INTRODUCTION
In the last decade, there has been a significant increase in the efficiency of pork production, which has been reflected in improved offspring growth, better feed conversion, and higher reproductive performance of breeding herds. This type of pork production could change dramatically in the coming years as the need to ensure food safety, minimize environmental impacts, improve animal welfare, and optimize consumer health continues to improve the production efficiency of pork and nutritional quality. Given the magnitude of productivity changes between 2000 and 2010, most herds’ basic genetics, management, and nutrition will also change. Besides, many scientists point out the need for macro minerals such as calcium (Ca), zinc oxide (ZnO), copper (Cu), and manganese (Mn) has remained the same; research conducted primarily in the 1950s and 1960s has much to do with modern swine. For example, the National Research Council [1] and the Agricultural Research Council [2] report that animal diet estimating zinc requirements is complex and needs more explanation on interacting factors. After several studies, they suggest that a dietary zinc level of 50 ppm (assuming that dietary calcium does not exceed 0.75%) may be the best option for preventing deficiency and avoiding hyperkeratosis or parakeratosis. In 1987, the Standing Committee on Agriculture [3] studied adequate dietary zinc intake and recommended that 45 mg/kg might be optimal for growing pigs and sows. Later, the National Research Council [4] reiterated that 50 ppm of dietary zinc might be optimal for pigs at any production cycle. Since 2010, we have been using the exact dosage of dietary zinc supplementation, based on studies conducted between 1960–1980 with the primary aim of preventing parakeratosis due to zinc deficiency. The same fact withstands for Ca, phosphorus (P), Mn, and Cu. The recommended daily intake of these minerals and the estimated feed intake of pigs of different classes remained the same during the 20 years from 1979 to 1998 [1,4].
