INTRODUCTION
Hanwoo beef is uniquely palatable due to its high intramuscular fat (IMF) content. It has less subcutaneous fat and higher marbling scores than other beef cattle breeds such as Australian Angus [1]. Beef IMF, in general, contains saturated fatty acids (SFAs) which is mainly are palmitic (C16:0) and stearic (C18:0) acids and typically account for less than 50% of the total lipid composition [2–4]. These SFAs comprise the main source of health concerns and associations with cardiovascular disease (CVD) [5]. However, aside from the SFAs, beef IMF also has higher overall concentrations of monounsaturated fatty acids (MUFAs) mainly the oleic acid (OA, C18:1 n-9) and polyunsaturated fatty acids (PUFAs) [1]. Oleic acid is a regulator of immune function and health. Substituting saturated fats with OA in the diet reduces the risk of CVD by reducing blood lipids, mainly cholesterol. Increasing high-density lipoprotein cholesterol (HDL-C) or the commonly known good cholesterol in the blood [6] and reducing the circulating bad cholesterol low-density lipoprotein cholesterol (LDL-C) concentrations [7–10] have been associated with reducing CVD risks. These documented findings highlight the importance of OA intake on human health concerning blood lipid profiles. In our previous study, we found that the oleic acid in olive oil can reduce the risk for CVD as evidenced by a loss of body weight, reduced triglyceride (TG), and increased PUFA such as α-linolenic acid (ALA), eicosapentaenoic (EPA), docosahexaenoic (DHA), and arachidonic (AA) acids in the blood lipid profiles of experimental rats [11]. Aside from the blood lipid profiles, understanding the lipogenic enzyme activities in the adipose tissue of rats would contribute to the effort to understand the health-related effects of oleic acid. Increased adipose tissue is linked to metabolic and hemodynamic processes in the production of adipokines, which are responsible for causing insulin resistance and atherosclerosis [12]. Decreased in activities of lipogenesis-related enzymes indicates a decrease in adipose tissue ultimately decreasing the risks for CVD. Thus, determination of the activities of enzymes related to lipogeneses such as fatty acid synthase (FAS), malic enzyme (ME), and glucose-6-phosphate dehydrogenase (G6PDH) would be a vital step in understanding the effects of dietary oleic acid on health-related parameters. In this study, we aimed to analyze the blood lipid profiles, as well as the FA profile, and lipogenic enzyme activities in adipose tissues of rats fed Angus beef fat (ABF) and Hanwoo beef fat (HBF) to determine the effects of oleic acid contained in these beef fats in alleviating its health impact.
MATERIALS AND METHODS
The experiments conducted in this study were carried out using the Animal Laboratory Ethics number CBNUVA-573-13-01. After 2 weeks of acclimation in the animal room, sixty (60) male Sprague-Dawley rats (249 ± 3.04 g) were randomly divided into three treatment groups consisting of twenty rats (n = 20) each group. The treatment includes the CON diet containing 7% coconut oil as control, the ABF diet containing 7% Angus beef fat, and the HBF diet containing 7% Hanwoo beef fat. The coconut oil was used as a control in this experiment as it is the most commonly used regular cooking oil and is viewed as the most deleterious cooking oil that could increase cardiovascular risks [13]. The beef fats used were IMF carefully taken from the Australian Angus and Hanwoo beef ribeye. Briefly, the beef meats were ground using the meat grinder with a fine plate (3-mm holes) and were heated in a non-grease pan for 5 minutes to melt and extract the fat from the meat. The collected beef fats in liquid state were then mixed with the other ingredients of the diet shown in Table 1 and prepared by extrusion and formulated based on the American Institute of Nutrition AIN-76A diet [14] with a slight modification: sugar content was reduced from 50% to 48%, and corn oil was replaced with coconut oil and beef fat. Coconut oil, ABF, and HBF were first analyzed using gas chromatography to determine their fatty acid profile as shown in Table 2. Each of the three modified diets contained 4,002 kJ/kg of total energy and the diets were vacuum-packed and stored at 4°C to minimize rancidity and oxidative damage. The nutrient composition of the diets was analyzed as follows: crude protein calculated as N × 6.25, crude fat, and ash based on the AOAC method [15]. The total carbohydrate, crude “by difference”, was calculated by using the formula: total carbohydrate, % = 100 – (crude protein + ash + crude fat). Energy value (estimated, kJ/kg diet) = [4 × crude protein, %] + [4 × ash, %] + [9 × crude fat, %].
