INTRODUCTION
Pollen-supplementary diets play a major role in honeybee health and honey production. Supply of artificial pollen diets to honeybee colonies is necessary for the development of young bee brood rearing, reproduction and maintenance of bee colonies, and honeybee production [1–3]. In cases of insufficient pollen supply, the immune system of bees and their strength weaken, which directly increases their mortality rate from attacks by various bee pests and pathogens [4–6]. Thus, most beekeepers feed honeybee colonies with pollen supplements such as defatted soybean, maize, and gram flour, especially when the natural pollen is not sufficient to maintain colony health and immunity in June-July [3,7,8]. Also, beekeepers supply artificially synthesized food known as pollen patties to increase food storage and nutrition in the winter season [9]. Therefore, several researchers have formulated and tested various artificial pollen diets to supply sufficient nutrients to maintain bee colonies [10–12].
Pollen patties, which contain bee-collected pollen, are mixed with different ingredients to meet the desired nutrient requirement [13]. Supplements contain bee-collected pollen mixed with other ingredients, such as soybean flour and honey, to form the desired patty consistency [14]. Therefore, numerous studies have evaluated the effects of supplying pollen patties and identifying new materials for improving honeybee performance and honey production [4,–12].
Curcumin, which is produced by Curcuma longa L., is a natural phenol that promotes therapeutic properties such as anti-inflammatory, anticarcinogenic, and antioxidant activities [15–17]. Also, curcumin has been shown to be a bifunctional antioxidant that scavenges reactive oxygen species (ROS) and triggers an antioxidant response to exert antioxidant activity both directly and indirectly [18,19]. However, curcumin possesses low absorption due to its impaired water solubility, unstable chemical structure, and rapid metabolism in the body [20,21]. To improve the bioavailability of curcumin, steviol glycosides have been used to increase the solubility by utilizing the solubilizing properties [22]. Steviol glycosides are substances extracted from stevia (Stevia rebaudiana Bertoni) leaves that have been reported to improve solubility by dissolving soluble substances [23,24]. Thus, the supplementation of pollen patties with a curcumin-steviol glycoside complex (CSG) could be an ideal strategy to increase immune systems and alleviate the adverse effects of bacteria and pathogens.
Therefore, the main objective of this study was to investigate the effects of pollen patty with supplementation of different concentrations of CSG on body weight, diet consumption, honey production, brood area measurement, and antioxidant gene expression.
MATERIALS AND METHODS
Twelve colonies of A. mellifera were conducted from July 10th to August 21st for 42 days at Chungbuk National University (36˚37’48" N, 12727’5” E) in Cheongju-si, Republic of Korea. The formulation of pollen patties is shown in Table 1. The CSG used in this experiment was obtained from a commercial company (BIOTEN, Jeongeup, Korea). A. mellifera were assigned to four dietary treatments with 3 replicates of equal size as follows: no supplementation of pollen patty (NC), supplementation of basal pollen patty (PC) supplementation of basal pollen diets + 0.04% of CSG [T1], supplementation of basal pollen diets + 0.08% of CSG (T2). The percentage of CSG was calculated based on the total weight of pollen patties. Each of the four groups consisted of 1 populated frame and 3 brood frames. Pollen patty diets were directly placed over the brood nests of bee colonies and covered with plastic sheets to prevent drying. They were freely and easily available to the A. mellifera colonies. The consumption of pollen patties was checked every day, and new pollen patties (300 g) were supplied every week.
Compositions of moisture content, crude protein, ether extract, crude ash, crude fiber, and nitrogen free extract (NFE) were analyzed according to the standard recommended by the Association of Official Analytical Chemists (AOAC) [25].
Moisture content was calculated by drying the sample in an oven at 100°C for 2 h. The dried sample was placed into desiccators, cooled down and then reweighed. This process was repeated until a constant weight was obtained. Crude protein was analyzed by the Dumas method (Rapid MAX N-Exceed, Elementar, Langenselbold, Germany) [26]. The ether extract was analyzed by using a Soxhlet extractor (EAM model, Misung Scientific, Seoul, Korea) [25]. Crude ash was analyzed according to the method of AOAC by using dry oven circulation (550°C) [25]. The percentage of crude fiber was determined according to the method of AOAC [25]. Calculating the NFE used the following formula: 100 – (Crude protein + ether extract + crude fiber + crude ash+ H2O). All the analyzed data were expressed as mean ± standard deviation.
