INTRODUCTION
Mammalian oocytes are arrested at first the meiotic prophase I during birth [1]. The prophase I oocyte undergoes maturation, including germinal vesicle breakdown and extrusion of the first polar body [2]. Following maturation, the oocyte enters secondary meiotic arrest at metaphase II (MII), wherein the optimal time for fertilization is generally within 10 h. If this event is delayed, oocytes experience a degradative progression referred to as post-ovulatory aging (POA) [3]. POA is a common disorder characterized by reactive oxygen species (ROS) and demonstrates various reproductive phenomena of aging. So far, there has been limited discussion regarding methodologies to suppress this problem by promoting the mitochondria and oxidant system, although ROS and mitochondria are directly connected to aging.
POA plays an important role in oocyte quality for generating successful blastocysts. Since the oocyte status determines embryogenesis [4], it is reasonable that POA causes the defects in embryonic development. Post-ovulatory aged oocytes exhibit various aberrations in their functional and biochemical mechanisms. Recent evidence suggests that POA impairs mitochondrial function [5–7], lipid peroxidation [6], and apoptosis [8] by activating caspases [7,9]. Furthermore, a survey conducted by Miao [10] dealt with the possibility of having a negative influence on porcine embryo through uncompleted mitochondrial integrity, ROS, and apoptosis [10]. Therefore, embryos originating from aged oocytes are usually of poor quality and develop slowly or are damaged in subsequent embryogenesis [7,11].
Among numerous issues, mitochondria and oxidative stress are specific mediators of the POA system. This implied that aged mitochondrial damage produces cytosolic oxidative stress [12]. Simultaneously, excessive ROS triggers apoptosis, leading to cell decomposition by releasing mitochondrial apoptogenic factors such as Caspase 3 [13]. As aforementioned, we provide an insight into blocking inferior disorder, and the point of mitochondrial regulation is special to existing studies.
Bezafibrate (Bez), one of the most widely used lipid-lowering agents to treat hyperlipidemia, is a pan-agonist of peroxisome proliferator-activated receptor (PPARα) or coactivator of peroxisome proliferator activated receptor gamma coactivator 1-alpha (PGC-1α). A key aspect of PGC-1α is the control of multiple biological pathways related to energy metabolism, gluconeogenesis [14], lipid oxidation [15], mitochondrial biogenesis [15–17], mitochondrial phosphorylation [15,16], and ROS scavenging [17,18] via activation of several nuclear receptor expressions. Thus, the present study aimed to assess the management effect by Bez. However, the effect of Bez on porcine oocyte and embryo after POA remains unclear.
Therefore, we designed an experiment focusing on mitochondrial and ROS disturbances affected by Bez. To clarify the mechanism of Bez on porcine oocytes and blastocysts after POA, we hypothesized that POA will be prevented by reducing the oxidative and mitochondrial disturbance cascade.
MATERIALS AND METHODS
Unless otherwise specified, all chemicals were purchased from Sigma‐Aldrich (St. Louis, MO, USA), and all operations were carried out on a heating platform adjusted to 38.5°C.
The ovaries of prepubertal gilts were used for aspiration from a local slaughterhouse (Farm Story Dodarm B&F, Eumseong, Korea) and moved to the laboratory in 0.9% saline with 75 mg/mL penicillin G and 50 mg/mL streptomycin sulfate at 37°C. Cumulus oocyte complexes (COCs) in a 3–6 mm diameter follicle was collected by preparing a 10 mL syringe connected to an 18-gauge needle, and then washed thrice with hydroxyethyl piperazine ethane sulfonic acid (HEPES) buffer. At least three layers of COCs were acquired under a stereomicroscope (Olympus, Tokyo, Japan). Thereafter, approximately 100 COCs were cultured for 48 hrs in a 4-well dish with 500 μL of TCM-199 (11150–059; Gibco, New York, NY, USA) combined with 10% (v/v) porcine follicular fluid, 0.6 mM L-cysteine, 0.1 g/L sodium pyruvate, 10 IU/mL follicle-stimulating hormone (FSH), 10 IU/mL luteinizing hormone (LH), and10 ng/mL epidermal growth factor (EGF). The in vitro maturation medium (IVM) was covered by mineral oil, the plates were balanced in a humidified atmosphere of 38.5°C and 5% CO2 for 2 hrs.