In young pigs, the zinc (Zn) content of the premix is not considered and corresponds to the requirement due to the routine use of 3,000 ppm of zinc oxide and the premix to control Escherichia coli scours. Considering that Ca, P, Zn, Cu, and Mn also become essential minerals for structural development and physiological functions of growing and breeding pigs (Fig. 1). Since minerals play an essential role in growth and reproduction, their presence can affect the quality of the final product, which ultimately affects human health. In this review, therefore, we would like to discuss the importance of minerals, different sources of mineral supplements, their function and requirements, and how they help to improve pig performance for better productivity.
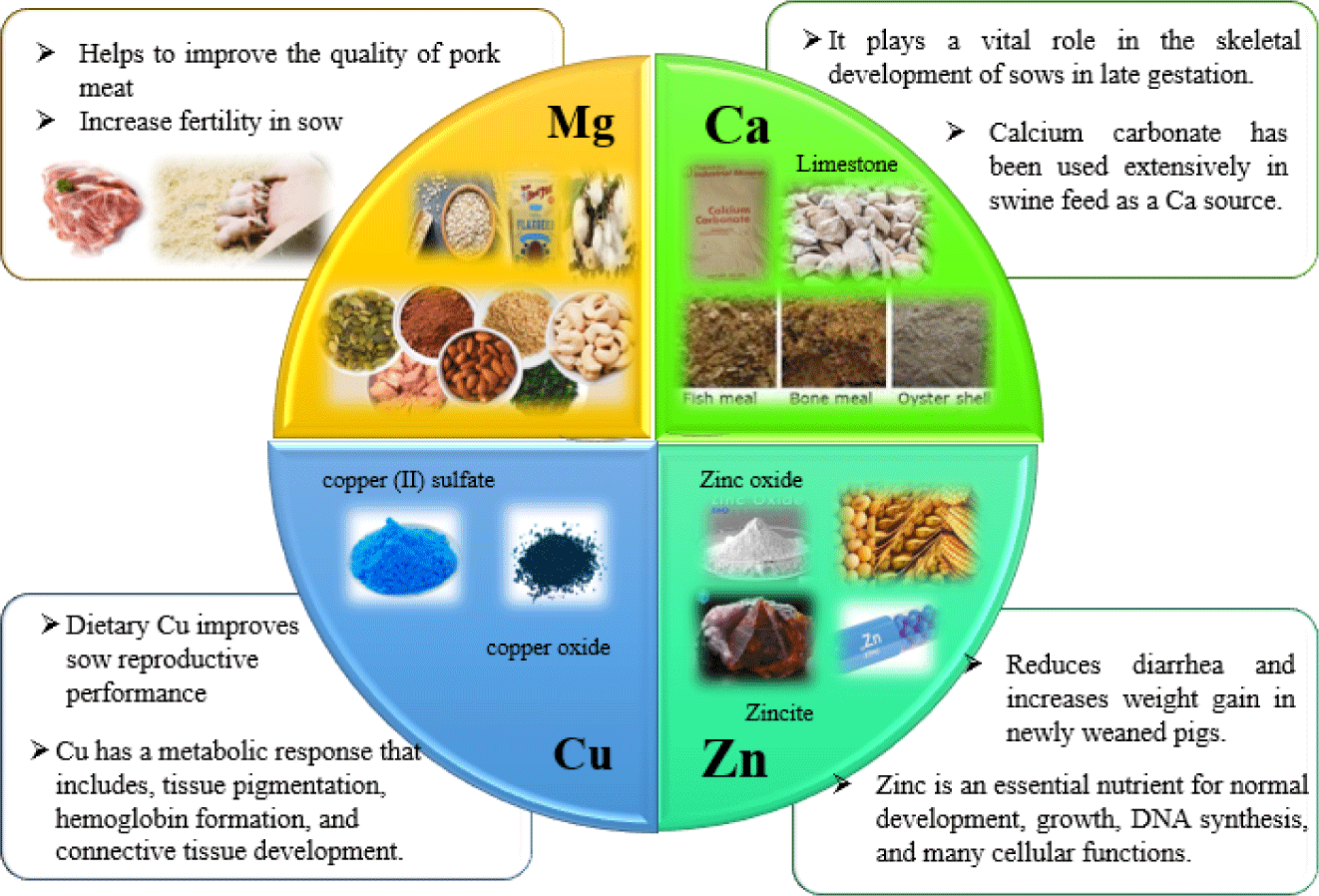
SOURCE AND FUNCTIONS OF MINERALS IN SWINE NUTRITION
Minerals are essential for the proper growth, productivity, and health of all farm animals (Fig. 2). In particular, sodium chloride (NaCl), Ca, P, sulfur, potassium (K), Mn, Fe (iron), Cu, Co, iodine (I), ZnO, Mg (Magnesium), and Se (selenium) serve as essential minerals for sustainable animal development. However, Cu, Co, I, Zn, and Se are considered to be highly toxic when added to livestock diets in excess [5]. Minerals constitute only a tiny portion in pig feed, but significantly improve their growth performance, health status, and productivity. Typically, pigs require fifteen minerals in their diet; however, macro-minerals should be supplied significantly to improve their performance. Such macro-minerals are Ca, P, sodium (Na), chlorine (Cl), K, and Mg [6]. Approximately 5% of a pig’s body weight is composed of minerals. Though these minerals are present in most feed grains, some contain inadequate levels to meet the requirements of pigs. Therefore, it is necessary to use additional minerals to balance the diet as they play an essential role in the digestion and structure of chromosomes, nerves, bones, hair, and milk, as well as in the metabolism of proteins, fats, and carbohydrates. It is also essential for most of the body’s primary metabolic reactions, making it an important factor in growth, reproduction, and disease resistance. The efficiency of mineral intake always depends on the availability, source, and concentration of minerals used in the diet, as their interactions may vary depending on the health and needs of the individual animal. To date, most pigs are kept in confinement and rely mainly on daily feed, which provides more minerals to meet daily needs, and therefore it should be added to the diet arbitrarily.
