression levels of developmentally related genes, and an enhanced improvement in blastocyst quality compared to FA. To affirm the antioxidant effect of POEE and its capability to scavenge free radicals that pose a threat to biologically significant macromolecules, we conducted a comprehensive analysis of its components. Free radicals, known for their ability to inflict damage on DNA, proteins, carbohydrates, and lipids in the nucleus and cell membrane, disrupt cellular homeostasis [17,18]. Employing the DPPH free radical scavenging activity assay, a well-established method for screening antioxidant activity, we determined the radical scavenging ability of POEE [19]. Previous studies have utilized DPPH to investigate various extracts, such as the radical scavenging and antioxidant activities of dried plum [20], local native plants [21], and distinct parts of Tabebuia pallida [22]. In particular, the DPPH radical scavenging ability of dried plum was reported as 79.78 ± 1.34%, with a calculated correlation coefficient (r² = 0.922) between total phenolic content (TPC) and DPPH [20]. Similarly, another study on Tabebuia pallida leaves (TPL) reported DPPH and hydroxyl radical scavenging activities of 91.05 ± 1.10% and 62.00 ± 0.57%, respectively [23]. For POEE, the observed DPPH radical scavenging activity was 71.6 ± 1.5%, a level akin to that of dried plum and falling within the range reported for TPL extract (91.05 ± 1.10% and 62.00 ± 0.57%). Moreover, the correlation coefficient between DPPH and POEE was calculated as r² = 0.95, surpassing the correlation observed for plum extract. These findings collectively suggest that POEE exhibits an antioxidant activity on par with that of TPL and plum extracts.
This investigation aimed to assess the impact of POEE, recognized for its antioxidant activity and substantial FA content (330 mg/100 g POEE), during IVM of peroxidized porcine oocytes. The selection of the minimum effective concentration with a significant difference from the control group was undertaken to evaluate the overall developmental rate during POEE treatment (650 POEE). Additionally, to validate the efficacy of FA, the principal constituent of POEE, various FA concentrations were tested, revealing a significantly increased overall developmental rate at 0.3 mM FA compared to the control group. FA, belonging to the vitamin B group [23], serves as a pivotal inhibitor of free radical formation and action, thereby mitigating oxidative stress, and holds critical biosynthetic functions [24]. Acknowledged for its antioxidant capabilities and affinity for biomolecules [25], FA assumes a secondary role in DNA synthesis and contributes to cell regeneration by fostering cell division [26]. Furthermore, functioning as a methyl group source, FA facilitates the methylation of homocysteine into methionine, playing a crucial role in the synthesis of pyrimidines and purines, as well as in cell growth and division processes [27]. The presence of FA in POEE may attenuate apoptosis in porcine oocytes, augment maturation rates, and enhance the maturation process and GSH synthesis during subsequent embryonic development. Consequently, FA-enriched POEE has the potential to shield oocytes from oxidative stress, thereby potentially elevating the embryonic development rate in oocytes exposed to a peroxidation environment.
To investigate the antioxidant effect of in vitro oocytes in a peroxidative environment, an analysis of GSH and ROS levels was conducted. Numerous studies have demonstrated alterations in the oxidant-antioxidant balance upon the addition of H2O2 to IVM medium [28–31]. Elevated ROS levels in vitro can result from external oxygen exposure or an insufficient antioxidant activity mechanism [32]. The observed mechanism linking GSH and ROS levels underscores the relationship between the antioxidant effect in oocytes and oxidative stress. Exposure to oxidative stress detrimentally impacts the growth potential and embryonic development of oocytes, disrupting microfiber and microtubule dynamics and leading to a reduced proportion of MII oocytes [33,34]. GSH plays a protective role against oxidative damage in oocytes [35]. The accumulation of ROS induces alterations in mitochondrial activity, diminishing the rate of cell division and resulting in structural and embryonic development arrest [36]. The protective effect of GSH against ROS is facilitated by its interaction with enzymes such as GSH peroxidase and GSH reductase [2]. Moreover, a critical function of GSH is to maintain the redox state within cells, thereby shielding them from the detrimental effects of oxidative damage [37]. Previous studies have demonstrated the protective effects of antioxidants such as dieckol, astaxanthin, and melatonin on oocytes exposed to oxidative stress [38–40]. Our investigations reveal that FA and POEE regulate the antioxidant mechanism in oocytes, suggesting their roles as antioxidants in oocyte physiology.