Pipetting was repeated several times to remove the cumulus cells in HEPES added with 1 mg/mL hyaluronidase, and thereafter, oocytes which had extruded polar body were obtained. To determine whether Bez affects aging, denuded oocytes were cultured in fresh IVM medium without cysteine at 0.05, 1, 2.5, 5, 25, 50, 100, 150, or 200 μM Bez, and the same amount of dimethyl sulfoxide (DMSO) was diluted as control for 24 hrs under incubation conditions.
Aged oocytes were washed thrice with HEPES, activated by twice direct current pulses of 110 V/cm in mannitol for 60 µs and incubated in 7.5 µg/mL cytochalasin B for 3 hrs. Eventually, 4-cell and blastocysts were generated in in vitro compartmentalization (IVC) medium supplemented with 4 mg/mL bovine serum albumin (BSA) in PZM-5 [19] under incubating conditions at 48 hrs, or until Day 7.
To conduct the ROS assay, oocytes and embryos were treated with 10 μM 2’,7’-dichlorodihydro-fluorescein diacetate (D399, Invitrogen, Carlsbad, CA, USA) in poly-butylene succinate (PBS) / poly-vinyl alcohol (PVA) under incubation conditions for 30 min, and then washed thrice PBS/PVA.
For the glutathione (GSH) assay, oocytes and embryos were treated with 10 μM 4-chloro-methyl-6,8-difluoro-7-hydroxycoumarin dye (C12881, Thermo Fisher Scientific, Waltham, MA, USA) in PBS/PVA under incubation conditions for 30 min, and then washed thrice with PBS/PVA. Live imaging and intensity were captured using an epifluorescence microscope (Nikon, Tokyo, Japan). Furthermore, the fluorescence intensity was analyzed by Image J version 1.44 g software (National Institutes of Health, Bethesda, MD, USA) to quantify GSH and ROS levels.
After washing three times with PBS/PVA, the oocytes and embryos were fixed in 3.7% (w/v) paraformaldehyde (PFA) at room temperature for 30 min. Permeabilization was carried out with 0.5% (w/v) Triton X-100 in PBS/PVA in an incubator for 30 min and the cells were blocked with 1.0% BSA in PBS/PVA in an incubator for 1 hr. Samples were incubated with anti-TOM20 (1:200, SC-17764, Santa Cruz Biotechnology, Dallas, TX, USA), Phospho-Histone H2A.X (1:200, #9718, Cell Signaling Technology, Danvers, MA, USA), anti-CASPASE 3 (1:200, C8487, Sigma-Aldrich), or anti-PGC-1α (1:200, 66369-1-Ig, Proteintech, Rosemont, IL, USA) overnight at 4°C. After washing thrice with PBS/PVA, they were further incubated with Alexa Fluor 488 TM Donkey anti-mouse IgG (1:100, A21202, Invitrogen), Alexa Fluor 546 TM Donkey anti-Rabbit IgG (1:100, A10040, Invitrogen), or Alexa Fluor 488 TM goat anti-rabbit IgG (1:100, A11034, Invitrogen) at room temperature for 1 hr. Nucleus was stained with 10 μg/mL Hoechst 33342 for 10 min, and washed in PBS/PVA for three times, mounted on the slides, and imaged by confocal microscope (LSM 710 META, Zeiss, Oberkochen, Germany). The image was quantified by Zen software (version 8.0, Zeiss).
Oocytes and embryos were incubated in PBS/PVA with 500 nM MitoTracker Red CMXRos (M7512, Invitrogen) for 30 min. Samples were fixed in the PFA for 30 min after three times washes with IVM or IVC medium. TOM20 staining has been described in the subsection of immunofluorescence and confocal microscopy methods.
Blastocysts were incubated with 2.5 μM 5,5’,6,6’-tetrachloro-1,1’,3,3’-tetraethylimidacarbocyanine iodide (JC-1) (M34152, Invitrogen) in PBS/PVA at 38.5°C under 5% CO2 for 30 min. The mitochondrial membrane potential was calculated as the ratio of red florescence to green fluorescence. Red florescence corresponds to strong activated mitochondria (JC-aggregates) and green fluorescence corresponds to less-activated mitochondria (JC-monomers). Fluorescence was observed by an epifluorescence microscope (Nikon).