4) Mineral mix used at 35 g/kg diet containing calcium phosphate dibasic (500 g/kg), sodium chloride (74 g/kg), potassium citrate H2O (220 g/kg), potassium sulfate (52 g/kg), magnesium oxide (24 g/kg), manganous carbonate (3.5 g/kg), ferric citrate U.S.P (6 g/kg), zinc carbonate (1.6 g/kg), cupric carbonate (0.3 g/kg), potassium iodate (0.01 g/kg), sodium selenite (0.01 g/kg), chromium potassium sulfate 12H2O (0.55 g/kg), and sucrose (118.03).
5) Vitamin mix used at 10 g/kg diet containing thiamine HCl (0.6 g/kg), riboflavin (0.6 g/kg), pyridoxine HCl (0.7 g/kg), niacin (3 g/kg), calcium pantothenate (1.6 g/kg), folic acid (0.2 g/kg), biotin (0.02 g/kg), vitamin B12 0.1% (1 g/kg), vitamin A palmitate 500,000 IU/g (0.8 g/kg), vitamin D3 400,000 IU/g (0.25 g/kg), vitamin E acetate 500 IU/g (10 g/kg), menadione sodium bisulfite (0.08 g/kg), and sucrose (981.15 g/kg).
The rats were housed in groups of two per cage at room temperature (22°C) under a 12-h light-dark cycle, 40%–60% relative humidity, and water ad libitum. The initial body weight of each rat was recorded prior to the conduct of the experiment. The body weight and feed intake (FI) of each rat were taken every week during the entire experimental period. FI was determined by weighing the food in each cage dispenser including spilled feed within the cage. After four and eight weeks, 10 rats per group were fasted overnight, anesthetized with ethyl ether, and sacrificed. Blood samples were collected from the abdominal aorta into Vacutainer® tubes, and the plasma was separated by centrifugation at 1,075×g for 20 min for biochemical analysis. The blood lipid profile was evaluated by measuring the glutamic-oxaloacetic transaminase (GOT), and glutamic-pyruvic transaminase (GPT), HDL-C cholesterol, total cholesterol (TC), and level of TGs in plasma using an Advia 2120 chemical analyzer (Siemens Healthcare GmbH, Erlangen, Germany). The liver, kidneys, heart, lungs, spleen, and subcutaneous fat were excised, weighed, and immediately snap-frozen in liquid nitrogen.
Adipose tissues (0.3 g) were placed in Folch solution (chloroform: methanol 2:1 v/v; 10 mL) and homogenized for 1 min at 16,028×g (CH/PT3100 Polytron, Kinematica AG, Malters, Switzerland) with the internal standard (5 mg) C13:0. Thereafter, the homogenate and 0.74% KCl (5 mL) were Vortex mixed for 1 min, and the mixture was separated by centrifugation at 1,912×g for 30 min at 4°C. The lower chloroform layer containing lipids was evaporated at 70°C under N2 gas using a heating block. Lipids were then saponified with 0.5 M KOH in methanol (1 mL) at 90°C for 10 min and methylated with 1 mL of 14% BF3 in methanol at 90°C for 30 min. Fatty acid methyl esters (FAME) were mixed with hexane and NaCl, then separated by centrifugation. The top layer was evaporated to calculate the amount of FAME, then diluted with HPLC-grade hexane. Samples were analyzed using an HP6890 gas chromatograph with an SP®-2560 fused silica capillary column (Supelco, Bellefonte, PA, USA) measuring 100 m × 0.25 mm × 0.2 μL film thickness. The inlet temperature was 270°C and the flame ionization detector (FID) was held at 300°C. The oven temperature was held at 150°C for 10 min, then increased at a rate of 2°C per min to 220°C and maintained for 10 min. The flow rate of the column was 10 cm/min, and the split/splitless ratio was 50:1. Fatty acids were quantified using a standard mixture (Nu-Chek Prep, Tokyo, Japan), and C13:0 fatty acid (Sigma Aldrich, St. Louis, MO, USA) as the internal standard.