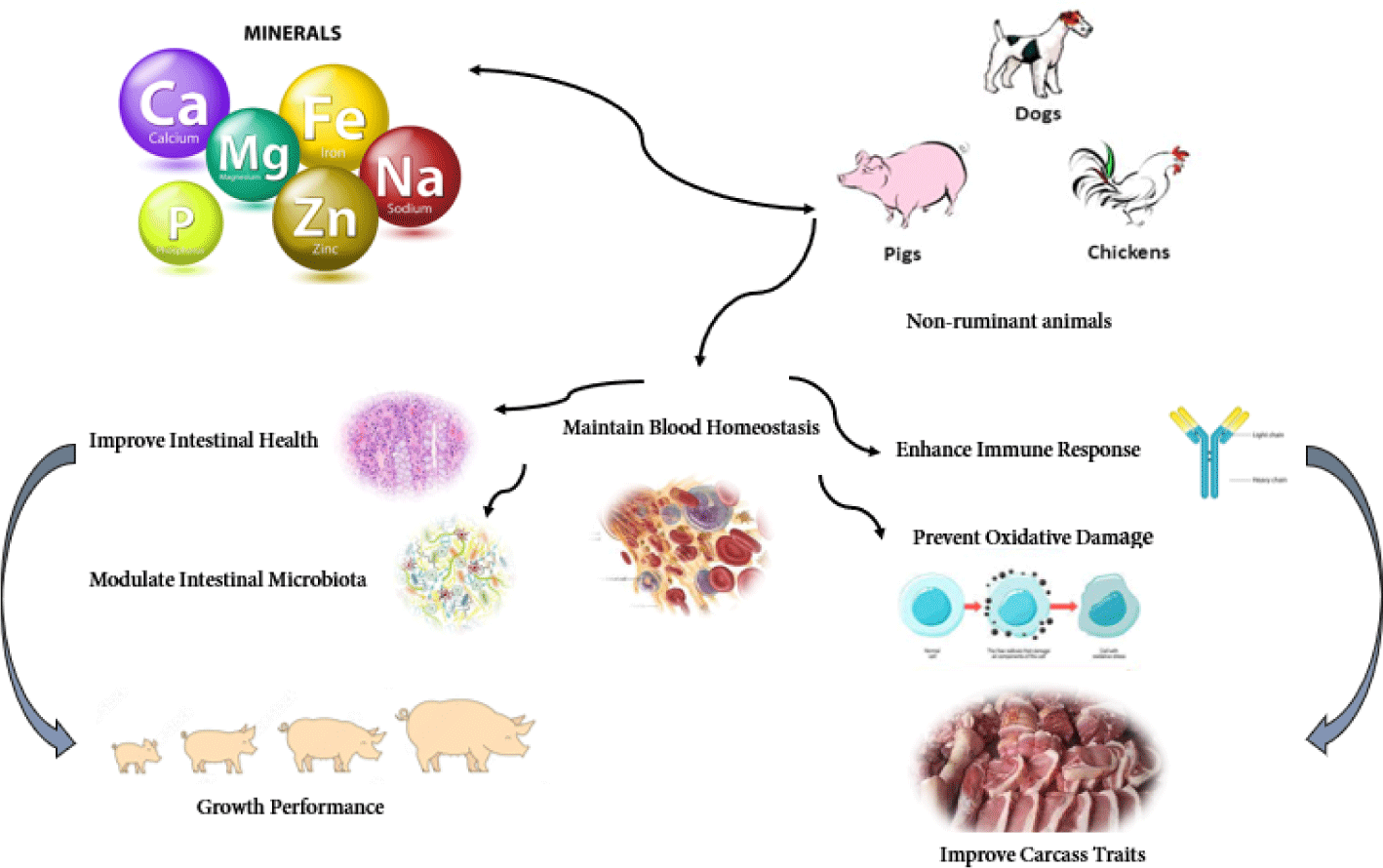
Mg is considered one of the seven most essential macro-minerals in the diet of livestock because it plays a critical role as a cofactor for more than 300 enzymes [7]. This Mg is widely distributed in green leafy vegetables, nuts, seeds, dried beans, and whole grains [8]. It is also found in feed ingredients such as wheat bran, dried yeast, flaxseed, cottonseed, and green pastures. The average Mg content (g/kg DM) in cereals is estimated to be 1.1–1.3 g, while only 3.0–5.8 g in oil and fish meal containing 1.7–2.5 g [9]. Unlike Ca, Mg is readily available to ruminants (grazers) compared to non-ruminants. As for additives, oxides, carbonates, and sulfates are highly available sources of Mg for livestock [10]. Mainly, Mg oxide (MgO) is used as the highest mineral Mg source in feeds, and the MgO usually guarantees adequate uptake of Mg ions. Administration of Mg supplements is a best practice to improve the performance of livestock, especially fertility and yield. Previously, Gao et al. [11] study demonstrated that the inclusion of 150–300 mg/kg Mg supplementation increased the conception rate of sows by 11–15% and shortened the weaning to estrus interval in gilts by nine days.