Three oocytes per tube were collected with 8 μL lysis buffer which contained 0.4 mg/mL proteinase K, 20 mM Tris-HCl, 0.9% Tween 20, and 0.9% Nonidet-40, and incubated at 65°C for 30 min, and 95°C for 5 min. Samples were diluted by sterile H2O in 1:25 ratio before analysis. Real-time quantitative polymerase chain reaction (RT-qPCR) was performed as described below.
DNA was obtained via RT-qPCR by using the WizPure™ qPCR Master (Super Green) mix (W1731-8, Wizbiosolution, Loco Hills, NM, USA). Amplification was conducted as follows: 95°C for 3 min, followed by 95°C for 15 s, 60°C for 20 s, and 72°C for 15 s for 40 cycles, and 72°C for 5 min as a final extension. Primer sequences at target gene ND1 are: forward (5′-CCT ACT GGC CGT AGC ATT CC-3′) and reverse (5′-GAG GAT GTG CCT GGT CGT AG–3′).
One-way analysis of variance and Student’s t-test in the Statistical Package for the Social Sciences (SPSS) were used for data analysis. Data are expressed as mean ± SEM. Every experiment was conducted at least thrice, and differences were considered significant when p < 0.05 or highly significant when p < 0.001.
RESULTS
To determine the appropriate concentration, we pre-tested the effects of Bez on blastocysts after culturing parthenotes for 7 days and the blastocyst rate and quality were considered as an optimal dose response. As illustrated in Figs. 1A and B, Bez had no effect on the blastocyst rate at 0.05 µM (20.97 ± 1.41%), 1 µM (23.38 ± 3.48%), 2.5 µM (21.56 ± 1.39%), 5 µM (25.03 ± 3.32%), 25 µM (20.39 ± 0.65%), 50 µM (24.41 ± 1.66%), and 100 µM (24.44 ± 4.68%); however, it was toxic at 150 μM (9.39 ± 0.25%) and 200 µM (9.09 ± 2.94%) compared as 0 µM (22.31 ± 1.31%, p < 0.05). Nevertheless, the blastocyst quality, reported as the total cell number stained with Hoechst 33342, was significantly increased at 50 µM treatment as compared with the control (p < 0.01, Figs. 1C and D). Moreover, 50 µM Bez was assumed as the base concentration for subsequent experiments. These results revealed that Bez improved the blastocyst quality in aged oocytes but not during blastocyst formation.
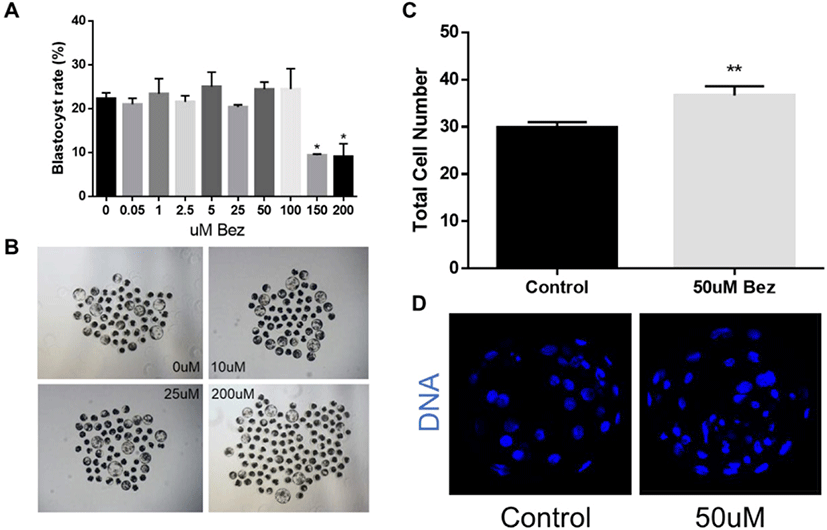
To determine Bez-induced variations in oxidative stress in aged oocytes and embryos, the green and blue intensities were measured using 2’,7’-dichlorodihydrofluorescein, and 4-chloromethyl-6,8-difluoro-7-hydroxycoumarin, respectively. ROS reaction was significantly diminished in oocytes (p < 0.001, Figs. 2A and B) in the presence of Bez, and GSH was naturally ameliorated in oocytes (p < 0.01, Figs. 2C and D). The evidence demonstrated that Bez directly prevented oxidative stress, which was induced by POA. We next investigated whether the downstream response to oxidative stress is suppressed. H2A.X and CASPASE 3 were stained using immunofluorescence staining in aged oocytes. Subsequently, the H2A.X/Hoechst intensity ratio declined (p < 0.001, Figs. 3A and B), and CASPASE 3 intensity was lower than that of the control in oocytes (p < 0.001, Figs. 3C and D). Results revealed that, Bez decreased DNA damage, and apoptosis in oocytes against oxidative stress caused by POA.