A. mellifera were divided into three body parts to determine the effects of CSG. Total body weight, thorax weight, head weight, and abdomen weight were measured by dehydrating to a persistent temperature (60°C for a period of 48 h) [27].
The amount of pollen patty consumed was calculated by subtracting the weight of pollen patties and the weight of 1-day-old pollen patties after being placed in the colony (Patty consumption = beginning patty weight-ending patty weight). The weight of pollen patties was measured every day. The data were obtained by recording each formulated diet. The total consumption for each diet during the experimental period (42 days) was also calculated.
At the end of the experiment, the production of honey was measured in g by harvesting with an extracting machine (Manual honey harvester) to compare honey production for each colony.
Sealed worker brood area was calculated after 14, 28 and 42 days by using measuring a frame wire grid with divisions giving an area of one square inch each [28–30] and then converted in to cm2 by multiplying with 2.54. Sealed brood was used as a criterion for evaluating the development of colonies.
A. mellifera were collected at 42 days, and the head, wings, and legs were removed to obtain the thorax and abdomen. The RNA was extracted from the obtained thorax and abdomen using the total RNA extraction kit (iNtRON Biotechnology, Seongnam, Korea). The mRNA was converted to cDNA using high-capacity cDNA Reverse transcription kit (Applied Biosystems, Waltham, MA, USA). The mixed solution was heat treated at 25°C for 10 min, at 37°C for 2 h, and at 85°C for 5 min. Gene amplification was performed using the Fast qPCR 2 × SYBR Green Master Mix (Applied Biosystems). Gene amplification was performed for 40 cycles as followed cycle: 50°C for 2 min and 95°C for 10 min; 15 secs at 95°C; 1 min at 53°C; 15 secs at 95°C; 1 min at 53°C. The target genes were catalase, thioredoxin reductase 1 (Trxr1), superoxide dismutase 1 (SOD1), superoxide dismutase 2 (SOD2) and glyceraldehyde-3-phosphate dehydrogenase 2 (GAPDH). Primers used in the amplification are shown in Table 2 below. Normalization was performed using the reference gene GAPDH. Relative gene expression was analyzed using the 2−ΔΔCt method [31].
All data were statistically processed using the one-way ANOVA using JMP Pro 16 (JMP® Pro version 16.0.0, SAS Institute, Cary, NC, USA), using each pen as the experimental unit. Differences among all treatment means were determined using the Tukey multiple-range test. The level of significance was established at p < 0.05.
RESULTS
As shown in Table 3, thorax weight was significantly increased (p < 0.05) in the T2 diet (9.80 g) compared with the NC (8.90 g) and PC diet (9.00 g) at 42 days. There was no significant difference (p > 0.05) in head, abdomen, and total BW at 0, 14, 28, and 42 days.
As shown in Table 4, there was no significant difference (p > 0.05) in pollen patties consumption among the PC, T1, and T2 diet.
Items (g) | PC1) | T1 | T2 | SEM | p-value |
---|---|---|---|---|---|
Daily consumption2) | 28.27 | 27.61 | 28.03 | 1.493 | 0.952 |
As shown in Fig. 1, the T1 and T2 diets showed significantly higher (p < 0.05) honey production than the PC and NC diets. Also, the PC diet showed significantly higher (p < 0.05) honey production than the NC diet.