Macro-minerals are a group of mineral elements that animals require in their diet to perform numerous physiological functions. Deficiencies of these elements in the animal’s diet can lead to disease or dysfunction and must be addressed immediately. Ca and Mg are nutritionally essential minerals, especially Ca is a divalent extracellular cation, while Mg is an intracellular cation. Therefore, the cellular function of animals must be maintained by precise regulation to achieve better gastrointestinal absorption, renal reabsorption, and exchange with bone tissue in both cases. Most Ca supplements in swine diets are from inorganic sources because of inadequate Ca concentrations in the grain. In recent years, calcium carbonate (CaCO3) has been used extensively as a source of Ca in swine feed. It is usually obtained from pure limestone deposits (> 95% CaCO3) with Ca content between 36–38% and low impurities or trace traces of other minerals. These limestones have a granular appearance and are processed (extracted, selected, separated from impurities, coarsely crushed, and ground) and sorted by particle size to be used as a Ca source. Small fractions such as < 1.4 mm (~90%), < 1.0 mm (~8%–9%), and < 0.5 mm (< 1%) are commonly used in swine feed. Ca readily interacts with various minerals in sediments such as P, sulfur, Zn, Cu, Mg, I, Mn, and Co. The ionic nature of these elements promotes the formation of insoluble complexes that precipitate and hinder their absorption and utilization in the gut. This Ca is considered a macro-mineral because it is present in the diet in amounts greater than 100 ppm. Cereals, oilseed meals, and many other plant components have very high and low Ca concentrations compared to animal proteins such as fish meal, meat and bone meal, and inorganic minerals such as limestone, CaCO3, and Ca3(PO4)2. Therefore, the demand for Ca has increased in modern sows with larger litters and higher milk production. Previously, Gao et al. [11] reported that dietary Ca plays an essential role in the skeletal development of sows in late gestation. However, according to Miller et al. [12], dietary calcium concentration in milk and other mineral elements is influenced by diet. In addition, Khoushabi et al. [13] reported that higher mineral requirement (Ca) in late gestation affects colostrum synthesis by the mammary gland. in 1990, Mahan [14] said that high performance and prolonged farrowing time in sows are associated with a hypocalcemic response. Lower calcium availability reduces litter size, prolongs farrowing time, leads to more stillbirths, and causes skeletal problems in piglets.
Micro-mineral copper has a metabolic response that includes cellular respiration, tissue pigmentation, hemoglobin formation, and connective tissue development. CU is absorbed mainly in the upper gastrointestinal tract, especially the duodenum, but some Cu is absorbed in the stomach. Cu has been reported to have antimicrobial effects when administered at concentrations above pharmacological requirements (100–250 ppm) [15]. It is also an essential component of several metalloenzymes, including cytochrome C oxidase, lysyl oxidase, cytosolic Cu-Zn superoxide dismutase (SOD1), extracellular SOD3, monoamine oxidase, and tyrosinase [16]. This Cu in pig feed comes from plant or animal ingredients or mineral supplements. The most commonly used cereal grains and their by-products in swine diets contain 4.4 to 38.4 mg/kg Cu on a per-feeding basis. However, the Cu content of individual plant feed ingredients varies depending on variety, soil type, maturity, and climatic conditions during growth [17]. Oilseed meals, including soybean, cottonseed, and flaxseed, generally have higher Cu concentrations than cereal grains [18]. Copper in dairy products such as skim milk, lactose, casein, and whey powder ranges from 0.10 to 6 mg/kg [19].
