INTRODUCTION
Despite increasing meat consumption, the use of slaughter by-products is limited owing to the increase in the volume of imported by-products, the lack of improvement in consumer awareness, and a lack of product development [1,2]. The efficient use of by-products from the meat production process is very important not only in reducing waste, but also in improving the efficiency of utilization of livestock products. Although the utilization rate is not high owing to a low efficiency of extraction, there are many bioactive substances in meat by-products, such as heparin, collagen, chenodeoxycholic acid (CDCA), and ursodeoxycholic acid (UDCA), conjugated linoleic acid (CLA) [3–5].
Among the many bioactive substances in meat by-products, heparin is a compound carbohydrate that is an anticoagulant (blood thinner) to prevent blood clotting [6]. It is a polysaccharide of glycosaminoglycans with a strong negative charge and is found on the cell surface and in the extracellular matrix [6]. It was also confirmed in the 1950s that the activity of anti-thrombin was accelerated by heparin [7,8]. Heparin is widely distributed in various tissues such as the lung, liver, blood, and intestinal tissues of higher animals, especially in the mast cells. Heparin, which is used in laboratories and clinics, is generally extracted from the bovine heart or the small intestinal mucosa of swine, and is made into a calcium or sodium salt through enzymatic and chemical treatment [6].
Heparin is especially used for preventing of blood clotting during ventrotomy and organ transplantation, deep vein thrombosis, or heart thrombosis that develops as a result of blood circulatory deterioration. In addition, it has many biological functions, such as anti-inflammatory, antithrombotic, antihyperlipidemic, and anti-arteriosclerotic properties, as well as anticoagulant function that inhibits thrombin activity [9,10]. Previous studies have shown that heparin inhibits the production of angiotensin by renin and inhibits aldosterone secretion, and ultimately has an antihypertensive effect [11–13]. Infection and thrombosis are the most common complications of central venous catheters. Various gram-positive and negative bacteria cause catheter-related sepsis through central venous catheter [14,15]. The antibacterial activity of heparin was confirmed in various bacteria related to the above [14–18].
However, it is very expensive and time consuming to extract and purify heparin from pork by-products. Moreover, there are no studies comparing the amount of heparin in each pig by-product. Therefore, the purpose of this study was to develop a low- cost and highly efficient heparin extraction method in seven major pig by-products and analyze the anti-angiotensin I-converting enzyme (ACE) inhibitory activity in the extracted heparin and the antimicrobial activity against pathogenic microorganisms.
MATERIALS AND METHODS
Six pig by-products (liver, lungs, heart, stomach, small intestine, and large intestine) for the extraction of heparin were obtained from the Dodram slaughter house (Anseong, Korea) and Woo-gyeung Livestock (Hwaseong, Korea). The pig by-products were transported to the laboratory and cleaned with tap water and then the bronchi connection to the lungs and esophageal connection to the stomach were severed, and the non-discharged digest inside and outside the organs was washed. After washing, the by-products were ground using a chopper, then subdivided, and stored frozen (Fig. 1).
Methods for the extraction of heparin from pig by-products were described previously by Charles and Scott [19–21], Freeman et al. [22], Volpi [23], and Sarwar [24] with modifications in order to simplify the extraction. Folch I solution (chloroform-methanol mixture, 2:1 by volume) was added to the by-product samples and homogenized using a homogenizer (SHG-15D-Set, SciLab-brand, Seoul, Korea) for autolysis. After shaking every 30 min for 3 h, the crude fat was extracted and the solution and by-product tissues were separated. The Folch I solution used in the extraction of crude fat was separated by adding 0.88% NaCl solution and left overnight. The chloroform layer (the lower layer) in which fat was precipitated was dried to obtain crude fat. The by-product tissue was rinsed once with 250 mL of acetone, completely dried at low temperature, and stored frozen. Crude fat and protein obtained in the process of extracting heparin can be used to extract other high-value-added materials present in pig by-products (Fig. 2).