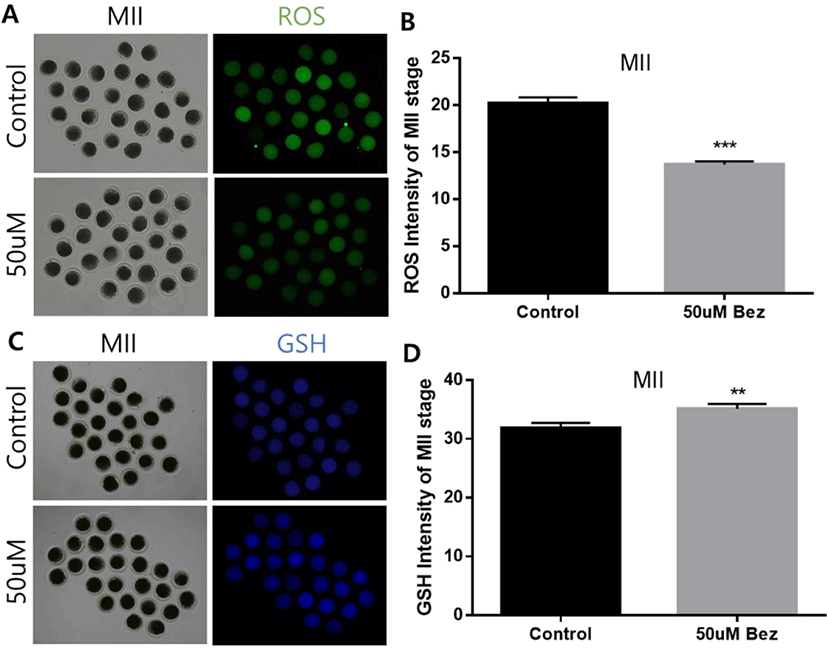
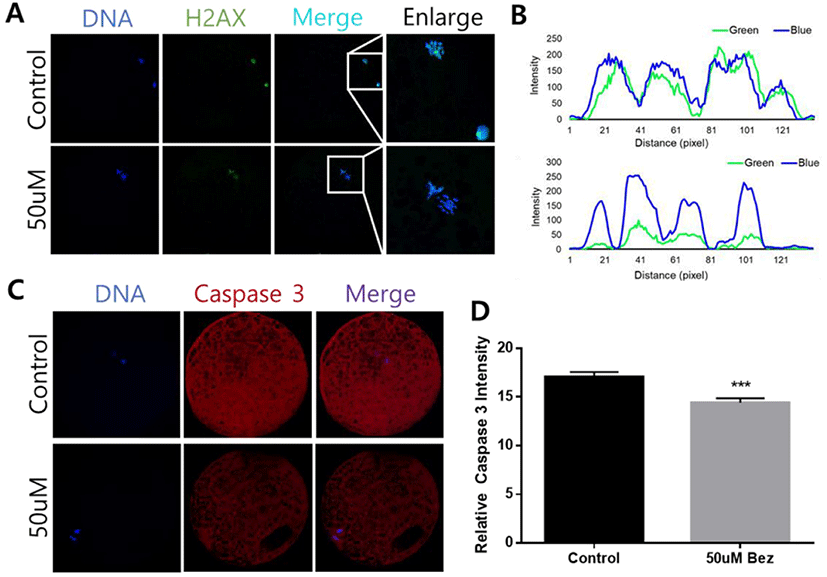
To confirm whether Bez induced PGC-1α successfully, we examined the protein expression of blastocysts via immunofluorescence staining after culturing aged oocytes. As hypothesized, we found that PGC-1α was upregulated suitably by Bez in the blastocysts (p < 0.01, Figs. 4A and B). Furthermore, ROS reaction was significantly diminished in blastocysts (p < 0.001, Figs. 5A and B) in the presence of Bez, and GSH naturally also ameliorated (p < 0.05, Figs. 5C and D). The CASPASE 3 intensity was lower than that of the control (p < 0.05, Figs. 5E and F). The evidence demonstrates that Bez-induced PGC-1α prevented oxidative stress and its apoptosis during embryo development.