Fresh liver and epididymal adipose tissues (1 g) were homogenized in 10 mM potassium phosphate pH 7.6, containing 150 mM KCI, 1 mM MgCI2, 10 mM N-acetylcysteine and 0.5 mM/L dithiothreitol. After centrifugation at 100,000×g for 40 min, supernatants were frozen and stored at −80°C. G6PDH (E.C.1.1.1.49), ME, (E.C. 1.1.1.40), and FAS (E.C. 2.3.1.85) were determined from the rates of the glucose-6-phosphate-dependent reduction of nicotinamide adenine dinucleotide phosphate (NADP+) [16], the malate-dependent reduction of NADP+ [17], and malonyl-CoA dependent NADPH oxidation [18], respectively. We measured NADPH as absorbance at 340 nm. All enzyme assays proceeded at 37°C. Soluble protein in supernatants was colorimetrically determined using bovine serum albumin as the standard [19]. The enzyme activities G6PDH and ME are expressed as nkat NADPH produced per mg protein, and FAS activity as pkat NADPH consumed per mg protein. The specific activity of acetyl CoA carboxylase (CBX) is expressed as pkat bicarbonate incorporated per mg protein.
Data were analyzed using the SAS Software General Linear Method [20] as a completely randomized design experiment. The fatty acid composition was analyzed across the treatment diets using the one-way analysis of variance (ANOVA) method. The growth performance, biochemical parameters, fatty acid profiles, and lipogenic enzyme activities were analyzed as a 3 × 2 factorial design representing the three treatment diets (CON, HBF, ABF) and two feeding periods (4 and 8 weeks). For each variable measured, values were averaged and subjected to least square analysis of variance ANOVA by using the GLM procedure in SAS to determine the main effects of treatment diets, feeding periods, and their interaction effects. Significance was declared at p < 0.05. Data were further subjected to Duncan’s multiple range test (DMRT) if significant differences were detected.
RESULTS
The fatty acid composition of the diets was shown in Table 2. Myristic, palmitic, and stearic were the only SFA significantly affected by the different diets. The CON treatment diet showed higher myristic acid and lower palmitic and stearic acid than ABF and HBF treatment diets (p < 0.001). The diets showed significant differences only on MUFAs palmitoleic and oleic acid. Palmitoleic acid was higher in the HBF group than ABF (p < 0.05) and was not detected in the CON group. Oleic acid, on the other hand, was highest in HBF and lowest in the CON group with the ABF group showing slightly lower oleic acid than HBF (p < 0.05).
The effect of CON, ABF, and HBF on growth performance and organ weights were shown in Table 3. Significant effects by fat (p < 0.05) and by period (p < 0.001) were observed on the final body weight (BW), average daily gain (ADG), weight gain, daily FI, and feed efficiency (ADG/FI) of the experimental rats, but no significant interaction was observed on all growth performance parameters except on ADG/FI (p < 0.032). Specifically, the HBF group significantly increased the final BW, ADG, weight gain, daily FI, and ADG/FI of the experimental rats compared to HBF and CON groups at both feeding periods (4 and 8 weeks). As expected, the feeding period of 8 weeks showed a greater increase in final BW, weight gain and FI than the 4 weeks feeding period (p < 0.001). However, ADG and ADG/FI was observed higher in the first 4 weeks of feeding. It was also observed that there was a nearly significant interaction effect between the fat and feeding period on the weight gain of the rats (p < 0.075) meaning that HBF tended to increase the weight gains of the rats with increasing feeding period. In terms of the weight of the internal organs, a significant effect by fat was not observed, but the feeding period for 8 weeks significantly increased the internal organ weights of the rats (p < 0.001).