Minerals can perform various functions on farm animals. They can form the structural components of body organs and tissues, exemplified by Ca, P, and Mg; bones and teeth are exemplified by silicon, while muscle protein is exemplified by P and S. Moreover, Zn and P can provide structural stability to the molecules and membranes they also comprise an electrolyte in body fluids and tissues and are involved in maintaining osmotic pressure, acid-base balance, membrane permeability, and transmission of nerve impulses [20]. Sodium, potassium, chloride, calcium, and magnesium in the blood, cerebrospinal fluid, and gastric juice are the best examples of this physiological function. In addition, they can act as catalysts in enzymes and endocrine systems, as structural components and specific components of metalloenzymes and hormones, or as activators (coenzymes) in these systems. Since the late 1990s, the number and diversity of identified metalloenzymes and coenzymes have increased. The activities can be anabolic or catabolic, life-promoting (oxidants), or life-protective (antioxidants) [20]. It can also regulate cell replication and differentiation. Thereby, Ca affects signal transduction, and selenocysteine affects gene transcription.
MINERALS REQUIREMENT FOR PIG PERFORMANCE
Mineral requirements are challenging to determine, and most assessments are based on the minimum amounts needed to overcome deficiencies, not necessarily for productivity or immunity [21]. Several studies have been conducted over the past 40 years to determine the mineral requirements of genotypes and feeding systems that differ significantly from modern commercial swine operations. The EU government has primarily become the benchmark for limiting mineral intake to reduce pollution. As a result, the use of Cu and Zn in pig feeding has been severely restricted recently. However, the industry views these two minerals as cost-effective for promoting growth and reducing post-weaning diarrhea. In 1998, the NRC [4] recommended that 400 mg/kg Mg was optimal for swine. In livestock, diarrhea was the most apparent effect of high Mg intake. However, Van Heugten [22] reported that pigs fed high Mg supplements (i.e., seven times the minimum requirement) had dramatically reduced feed intake and weight gain.
Nutritional factors and age influence the Cu requirements in pigs. Newborn pigs typically require 5 to 10 mg Cu per kg of feed for normal metabolism [23]; as pigs age increase, Cu requirements may decrease. However, Kim et al. [24] demonstrate that 75 to 250 mg/kg of Cu supplement could increase growth performance and reduce diarrhea incidence in growing and weaning pigs, respectively. Similarly, Lorenzen and Smith. [25] reported that primiparous and multiparous sows require 10 mg Cu per kg feed supplement during gestation. According to NRC [19], feeding high levels of Cu, i.e., 60 mg Cu per kg, during pregnancy and lactation improves the reproductive performance of sows compared to sows fed diets containing 6 mg/kg Cu (Table 1). However, Cromwell et al. [26] found that sows fed diets containing 250 mg/kg Cu from CuSO4 had lower shedding rates, farrowed larger litters of pigs, and had heavier pigs at birth and weaning compared to sows fed diets without added Cu.
Minerals | Requirement level | Animals | Reference |
---|---|---|---|
Magnesium | 400 mg/kg diet | Swine | NRC [4] |
Copper | 5 to 10 mg/ kg diet | Weaning pigs | Hill et al. [23] |
Copper | 5 to 6 mg/kg diet | Growing pigs | ARC [2] |
Copper | 10 mg /kg diet | Gestation sows | Lorenzen and Smith [25] |
Copper | 6 mg/kg diet | Lactation sows | NRC [19] |
FACTORS THAT AFFECT MINERAL REQUIREMENT IN PIGS
Understanding the principles of genetics, environment, herd health, management, and nutrition is more important for effective and profitable swine production because these sectors’ output may touch the production volume and profitability [27]. In addition, numerous factors affect the mineral need of animals, including weather conditions, breeding, the chemical form of elements, and mineral intake. For instance, McDowell [28] addressed that livestock gains weight rapidly during the wet season because energy and protein supplies are adequate, and thus mineral requirements are high but in the dry season, animals with insufficient protein and energy lose weight, which reduces mineral requirements. For successful breeding, pigs require certain minerals. Chromium is required for insulin production, which affects progesterone production and follicle stimulation, and luteinizing hormone. Both hormones are needed to regulate ovulation, which greatly impacts fertility and litter size [29]. Manganese is required for progesterone production, and iron and chromium are required for hormone functions that affect fetal survival during pregnancy. Additionally, breeding sows can often lack mineral intake, especially when tissue reserves are worn-out [29]. Thus, uterine capacity, which determines the number of piglets born, requires an adequate dietary intake of selenium, iron, and chromium. Furthermore, Zn has become an essential nutrient for many physiological processes in the organism, supporting health and good growth and development. The major functions of Zn on a cellular level are to catch free radicals and to prevent lipid peroxidation as part of the antioxidant system. At the same time, zinc deficit in pigs may reduce the pork quality after slaughter and processing [30].