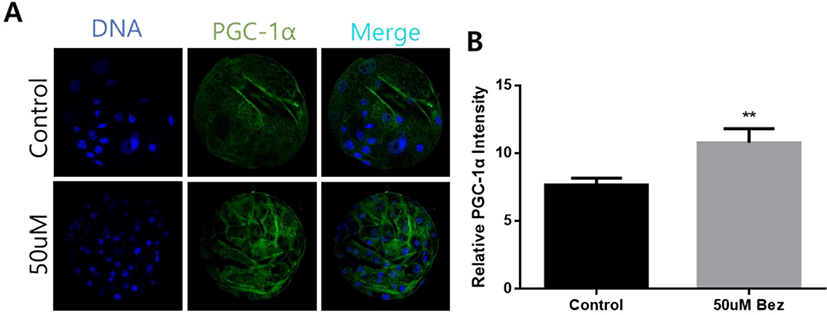
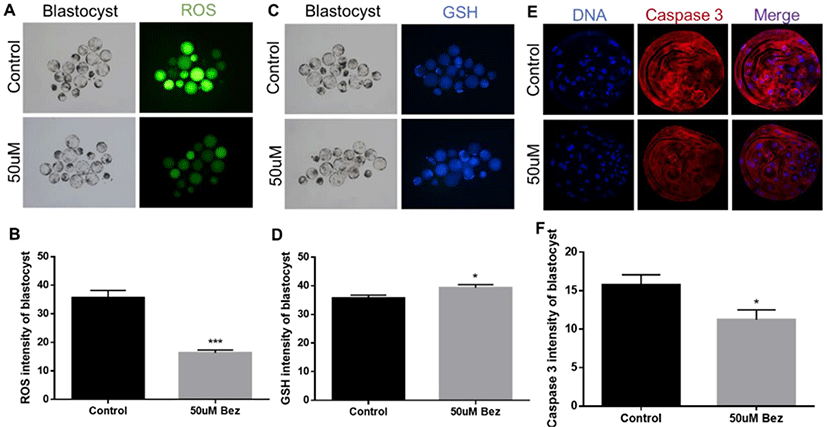
To verify that Bez improves aged mitochondrial biogenesis, active mitochondria were stained with MiotrackerRed dye, and TOM20 (mitochondrial outer membrane protein) was stained with immunofluorescence staining in aged oocytes and blastocysts. Compared with the control group, no difference was observed in protein expression such as active mitochondria, TOM20, and the MT-TOM20 ratio in oocytes. Next, while identifying the mtDNA copy number via RT-PCR, no significant difference was observed in gene expression (Figs. 6A, B, and C); however, impressive outcomes such as significantly decreased active mitochondria, increased TOM20, decreased MT-TOM20 ratio, and decreased mtDNA copy number were seen in the blastocysts (Figs. 6A, D, and E).
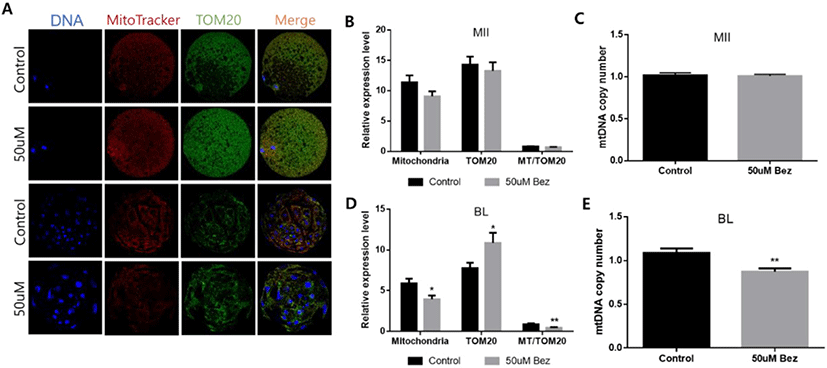
As illustrated in Fig. 7, we examined PARKIN, a marker of mitophagy, via immunofluorescence staining in aged blastocysts. Relative PARKIN intensity was remarkably improved in the Bez supplemented group (p < 0.001, Figs. 7A and B).