The different diets did not significantly affect the GOT and GPT levels in the blood plasma of rats but significantly affected the HDL-C, TC, and TG of rats (p < 0.05) as shown in Table 4. The ABF and HBF groups significantly increased HDL-C of rats both at 4 and 8 weeks of feeding as compared to CON (p < 0.001). The ABF group also significantly increased the TC level of rats compared to HBF and CON at 4 weeks of feeding (p < 0.05). At 8 weeks of feeding, the ABF and CON groups significantly increased the TC level of rats (p < 0.05). The CON group also markedly increased the TG levels of rats both at 4 and 8 weeks of feeding compared to ABF and HBF groups (p < 0.05).
The adipose tissues of rats fed CON, ABF, and HBF contained 28 fatty acids, of which 12 major fatty acids were shown in this study (Table 5). The different diets significantly affected the SFAs such as lauric, myristic, and palmitic acid, the MUFAs myristoleic and oleic acid, and PUFA linolenic acid in the adipose tissue of rats. The CON group showed higher lauric, myristic, and myristoleic acid than ABF and HBF in the adipose tissue both at 4 and 8 weeks of feeding (p < 0.001). Palmitic acid in the adipose tissue was also significantly increased by CON followed by ABF and HBF both at 4th and 8th week feeding periods but at a lower significance level (p < 0.05). The ABF and HBF groups showed higher oleic and linolenic acid than CON in the adipose tissue both at 4 and 8 weeks of feeding (p < 0.05).