The method for extracting heparin from dried by-products after degreasing proceeded on the basis of the amount of dry by-product powder. To 100 g of dried by-products, 1,460 mL of 0.5N NaOH and 174 mL of saturated ammonium sulfate solution were added and the mixture was extracted in a water bath at 60°C for 30 min. Using gauze, discard the solids and collect the solution. Then, 7N sulfuric acid was added to the solution for titration to obtain a pH of 2, which formed a precipitate. This solution was centrifuged (Combi 514R, Hanil Scientific, gimpo, Korea) at 2,691 × g for raw min to remove the precipitate. The supernatant was extracted with 200 mL of 95% ethyl alcohol at 25°C for 20 h. The solution was centrifuged at 2,697×g for 20 min to remove the supernatant, and 150 mL of alkaline water (pH 10) was added to the precipitate. For each experiment, 0.5% of alkaline-AK was added to the by-product amount in the above mixture, and trypsin and papain were added at 0.25% of the by-product amount. After addition of 300 μL xylene, the mixture was left at 60°C in a water bath (37°C for trypsin) for 12 h. One hundred milliliters of 95% ethyl alcohol was added to the solution that had undergone proteolysis, and this was left for 20 h. The resulting precipitate was collected by centrifuging at 2.691×g for 30 min, and dissolved in 50 mL of alkaline water (pH 10). After addition of 25 mL of acetone, the mixture was stirred sufficiently, and the supernatant was removed by centrifugation at 2.691×g for 30 min. Then, 25 mL of 95% ethyl alcohol was added to the precipitate and incorporated thoroughly by stirring, and the precipitate was dried to obtain the heparin (Fig. 3).
Heparin sodium salt from the porcine intestinal mucosa (192 USP unit/mg, Sigma-Aldrich, St. Louis, MO, USA) was used as a heparin standard reagent for HPLC analysis. Quantification of extracted heparin from pig by-products was performed according to the method of Arumugam and Shanmugam [25], with slight modifications. Standard solutions were prepared by dissolving the standard heparin in distilled water (DW) at concentrations of 0.625–10 mg/mL. For the extracted heparin, HPLC analysis was carried out at a concentration of 2 mg/mL (Table 1).
Heparin sodium salt from porcine intestinal mucosa (192 USP unit/mg, Sigma-Aldrich) was used as a standard heparin reagent for heparin unit analysis and was analyzed using COATEST Heparin (Chromogenix, West Chester, OH, USA). A COATEST Heparin kit (Chromogenix) was used to measure the units of extracted heparin according to the manufacturer’s instructions. Standard solutions were prepared by dissolving the standard heparin in DW at concentrations of 0.2 IU/mL (1 μg/9.6 mL), and the extracted heparin was analyzed at a concentration of 0.2 μg/mL (Fig. 4).
ACE inhibitory activity was measured according to the method of Cushman and Cheung [26], with slight modifications. ACE solution was prepared by adding rabbit lung acetone powder (Sigma-Aldrich) at a concentration of 50 mg/mL to 0.01 M potassium phosphate buffer (pH 7.0) containing 0.5 M sodium chloride, stirring for 18 h, and centrifuging at 10,280×g for 60 min to obtain the supernatant. Hippuryl-His-Leu (HHL) solution was prepared by mixing 0.05 M sodium tetraborate and 0.05 M boric acid to a pH of 8.3 and then adding hippuryl-1-histidyl-1-leucine to this 0.05 M sodium borate buffer (pH 8.3). Then, 50 μL of HHL was added to standard heparin or 50 μL of extracted heparin, and the mixture was reacted at 37°C for 10 min. ACE (50 μL) was added to this and reacted at 37°C for 30–60 min. After that, 250 μL of 1N HCl and 250 μL of ethyl acetate were added and vortexed for 1 min. After completion of the reaction, the mixture was centrifuged at 1,977×g for 10 min. The 200 μL of hippuric acid in the supernatant was added to a new tube and completely dried in a drying oven (WOF-50, Daihan scientific, Wonju, Korea). The dried sample was dissolved in 1 mL of water just before measurement and placed in a cuvette. The dried sample was measured using a UV/VIS spectrophotometer (Cary 300 UV VIS, Agilent Technologies, Santa Clara, CA, USA) at a wavelength of 228 nm. To measure the antihypertensive activity of the sample, the control reaction was carried out by adding DW instead of the sample. After adding the substrate to the blank, the reacted value was measured by adding 0.05 M sodium borate instead of ACE. The absorbance value was substituted into the following equation to evaluate the antihypertensive activity:
where C is the absorbance value of the control, B is the absorbance value of the blank, and S is the absorbance value of standard heparin or extracted heparin.