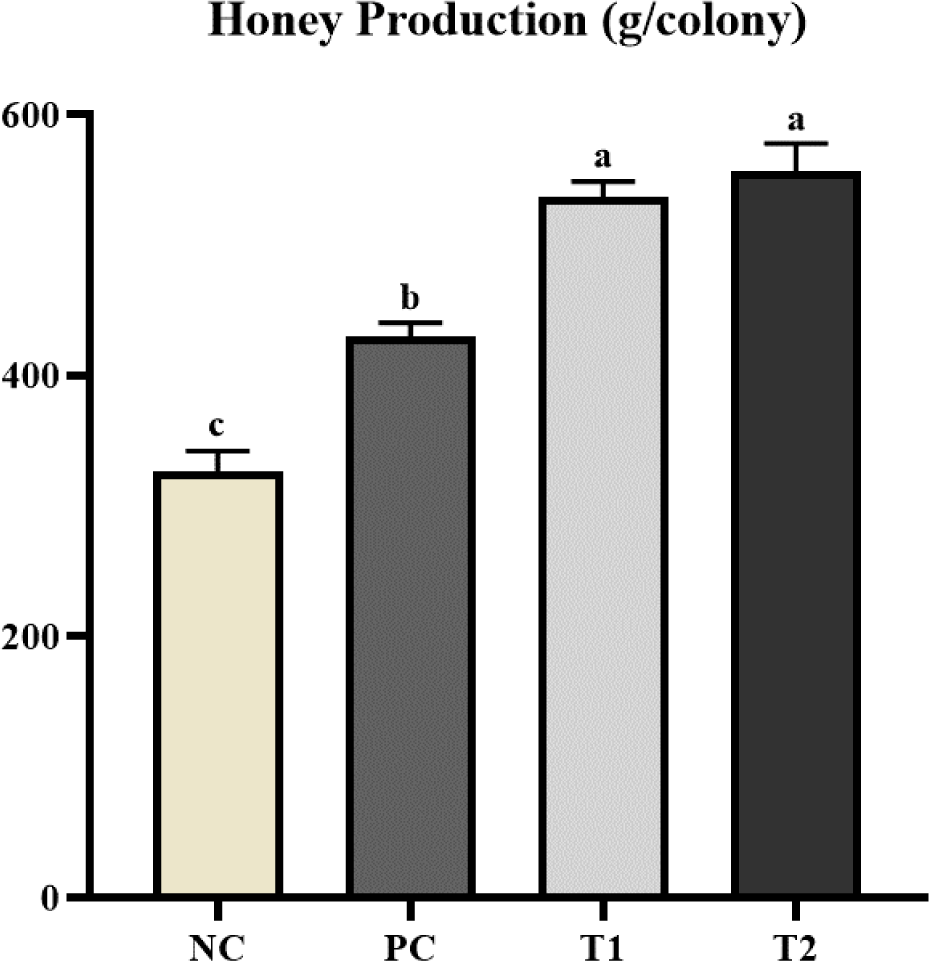
As shown in Fig. 2, the T2 diet showed significantly higher (p < 0.05) brood area than the PC and NC diets at 28 and 42 days. Also, the PC and T1 diets showed significantly higher (p < 0.05) brood areas than the NC diet. There was no significant difference (p > 0.05) at 0 and 14 days.
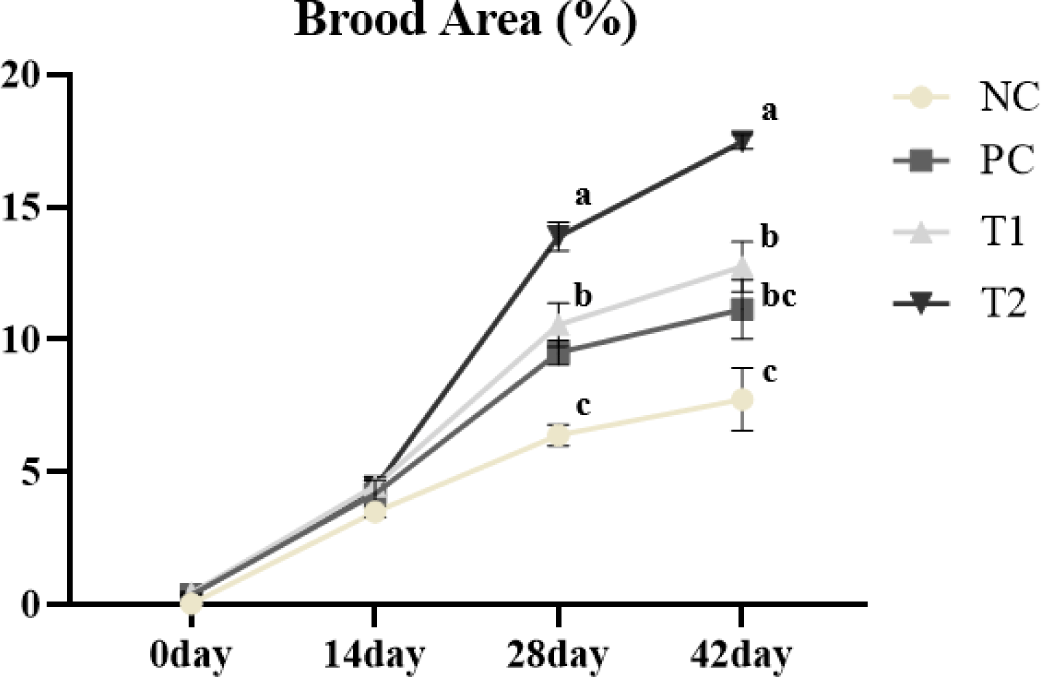
As shown in Fig. 3, the T1 and T2 diets showed significantly higher (p < 0.05) Catalase and SOD1 gene expression than the PC and NC diets. The expression level of the Trxr1 gene was significantly higher (p < 0.05) in the T1 diet, and decreased in the order of the PC, T2, and NC diets. The expression level of the SOD2 gene was significantly higher (p < 0.05) in the T1 diet than in other diets and was lower in the NC diet.
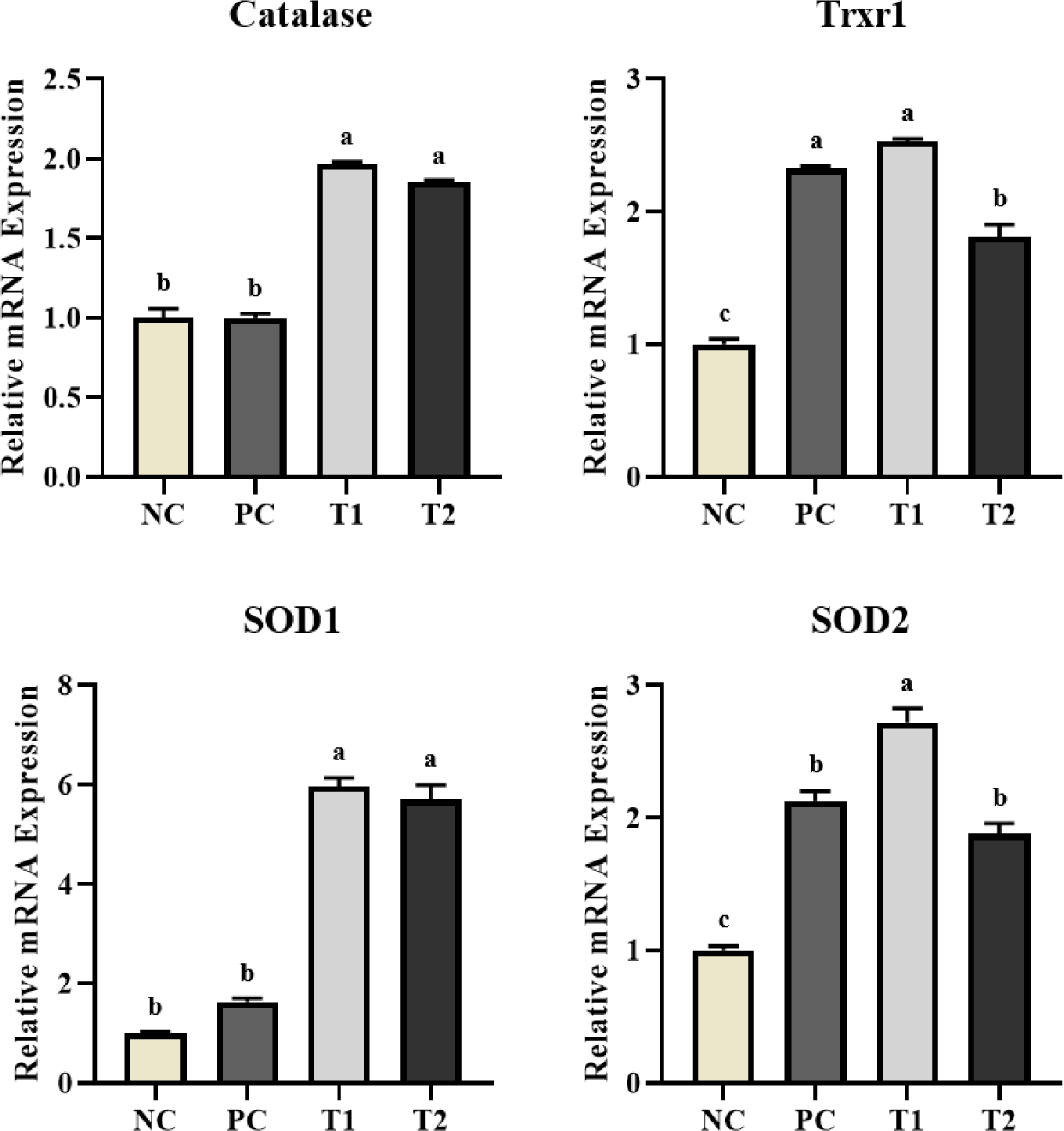
DISCUSSION
A higher thorax weight in A. mellifera has been suggested to induce stronger and more agile flight, which improves their foraging activities [32]. Numerous studies have demonstrated the positive correlation between thorax weight and flight performance [33,34]. Therefore, higher thorax weight is considered an index of higher flight performance in A. mellifera [35,36].