The activities of ME, and FAS, both lipogenic-related enzymes, in the liver and adipose tissue of rats fed with CON, ABF, and HBF diets at 4 weeks of feeding period were shown in Figs. 1A and B. The different diets did not significantly affect the ME activity both in the liver and adipose tissue of rats. The FAS activity in the liver of rats was also not significantly affected but a decrease in the FAS activity in the adipose tissue of rats fed HBF and ABF was observed (p < 0.05). On the other hand, activities of ME, FAS, and in addition the G6PDH, also a lipogenic-related enzyme, at 8 weeks of feeding period were shown in Figs 2A, B, and C. The different diets did not significantly affect the activities of ME, FAS, and G6PDH in the liver but significantly affected the activities of the enzymes in the adipose tissue. The HBF and ABF groups significantly reduced the activity of FAS in the adipose tissue of rats fed at 8 weeks feeding period (p < 0.05), while HBF and CON markedly increased the activity of G6PDH (p < 0.05).
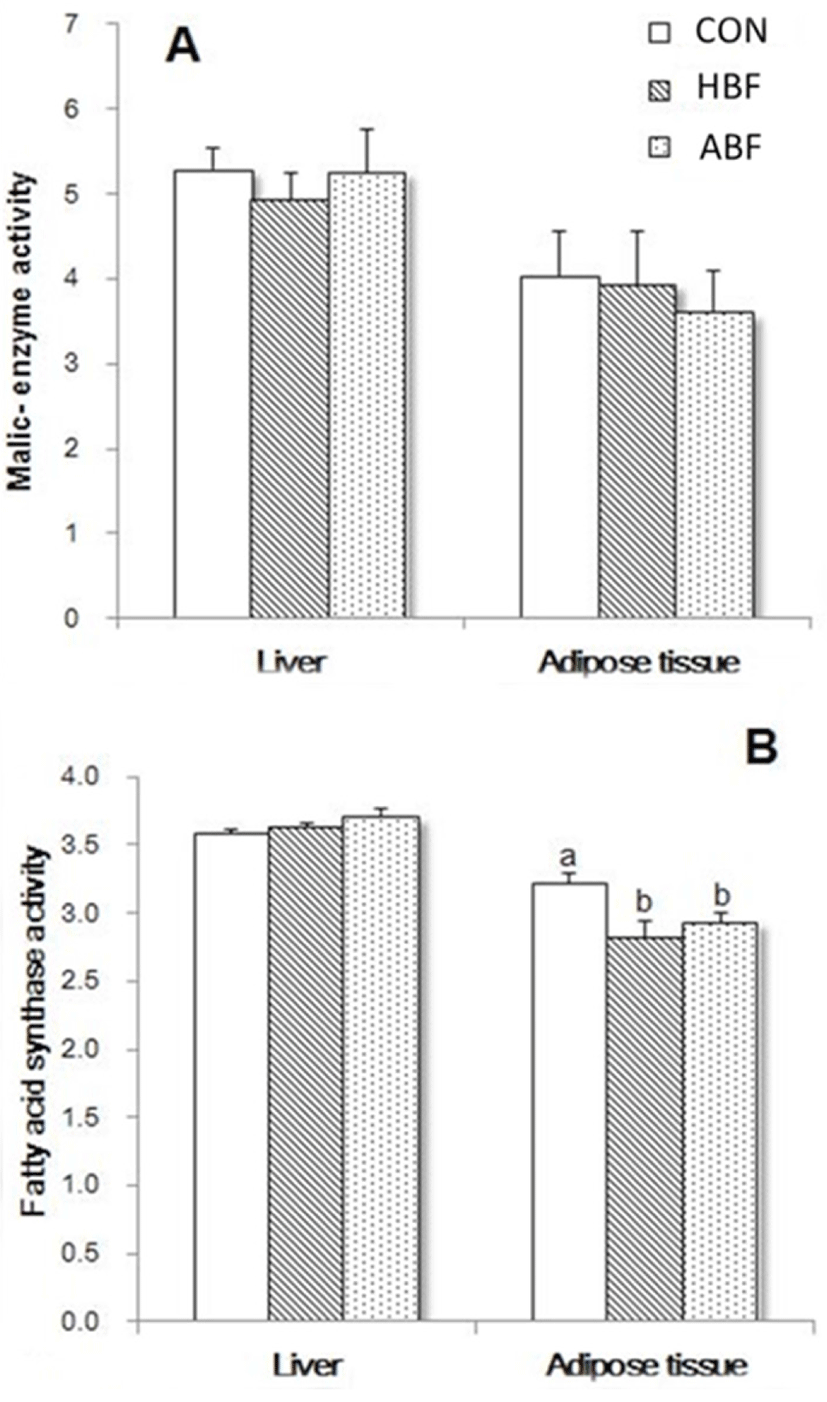
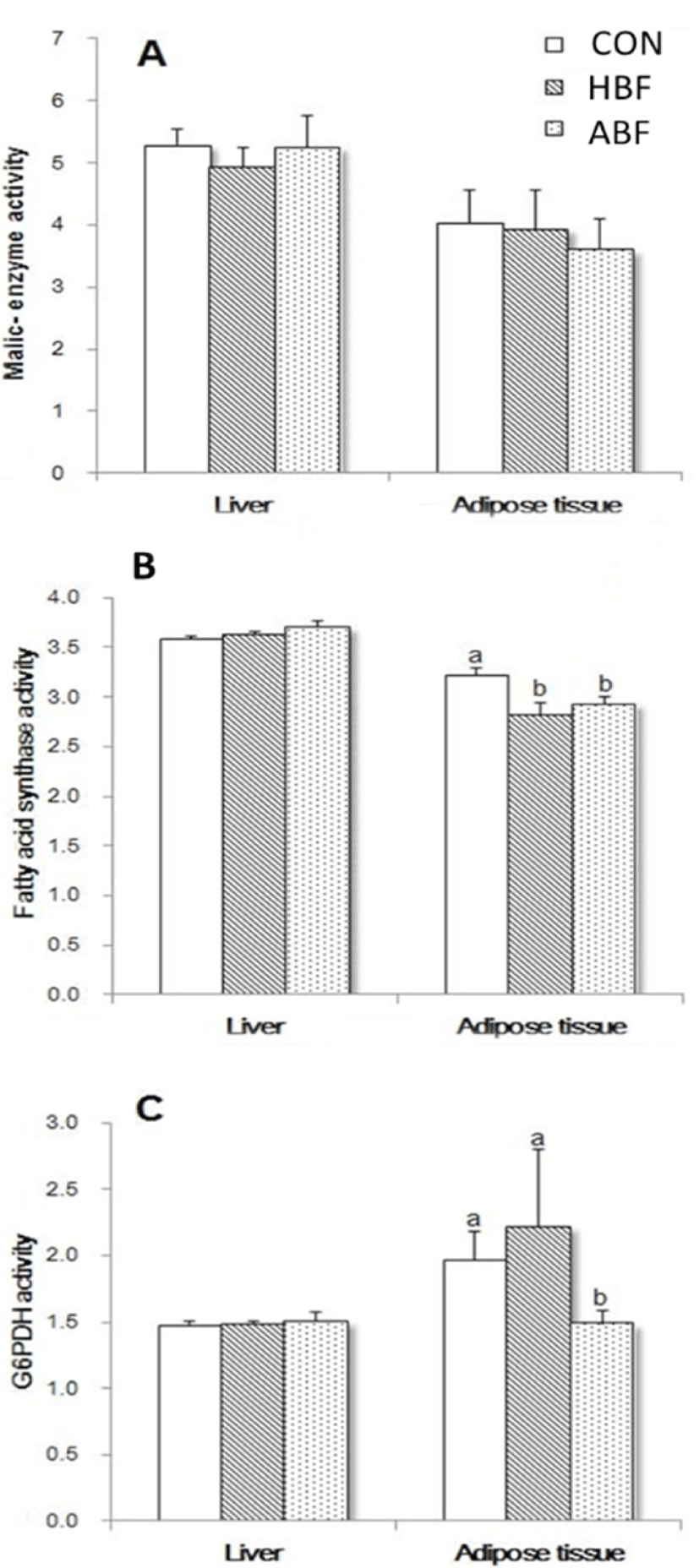
DISCUSSION