In order to measure the antimicrobial activity of heparin, four kinds of bacteria, Listeria monocytogenes ATCC 7644, Pseudomonas aeruginosa KCTC 1750T (= ATCC 10145), Escherichia coli ATCC 25922, and Staphylococcus aureus ATCC 33591 were used. The viable count of microorganisms was determined by counting the number of colonies formed after incubation at 37°C for 24 h. Brain heart infusion broth and agar (BHIB/BHIA, BD DifcoTM, Franklin Lakes, NJ, USA) was used as culture media for culturing the bacteria. The bacteria cultured in BHIB at 37°C for 12 h was inoculated BHIA at 37°C for 24 h to measure the number of viable microorganisms. Based on the number of viable microorganisms, the bacteria cultured for 12 h were diluted to 1 × 107 CFU/mL. Then 0.625–10 mg/mL standard heparin was diluted 9:1 in the medium (medium: heparin). The cultured bacteria were divided into 96 cell culture plates (SPL, Pocheon, Korea) for 0, 2, 4, 6, 8, 12, and 24 h and analyzed using a microplate reader (ELISA [enzyme-linked immunosorbent assay device], Spectramax 190, Molecular Devices, San Jose, CA, USA), and the relative antibacterial activity was calculated. For the extracted heparin, concentrations of 5–20 mg/mL were prepared and the turbidity of the culture was measured.
Statistical analysis of the experiment was conducted by one-way ANOVA using the SPSS 22.0 program (IBM, Armonk, NY, USA). Statistical significance was assessed using the multi-range test of Student-Newman-Keuls’ and significance level of all data was evaluated based on p < 0.05.
RESULTS AND DISCUSSION
The amount of defatted pig by-product powder was significantly different among the organs. The liver, lungs, and heart had an average of about 19.5% of defatted-dried organ, and the stomach, small intestine, and large intestine, which are relatively hydrated, had 10.7% of defatted-dried organs (Fig. 5). The amount of defatted pig by-product powder was 420 g in liver, 162 g in lung, 83 g in heart, 105 g in stomach, 161 g in small intestine, and 40 g in the large intestine (Fig. 5) from 500 g of the pig by-product. This accounts for about 16% of the total 6 kg of pig by-product that are left after slaughter. The defatted-dried by-products are advantageous in delaying the decay of the by-products, thereby increasing the shelf life of the by-products, and reducing the cost of storage by reducing the volume of the sample.
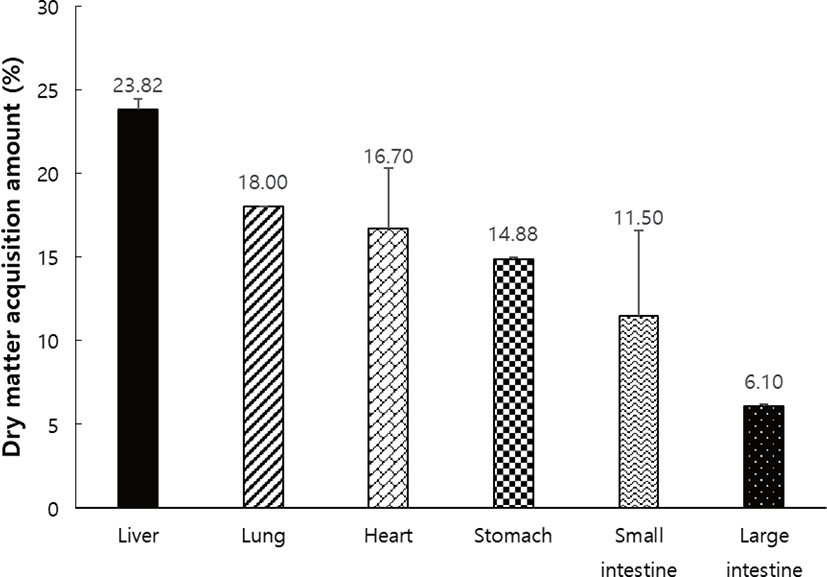
The extraction rate of heparin from each by-product was based on the weight of pig by-products before manufacturing the defatted pig by-product powder. Heparin was extracted at the highest rate in the liver and heart and was relatively low in the lung (Fig. 6, Table 2). These results indicate that the amount of heparin extracted from pig livers was similar to or greater than the results from a previous study [20]. The calculated amount of heparin extracted from total pig by-products was about 1,718 mg extracted by trypsin, about 1,697 mg extracted by papain, and about 1,905 mg extracted by alkaline-AK enzyme (Fig. 6, Table 2). In the conventional heparin extraction methods, proteolytic enzymes such as trypsin are used to remove proteins [19,20,22]. However, this study attempted to extract heparin using alkaline-AK, an industrial enzyme which is inexpensive, and a high titer enzyme, such as papain. Alkaline-AK is a proteolytic enzyme obtained by fermenting soybean meal by-products, which is about 1.3 million times cheaper than trypsin and 2,000 times cheaper than papain. The extraction of heparin after the acquisition of crude fat using Folch I reagent seems to reduce the final heparin extraction amount, but it generates additional benefits from the high value substances obtained in the crude fat.