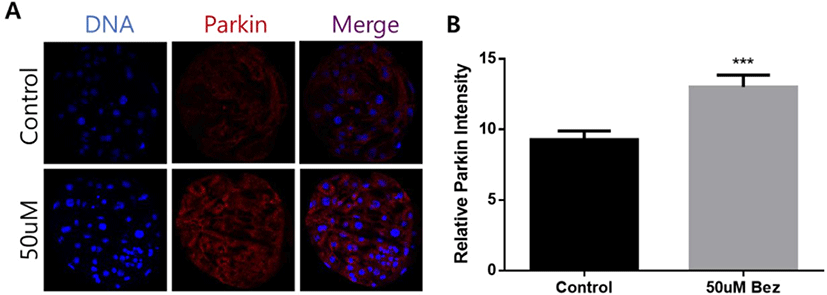
Eventually, we investigated the mitochondrial membrane potential measured by the JC-1 aggregate/monomer ratio during exposure to Bez in blastocysts. During Bez treatment, the mitochondrial membrane potential was enhanced against POA. This finding revealed that blastocysts undergo an increase in mitochondrial function (p < 0.001, Figs. 8A and B).
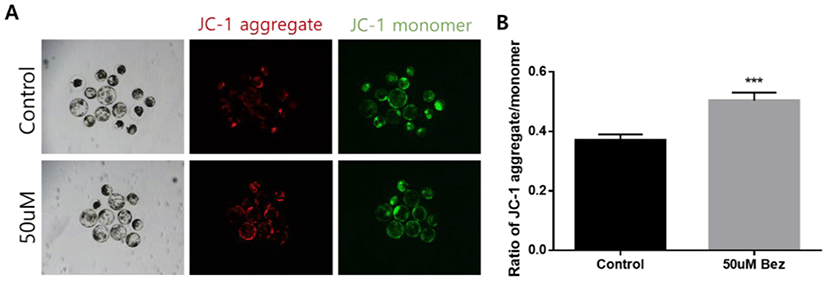
DISCUSSION
In recent years, POA, has gained immense interest in research; therefore, we plan to prevent POA in in vitro porcine oocytes. POA after ovulation is an ROS-induced process that occurs when fertilization is delayed beyond the optimal period. Numerous studies reported that POA has detrimental changes, such as mitochondrial distribution, dysfunction, and ROS, leading to DNA damage and apoptosis, has and further has a negative impact on fertilization and embryo development [5,10]. Therefore, our research aimed for an approach to deter this adverse process in post-ovulatory aged oocytes.
Eventually, Bez, which is a coactivator of PGC-1α, was treated in IVM for 24 h to delay the side effects of aging as it is widely known to activate mitochondrial biogenesis and function [20,21]. Thus, Bez is considered as an effective retrieval treatment for aged blastocysts.
The relationship between aging and ROS has been reported in the literature [10,12,13]. Reproductively aged oocytes, which follow as ROS levels increase, reveal a decline in the fidelity of protective mechanisms against ROS [22,23]. A previous study has confirmed that Bez has the potential to reduce the oxidative system via ROS reduction and GSH improvement in rats [24]. Thus, we first examined the oxidative stress levels in aged porcine oocytes. We conducted at oocytes, as ROS is the main mechanism that worsen POA and mitochondria, as mentioned earlier. Our observations were consistent with this report as well as Bez, directly alleviated ROS production and induced GSH production in oocytes. Then, we conducted the blastocysts, to verify the effect of PGC-1α. We determined that PGC-1α-induced oxidative stress declined against POA with Bez. These data suggest that Bez regulates oxidative stress during aging both in a dependent and independent manner.