The fatty acid composition of coconut oil, ABF, and HBF was first analyzed to determine the major fatty acids present that could affect the different parameters in this study. Coconut oil mainly contained the SFAs lauric acid (49%), myristic acid (8%), palmitic acid (8%), stearic acid (2%), and unsaturated fatty acids linoleic acid (2%), and oleic acid (6%) [21]. Similarly, the coconut oil in this study mainly contained 21.1% myristic acid, 9.80% palmitic acid, 3.70% stearic, and 6.10% oleic acid, but unfortunately, lauric acid was not measured. Evidently, coconut oil is rich in SFAs and low in oleic acid. The HBF and ABF contained 45.23% and 39.51% oleic acid. The beef fats contained higher oleic acid than CON indicating that fats from beef are a good source of dietary oleic acid. Comparing the two beef fats, HBF showed higher palmitoleic and oleic acid (5.66%, 45.23%), and lower palmitic and stearic acid (24.36% and 9.59%) than ABF, while myristic acid was equally present in ABF and HBF. Cho et al. [1] reported that Hanwoo beef showed higher myristic, palmitoleic, and oleic acid, and lower palmitic and stearic acid than Angus beef. This literature is similar to the fatty acid composition of HBF and ABF found in this study where the HBF group showed higher MUFAs (oleic and palmitoleic acid) and lower SFAs (palmitic and stearic acid) than ABF.