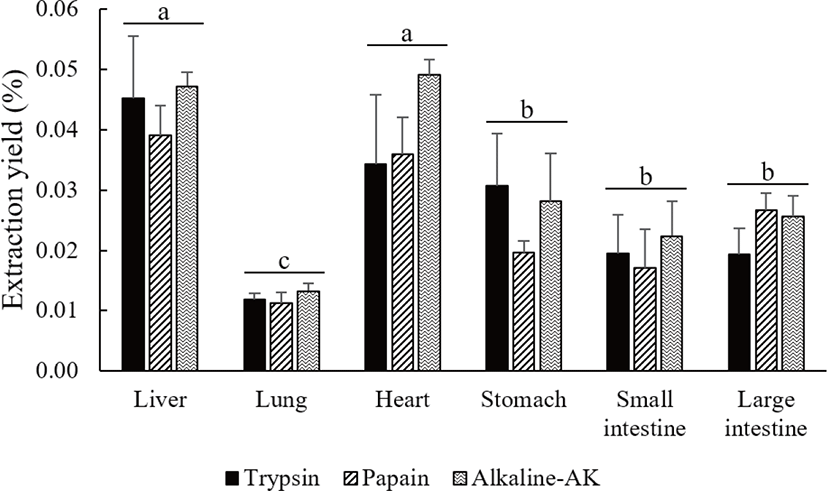
Glycosaminoglycan-based materials such as heparin can be identified using various chromatographic methods such as HPLC [25,27–29], UPLC [30], and liquid chromatography–mass spectrometry (LC/MS) [31,32]. Heparin is a strongly negatively charged substance [25,27–29]. When using reverse phase chromatography, heparin is first separated from the column by binding to a polar mobile phase. In this study, the small intestines contained the largest amount of heparin in 1 g of extracted heparin, and a relatively small amount of heparin was identified in the heart and stomach. This indicates that a high purity heparin is extracted in the small intestine and a low purity heparin is extracted in the heart and stomach. The statistical analysis confirmed that there was a significant difference in the amount of pure heparin by enzyme in each by-product. As a result, high purity heparin can be obtained using trypsin is higher than the others in the liver, lungs, heart, and small intestine. In the large intestine, papain is higher than the others. In the stomach, heparin of similar purity was obtained by the three enzymes. The average amount of heparin extracted by enzymes was confirmed in the order of trypsin is greater than or equal to papain, alkaline-AK (Figs. 7A and 7B).