We substantiated the antioxidant effect of POEE on porcine oocytes. To elucidate the mechanistic connection between this antioxidant effect and oocyte nuclear maturation, we monitored the proportion of oocytes exhibiting normal spindles. The process of spindle assembly, integral to nuclear maturation in oocytes, involves the condensation of chromatin fibers into chromosomes during mitosis and meiosis [41]. Subsequently, spindle microtubules extend from the centrosome in an afferent manner, orchestrating chromosome alignment and contributing to the regulation of the cell cycle [42]. Microtubules and microfibers play crucial roles in meiosis, where the MI spindle translocates to the plasma membrane, facilitating the extrusion of the first polar body and orchestrating the rotation of the MII plate before activation [43]. Normal mitochondrial function is paramount for maintaining the integrity of the meiotic spindle and microtubule networks [44]. Oxidative stress, stemming from factors such as aging or peroxidation, can compromise oocyte function by inducing excessive ROS production, leading to diminished adenosine triphosphate (ATP) levels and subsequent mitochondrial dysfunction [45]. The opening of the permeability transition pore (PTP) under oxidative stress-induced conditions further exacerbates mitochondrial dysfunction, potentially resulting in spindle degradation [46]. Treatment with POEE ameliorated the proportion of oocytes with normal spindles through the aforementioned mechanisms. Additionally, treatment with FA demonstrated a similar improvement compared to the control group, prompting an exploration of the distinctions between the effects of FA and POEE. Our experiments revealed a significant increase in the proportion of oocytes with normal spindles in both the 650 POEE and 0.3 FA groups compared to the control group, with levels being reinstated to those observed in the normal group. Previous reports have indicated that antioxidants protect against premature aging in mouse oocytes [47]. Antioxidants like astaxanthin and resveratrol, known for their antioxidant activity, have been shown to inhibit aging in porcine oocytes and enhance the quality of senescent oocytes post-ovulation [48]. These antioxidant treatments suppressed abnormal spindles, resulting in a significant increase in the ratio of normal spindles. Consequently, our study suggests that POEE treatment modulates the mechanism of nuclear maturation.
We investigated the impact of POEE treatment on the quality of pre-implantation blastocysts and examined whether it regulates development-related mechanisms. Treatment with POEE during IVM simulated the effects observed with FA treatment, resulting in enhanced developmental ability and improved embryo quality. Several specific mechanisms contribute to the development and enhancement of blastocyst quality. Oocyte quality emerges as a pivotal factor influencing embryonic capacity [49], with increased cell numbers correlating with embryonic development [50]. Furthermore, apoptosis, indicative of cellular stress, is inversely associated with embryonic development, as an elevated proportion of apoptotic cells impedes this process [51,52]. Developmental processes entail the expression of blastocyst-related genes (POU5F1, NANOG, and SOX2), which undergo distinctive regulation in embryos generated through PA or in vitro fertilization compared to in vivo-derived embryos. This regulation is essential for the isolation and maintenance of extraembryonic tissues [53]. The NANOG protein, in particular, binds to 74% of first-generation genes during the early stages of junctional genome activation, while POU5F1 and SOX2 proteins are associated with 40% of early junctional genes [54]. Treatment with POEE has demonstrated the capacity to ameliorate developmental potential and enhance embryo quality under oxidative conditions through engagement with these molecular mechanisms.
CONCLUSION
In summary, our findings reveal that supplementation of IVM medium with POEE provides protection for oocytes against oxidative stress. Moreover, POEE facilitates both nuclear and cytoplasmic maturation, enhancing blastocyst formation and developmental competence, thereby fostering the generation of high-quality monomeric embryos. Considering these outcomes, the application of POEE in ART emerges as a promising strategy to augment overall success in reproductive interventions.