The HBF group increased the final BW, ADG, and total weight gain of rats compared to ABF and CON similar to the report of Matsuo et al. [22] where rats fed beef tallow diets increased body fat accumulation. In terms of dietary fatty acid effect, long-chain fatty acids from C14:0 to C18:0, and MUFAs specifically oleic acid were reported to be positively associated with the human body mass index (BMI) [23]. These findings suggest that oleic acid (MUFA), and palmitic and stearic acid (long-chain SFAs) contribute to body weight gain. However, the increased body weight gain of the rats in this study might be mainly due to the increased daily FI and feed efficiency of the rats when fed HBF, suggesting the palatability of Hanwoo beef fat. Due to high IMF content, Hanwoo beef has increased flavor, juiciness, and overall palatability [4]. Hence, in this study, high oleic acid content (45.23%) in HBF can be attributed to - but could not be the prime reason to - the weight gains of the rats. The increased final BW and weight gain, and weights of internal organs of rats fed for 8 weeks of feeding period compared to 4 weeks signify that prolonged intake of fat regardless of the source (CON, ABF, HBF) could increase body weight. The significant interaction effects between the fat and feeding period on the ADG/FI imply that long feeding period of HBF decreases feed efficiency in rats. The experimental rats could have adapted to the sensory taste of the diet and thus, reduces its feeding. Furthermore, the observed nearly significant interaction effect between fat and feeding period on the weight gain of the rats signifies that prolong intake of HBF has the tendency to increase weight gain in rats.
The enzymes released in the bloodstream when the liver or heart is diseased or damaged are GOT and GPT, thereby, these enzymes serve as diagnostic indicators of liver and heart disease. The GOT and GPT levels were unaffected by the CON, ABF, and HBF indicating that these fat sources do not present deleterious liver and cardiovascular effects to rats. It is worth noting that the beef fats exerted attenuative effects on the GOT and GPT levels compared to CON although the effect of ABF and HBF did not reach statistical significance. This observation implies that the higher oleic and palmitoleic acid, and lower palmitic acid in beef fats than coconut oil have the potential to reduce the causes of diseases or damages in the liver and heart of the rats.
The HDL-C has been coined as “good” cholesterol partially due to its removal of the excess cholesterol molecules from peripheral cells and its back-transport to the liver and is inversely associated with CVD risk [24]. In this study, CON decreased, and ABF and HBF increased the HDL-C in the blood of rats. Beef fats contained higher oleic acid than coconut oil. Clinical trials have shown that increased oleic acid can increase HDL-C concentration or at least do not show a negative effect on HDL-C concentration [25,26]. Between the beef fats, HDL-C was higher in ABF than HBF although HBF had higher oleic acid content than ABF. This could be due to the higher stearic acid content of ABF than HBF. Even though stearic acid is aSFA, it is desaturated to oleic acid during metabolism [27] and this could have contributed to the positive increase of HDL-C in the ABF group. Unexpectedly, the TC level was also higher in ABF than HBF signifying that ABF might have increased LDL cholesterol levels. Other studies showed strong evidence that even-numbered SFAs such as lauric, myristic, and palmitic acids could elevate TC levels and LDL-C concentration [28–30]. Although some meta-analyses have identified that there is only a small lowering effect on the LDL-C concentration and an inconsistent effect on HDL-C content [31], our study found that HBF containing higher oleic and palmitoleic acid, and lower myristic and palmitic acid than ABF and CON increased HDL-C and lowered TC levels in the blood plasma of rats.
High plasma TG is a risk factor for insulin resistance that can affect susceptibility to atherosclerosis and cardiovascular risk. The HBF and ABF showed lower TG levels than CON. Beef fats contained higher MUFA (oleic and palmitoleic acids), and lower SFA (palmitic acid) than coconut oil and these could be associated with the reduced TG levels in the adipose tissue of rats fed with beef fats. As reported by Mensink [31], replacing SFA with MUFA reduced triglycerides in randomized controlled trials. Moreover, HBF showed lower TG levels than ABF and this could be associated with the higher palmitoleic acid of HBF than ABF. Mozaffarian et al. [32] reported that palmitoleate is inversely associated with TGs.