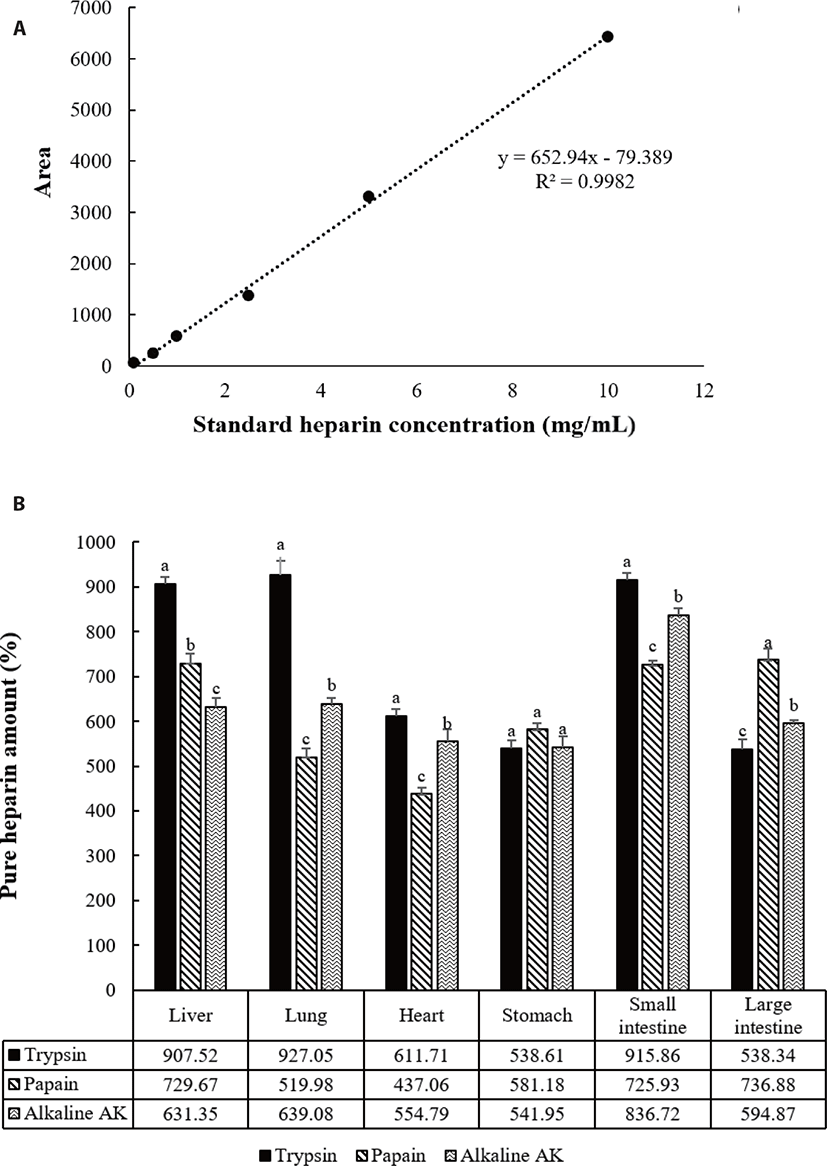
COATEST heparin assay quantifies the units of heparin. Heparin is analyzed in the form of a complex with anti-thrombin present in the sample. The units/mg of extracted heparin were similar in almost all pig by-products, but heparin extracted from the stomach was found to contain the highest amount of heparin at an average of 2,670 units/mg. The liver showed significant differences in units extracted by enzymes, in the order of alkaline-AK and papain greater than trypsin. In the case of the other by-products, there was a difference in the amount of extracted enzymes but there was no significant difference because the standard deviation was too large (Figs. 8A and 8B). However, the average amount of enzyme in the total by-products was in the order of papain and alkaline-AK greater than trypsin. It is assumed that the difference in the amounts of heparin in the same sample may be due to the proteolytic action of trypsin (serine peptidase) and papain (cysteine peptidase) [33].