When subjected to oxidative stress, a high level of ROS deteriorates the oocyte quality leading to apoptosis and damaged DNA [25]. In the present study, treatment with Bez not only prevented DNA damage in oocytes but also prevented CASPASE 3 activation in oocytes and blastocysts from aging. Our results reveal that Bez can protect downstream from oxidative stress, giving the possibility of protecting POA.
Mitochondria are organelles susceptible to ROS. Abnormal mitochondrial integrity and function are regularly followed by aging-induced oxidative damage [7,10]. To resolve this issue, we focused on the central function of PGC1α, which is the detoxification of ROS [26,27]. Moreover, we illustrated mitochondrial biogenesis under Bez management in aged oocytes and blastocysts. Oocytes mtDNA rise until fertilization, the accumulation of mtDNA evenly distributed in all cells during embryogenesis [28]. This might be particularly relevant to our results, as the representation of mitochondria and mtDNA copy number were similar to controls in oocytes. Interestingly, unlike Bez, which predicts the upregulation of mitochondrial biogenesis in blastocysts, mtDNA copy number was diminished in blastocysts. Surprisingly, Bez contributed to its negative effects on the probe for mitochondria and MT/TOM20 ratio, including TOM20 expression in blastocysts. This seems to be caused by mitochondrial decomposition. Moreover, evidence reveals that nuclear respiratory factors, which are activated by Bez, promote the expression of the major members of the mitochondrial transport protein TOM20 [29].
To reveal this interesting proof, further research is required to investigate mitophagy, which triggers mitochondrial elimination. We hypothesized that mitophagy may affect mitochondrial amount. In depolarized mitochondria, PINK1 recruits PARKIN into the outer membrane of damaged mitochondrial [30]. As expected, Bez was associated with mitophagy in porcine blastocysts.
Finally, the mitochondrial membrane potential was measured for mitochondrial function in aged blastocysts. Our findings figure out that mitochondrial membrane potential was remarkably elevated despite decreased mitochondria because mitophagy led to appropriate mitochondrial maintenance. Considerably, Bez improved mitochondrial competence of aging via mitochondrial protection and antioxidative properties. Furthermore, we provided additional evidence revealing that TOM20 overexpression results in an increase in cell proliferation [31].
CONCLUSION
This project was undertaken to design a method to prevent POA and evaluate the mechanism of Bez, which has been widely used as a lipid-lowering agent, in porcine aged oocytes and blastocysts. We assume that Bez is a valuable piece of blastocyst quality that may be crucial in mitochondrial protection and reveal antioxidative properties via PGC-1α during POA in pigs. Treatment with Bez during aging can diminish oxidative stress ditrectly and inditrectly through PGC-1α expression. Interestingly, we observed reduced apoptosis and DNA damage. Moreover, we found that Bez increased mitochondrial function even though showed mitochondrial biogenesis was reversed and active PARKIN expression was observed. Eventually, it is obvious that blastocyst quality was improved by Bez (Fig. 9). In conclusion, a greater focus on the fact that mitochondrial function was increased despite mitophagy activity could provide interesting insights for future research.
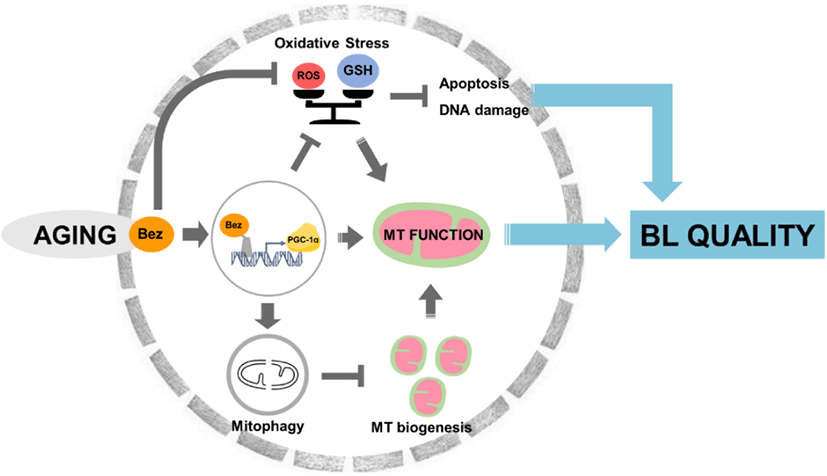