During the flight, A. mellifera significantly increases its metabolic rate, which, in turn, increases its flight foraging activity times in collecting pollen [37,38]. Carbohydrate catabolism plays a major role in producing an adequate metabolic rate to improve flight in A. mellifera [39]. Also, Teulier et al. [40] have demonstrated that A. mellifera utilizes carbohydrates as a metabolic fuel for flight. Moreover, Brodschneider et al. [35] have reported that when insufficient nutrition is provided, delayed maturation of the enzymes of carbohydrate metabolism induces impaired flight performance, which decreases the thorax weight in A. mellifera.
In this study, we observed a higher thorax weight and amount of NFE in supplementation of CSG. According to Ghosh and Jung [9], the NFE represents the soluble carbohydrates in pollen patties. This result indicates that supplementation of CSG increases the content of the carbohydrate in the pollen patty. Also, a previous study has reported that supplementation of curcumin could increase the digestibility of carbohydrates by improving intestinal enzymes [41]. Therefore, increased thorax weight might be reasonable due to the increase of carbohydrate and enhanced utilization of carbohydrates by supplementing CSG in this study.
In contrast, no significant differences were observed in total body, head, and abdomen weight in this study. Previous studies demonstrated that supplementation of dietary protein increases the size of the hypopharyngeal gland, which results in a higher head weight in A. mellifera [42,43]. Also, Ullah et al. [44] reported that the highest body weight was observed when sufficient protein (30 g of soybean flour) was available. However, there were no sufficient differences in the crude protein content of pollen patties (0.06%–0.08%) between the cases of supplementation or non-supplementation of CSG in this study. Although the recommended amount of protein in pollen patty has not been identified, it demonstrates that the amount of protein in pollen patty may be insufficient to increase the weight of honeybees. Therefore, a higher amount of protein in the pollen patty might be required to increase the body weight of A. mellifera.