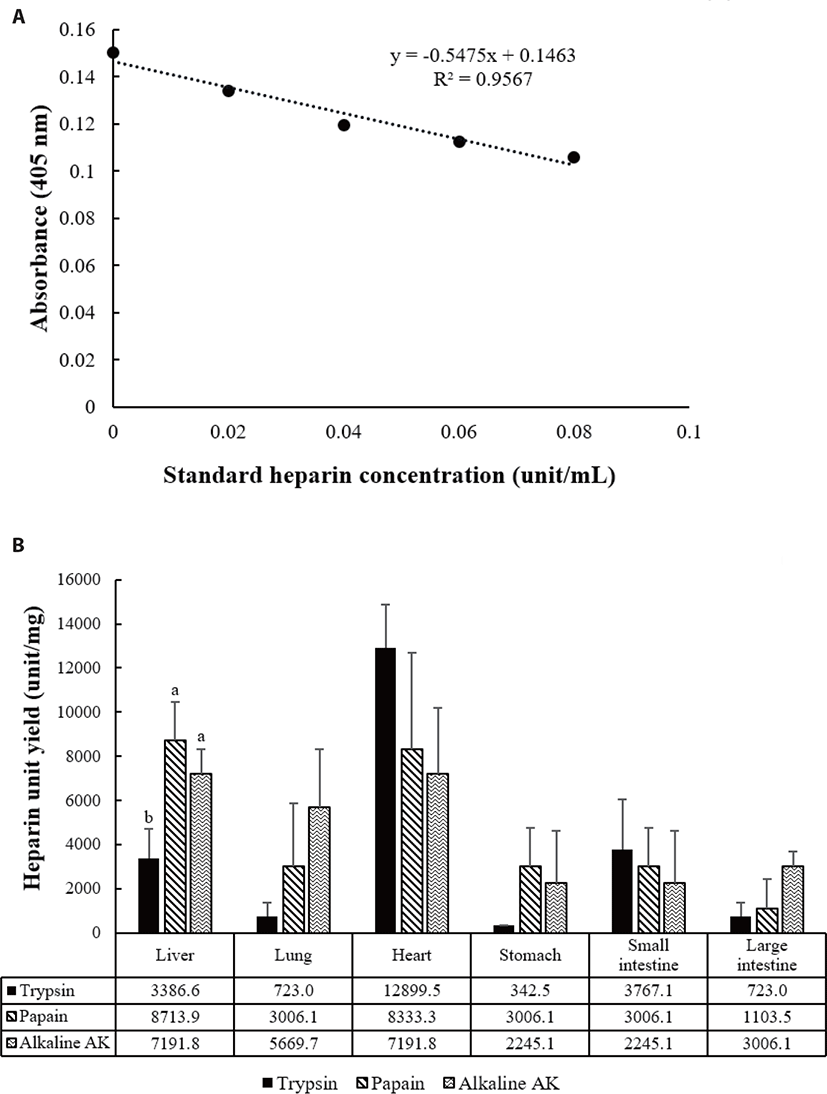
Studies on the antihypertensive activity of heparin have been conducted since the 20th century. Heparin inhibits the production of angiotensin by renin [12], and suppresses aldosterone secretion [11,13], and therefore is antihypertensive. Based on the previous studies, an experiment was conducted to confirm whether heparin extracted through an ACE inhibitory activity assay has antihypertensive activity that inhibits the production of angiotensin II (Fig. 9). Heparin extracted with papain had the highest antihypertensive activity (72.5%) and extracted heparin showed higher antihypertensive activity in alkaline-AK and trypsin (Fig. 10). Based on the results of the COATEST heparin assay, it can be inferred that the treatment containing the highest amount of heparin showed higher ACE inhibitory activity.
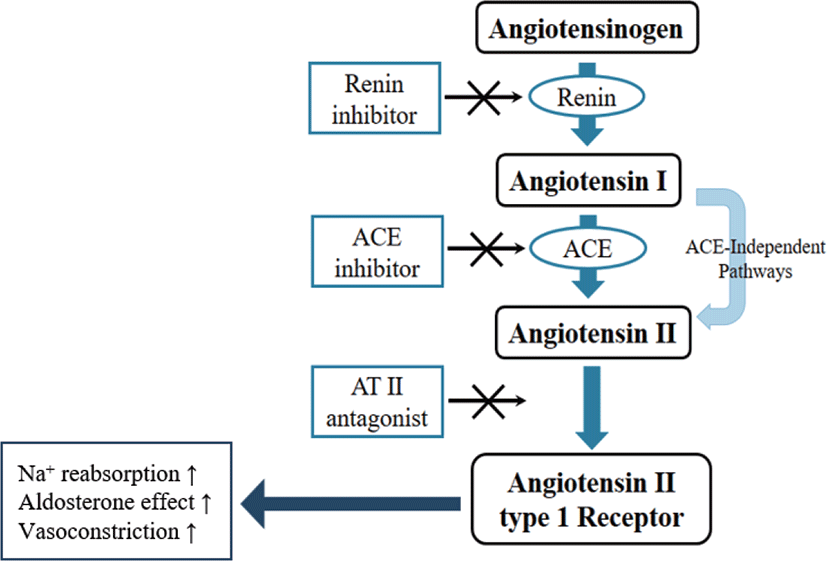