The fatty acid composition of adipose tissues largely reflects dietary composition, but it also reflects FA synthesis de novo coupled with chain elongation and desaturation [33]. The dietary or de novo synthesized fatty acids are absorbed in the small intestine, transported into the blood as chylomicrons with the excess fatty acids going into re-esterification for storage in either the liver or in the adipose tissue [34]. Increased lauric, myristic, palmitic acid, and myristoleic acid in the adipose tissue of rats were observed in the CON group. The CON diet contained high myristic and palmitic acids implying that these fatty acids were in excess for energy use and were stored in the adipose tissue of rats hence, the increased SFAs and MUFA myristoleic in the adipose tissue of rats fed CON diet. The lower SFAs (lauric, myristic, palmitic) in the adipose tissue of ABF and HBF could be associated to the low dietary SFAs in the two diets. The low TG level in the blood plasma coupled with the low SFAs in the adipose tissue of ABF and HBF signify that the fatty acids were almost completely oxidized to generate energy and therefore, no excess FAs were available for lipogenesis in the adipose tissue. The significant amount of oleic acid in the adipose tissue of rats fed ABF and HBF could be easily reflected by the high dietary oleic acid in the two beef fats. The oleic acid was probably high enough to potentially decrease GOT and GPT levels and elevate the HDL-C in ABF and HBF groups, and decrease the TC and TG levels in HBF. Dietary oleic acid in excess could have been used to reduce elevated levels of cholesterol hence, the lower TC level in HBF. Among the major PUFAs, ALA was the only one significantly elevated in the adipose tissue of HBF and ABF groups. The ALA is an essential omega-3 fatty acid also known to lower cholesterol levels.
The FAS is one of the major enzymes implicated in lipogenesis [35]. The HBF and ABF reduced the FAS activity in the adipose tissues of rats at 4 and 8 weeks of feeding indicating that the two beef fats could suppress the lipid synthesis for fat depot. The beef fats contained higher oleic acid compared to coconut oil and it was reported that the suppressing effect of the essential oleic acid along with other PUFA such as linoleic acid and ALA on lipogenesis was reported to be mediated by their conversion to arachidic acid (C20) [36]. The mechanisms by which the MUFA-oleic acid suppress lipogenesis are still not completely understood.
Park et al. [37] discussed that G6PDH is a rate-limiting enzyme of the pentose phosphate pathway and is connected to the inflammations in adipose tissue and insulin resistance in obesity. In this study, the activity of the G6PDH in the adipose tissue was surprisingly increased in rats fed HBF and was comparable to CON. Fatty acid composition in the adipose tissues of rats fed HBF and CON were greatly different where the HBF group had lower SFAs such as lauric, myristic, palmitic, and higher oleic acid and ALA than CON group. In addition, fatty acid contents of the adipose tissue of the HBF group were significantly comparable with the ABF group. Hence, it is highly unlikely that the increased in G6PDH activity both in CON and HBF had been caused by fatty acids. The increased G6PDH activity in the HBF group could be explained, however, by the increased body weight caused by increased feed intake of the rats fed HBF diet. In another study, it has been reported that expression of G6PDH in adipocytes isolated from obese adipose tissue [38]. The adipose tissue of the rats in this experiment has not been measured but it can be denoted that the increased body weight gain increased the G6PDH activity in the adipose tissue of rats.
CONCLUSION
Taken altogether, the two beef fats showed better blood plasma lipid profile effects than the CON. In addition, ABF and HBF reduced lipogenesis induced by a reduction in FAS activity in rat adipose tissues. Nevertheless, between the two fats, HBF showed high palatability as indicated by increased feed intake of the rats thus, increasing the weight gain of the rats. The higher oleic acid and lower myristic and palmitoleic acid in HBF than ABF group also showed an improved blood plasma lipid profile as indicated by lower TC and TG levels in HBF than ABF. Altogether, the reduced lipogenic enzyme activity, specifically that of FAS, coupled with increased HDL-C, decreased TC and TG levels in the bloodstream, reduced SFA, and increased oleic and ALA contents in rat adipose tissues indicated that HBF consumption does not pose significant risks of CVD.