Dietary curcumin consumption implicates the prevention of oxidative stress, which results in enhanced longevity in A. mellifera [45]. In addition, Avni et al. [46] have demonstrated that greater consumption of supplements (such as protein and carbohydrates) led to enhanced brood production and tended toward higher honey yields as well. Regarding diet consumption, several studies have indicated that diets with additional nutrition supplements were consumed at higher rates relative to diets without the additional nutrient supplementation [1,10,47]. Also, Anvi et al. [46] have reported that pollen patties consisting only of carbohydrates were more consumed than those consisting of protein and lipid sources. Similarly, Scheiner et al. [48] have demonstrated that high sucrose concentrations increase the phagostimulating effects to induce the consumption of pollen patties. Therefore, we guessed that diet consumption might be increased due to the supplementation of pollen patty with CSG. However, no significant differences were noted in the total diet consumption between the supplementation of pollen patties with CSG and those without it. These results indicate that the NFE (differences among the PC, T1, and the T2 diets: 0.69%–1.50%) was insufficient to trigger the phagostimulating effects of increasing the consumption of pollen patties containing the CSG.
The amount of honey production is correlated with pollen collection and consumption in honeybees [10]. Insufficient nutrient supplementation causes impaired strength and health in A. mellifera, which accounts for the decreased foraging activity in terms of collecting pollen into their colonies [1,2,49]. The present results confirmed that the supplementation of pollen patties with CSG yielded higher honey production compared to that without the supplementation. As shown in Table 1, pollen patties with the CSG showed relatively higher NFE levels (0.69%–1.50%) to the non-supplementation of CSG. Carbohydrates are considered a major source of fuel for foraging flights, which refers to the activity of collecting pollen in the honey colonies [50]. Thus, carbohydrate supplements could provide sufficient nutrients to the colonies and increase honey production by improving their strength and health. Numerous studies have reported that the supplementation of pollen patties enriched with carbohydrates increased honey production when compared to the case of non-supplementation of pollen patties to the colonies [4,51–53]. Therefore, increased honey production might be reasonable due to the supplementation of pollen patty with CSG in A. mellifera.
In this study, the supplementation of pollen patties with CSG resulted in improved brood area. The brood area at day 42 was approximately 10% higher in the T2 supplemented with pollen patty than in NC without pollen patty supplementation. In addition, the T2 supplemented with the CSG showed a significantly higher area than the PC. Supplementing A. mellifera with additives possessing antioxidant properties has been shown to improve their health and functionality [54–56]. Curcumin, when used in feeding, can reduce oxidative stress through its antioxidant function [18,19]. Tawfik et al. [57] have reported that reducing oxidative stress improves the colony strength and health of honeybees. The size of the brood area is highly correlated with the number of colonies and populations as it can predict the number of new bee larvae born [58]. As a result, improving the brood area could improve the colony strength and, thus, increase the honey production [40]. Based on the above results, we suggest that supplementing CSG when feeding pollen supplements to bees can improve their brood area.
In this study, the expression of genes related to antioxidants, Catalase, and SOD1 was significantly higher in the T1 and T2 supplemented with the CSG. In addition, the treatment group fed with pollen patties showed significantly higher values than the NC treatment for Trxr1 and SOD2. It shows a similar trend to the results of Alaux’s study [59] analyzing gene expression after feeding pollen patties to A. mellifera. Feeding pollen patty appears to increase the expression of antioxidant genes and adding 4% of the CSG appears to further improve it. Bees can fly up to 7km a day to collect pollen or nectar in nature [60,61]. Flight requires a lot of energy, which increases metabolism. Additionally, it triggers the production and accumulation of ROS in the body, causing faster aging [62,63]. ROS causes significant oxidative stress in A. mellifera [64–6]. A decrease in the health and lifespan of bees can lead to weakened colony strength and decreased productivity [67]. Rueppell et al. [67] have reported that delaying nurse-to-forager can increase lifespan by up to 8-fold. In other words, the lifespan of A. mellifera improves when ROS production decreases due to the absence of flight for pollen or nectar collection. Catalase, SOD1, SOD2, and Trxr1 measured in this study are considered powerful enzymes that can remove ROS [68,69]. Feeding pollen patty and supplementing with CSG is expected to reduce oxidative stress by increasing the expression of antioxidant enzymes and improving the health of bees.
CONCLUSION
In this study, supplementation of pollen patties with CSG showed improved thorax weight, honey production, brood area, and antioxidant gene expression. This result indicates that supplementing pollen patties with a CSG enhanced the performance of A. mellifera. Therefore, CSG as supplement to pollen patty might be the ideal strategy to improve A. mellifera performances.