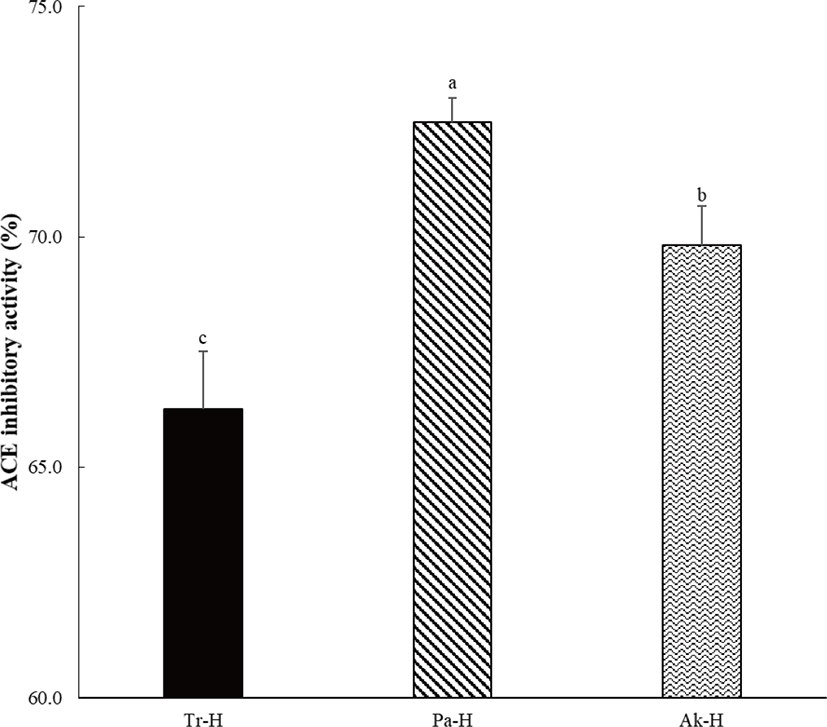
In the case of S. aureus, 2 mg/mL heparin was added to a medium containing an initial bacterial count of 1.21 × 103 CFU/mL. Heparin extracted by papain and alkaline-AK were shown to have an antimicrobial activity at low concentration (Fig. 11). However, in the case of L. monocytogens, E. coli, and P. aeruginosa, all the treatments in the samples cultured for 12 h did not grow compared to the control, but an antibacterial effect of heparin was not found (Fig. 11). As a result, the antimicrobial activity of the extracted heparin could not be confirmed and the regularity of the antimicrobial activity treatment by concentration could not be confirmed. These results suggest that there is no antibacterial activity of heparin against P. aeruginosa, or that the antimicrobial activity was not exhibited by using an excessive concentration of bacteria (Fig. 11).
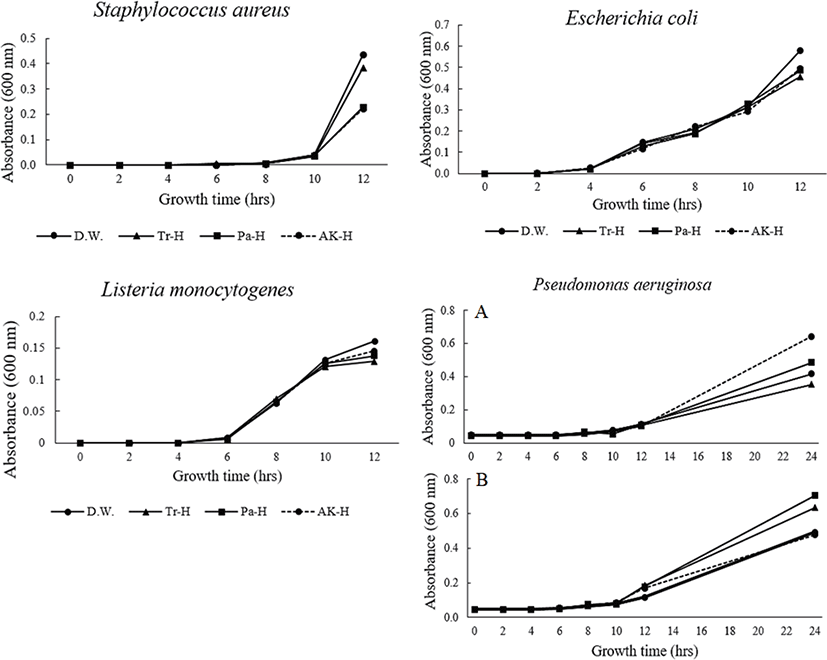
Heparin can be extracted from pig by-products, and is used in the medical fields for anticoagulant purposes. Heparin, which has been commercialized in the past, was limited to extractions from the pig small intestine, and heparin in other pig by-products was not utilized. Various studies have been conducted to reduce the disposal cost of the discarded pig by-products and further industrialize them [1]. The current study carried out experiments on the extraction, quantification, and validation of heparin. In this study, heparin was extracted from not only the small intestine (as is usually done), but also from six other major pig by-products. Using various types of proteolytic enzymes (papain and alkaline-AK), it was confirmed that heparin extraction could be similar to or greater than that of conventional enzyme extractions (trypsin), but using inexpensive and high titer enzymes. The above findings confirm that heparin can be extracted by a faster and simpler extraction method than the existing extraction method, and can verify the amount of heparin contained in the pig by-product. Heparin was purified immediately after extraction and subjected to quantitative analysis to confirm the amount. As a result of measuring the purity of extracted heparin by HPLC, extracted heparin from each enzyme showed similar purity. Among them, heparin extracted from the liver and small intestine were shown to have the highest purity. The COATEST heparin assay, which measures the units of heparin, indicated the highest extractions of heparin from the liver and heart. Based on the total amount of liver, it was confirmed that Pa-H (heparin extracted using papain) and AK-H (heparin extracted using alkaline-AK) contained more units than Tr-H (heparin extracted using trypsin).
ACE inhibitory activity and antimicrobial activity were measured to verify the efficacy of the extracted heparin. In the ACE inhibitory activity experiment, the activities were confirmed in the order of Pa-H, Ak-H, and Tr-H. This was similar to the units of extracted heparin analyzed by the COATEST heparin assay. Based on these results, it could be assumed that high heparin content confers ACE inhibitory activity. The antimicrobial activity of heparin (according to heparin units) was confirmed in S. aureus. No distinct antimicrobial activity was found against L. monocytogenes, E. coli, and P. aeruginosa, which may require further investigation.
-
The use of crude fat and protein generated during heparin extraction shortens the extraction time, and enzyme differentiation improved the efficiency of heparin extraction from pig by-products.
-
Data were presented to indicate the amount of heparin per pig by-product. The average amount of extracted heparin per kilogram of pig by-product was 439 mg from the liver, 127 mg from the lung, 398 mg from the heart, 261 mg from the stomach, 197 mg from the small intestine, and 239 mg from the large intestine.
-
Various enzymes were used to extract heparin, and the amount of extracted heparin was similar. Based on the major pig by-products (from one pig), trypsin, papain, and alkaline-AK enzymes extracted 1,718 mg, 1,697 mg, and 1,905 mg heparin, respectively.
-
Heparin showed antihypertensive activity and antimicrobial activity against S. aureus, and confirmed the possibility as a material to prevent or suppress disease.
For industrialization and mass production of heparin, it is important to establish a low-cost extraction process that can secure national competitiveness. Therefore, in this study, a method to reduce the production cost of heparin and generate additional profits was developed by simplifying the process and using inexpensive alkaline-AK (1.3 million times cheaper than trypsin, and 2,000 times cheaper than papain). Alkaline-AK enzyme extraction can obtain similar amounts of heparin compared to other enzymes and reduce the cost of the extraction process. Extracting heparin after crude fat is removed can reduce the use of enzymes and generate additional profits. Heparin’s ACE inhibitory activity and antimicrobial activity, which have not been fully studied, were confirmed, confirming the potential of heparin as a drug. Therefore, further investigation should be directed here in the future.