EFFICACY OF MINERALS SUPPLEMENT IN PIGS
Zinc oxide is inexpensive and may be the best alternative to antibiotics to control diarrhea after weaning. Therefore, dietary supplementation with ZnO is commonly used 2 to 3 weeks after weaning. However, excessive Zn concentrations in feces are of concern due to the environmental impact. Therefore, many studies have been conducted to evaluate the use of BioplexTM Zn as a possible substitute for ZnO due to its higher bioavailability. In 2003, Close [21] studied the immune response to pathogens and disease prevention by maintaining healthy epithelial tissue in pigs fed diets containing zinc. However, ZnO is known to alter the diversity of the microbiota in the gastrointestinal tract [31]. In light of efforts to limit or ban the use of antibiotics in swine diets, it is critical to learn more about how zinc affects the gut microbiota and its function; thus, it may contribute to the development of feeding Strategies to benefit animals in a cost-effective and eco-friendly environment. Bone is the principal deposit of calcium, containing more than 90% of the body, whereas the remaining 1% is essential for cell metabolism, blood clotting, enzyme activation, and neuromuscular action [32]. In most animals, calcium is absorbed in the duodenum and jejunum [33]. Vitamin D plays a vital role in the absorption and metabolism of calcium and phosphorus [34]. Moreover, calcium absorption is an active and passive process mediated by vitamin D [35]. In pigs, calcium absorption is increased by vitamin D, decreased by high dietary fat content, decreased by acidic dietary pH, and decreased by phyto-P. More recently, calcium carbonate has been widely used as a supplemental calcium source [36] because of its low cost and buffering capacity. Dietary calcium and phosphorus levels have been reported to affect reproduction and, thus longevity of sows [37]. Previous studies have reported that dietary calcium content can affect glucose and lipid metabolism [11].
Similarly, Zang et al. [38] found that magnesium supplementation significantly shortened the interval between weaning and oestrus in gilts and sows (p < 0.05). It also increases the number of piglets born, born alive, and weaned in sows. Digestibility of crude fiber (secondary effect, p < 0.05) and crude protein (p < 0.05) in gilts and sows was significantly affected by magnesium during late gestation and lactation. Serum prolactin levels in sows and alkaline phosphate activity increased linearly with magnesium supplementation at farrowing and weaning (p < 0.05). Magnesium levels in sow colostrum and piglet serum increased after magnesium supplementation (p < 0.05). In addition, growth hormone levels in the serum of lactating sow piglets increased linearly (p < 0.05). Yang et al. [39] reported that a diet supplemented with various calcium sources altered average daily feed intake (ADFI) and partial gut microbial composition in weanling piglets, but had little effect on gut microbial function.
Adding 0.015% to 0.03% magnesium to sow diets could positively affect the reproductive parameters and serum mineral content [38]. Besides, sows that have passed three parities exhibit lower mineral content in their blood compared to nongravid gilts [40,41]. As the sow’s age increases, Ca and Mg stores in their body may decrease, making sows more dependent on dietary minerals, indicating an average effect and the need for dietary supplementation with high-quality soluble minerals [42]. Thus, adequate nutrition via the placenta is critical for normal fetal development because the maternal-fetal interface acts as a nutrient sensor that coordinates maternal nutrient supply and fetal metabolic needs [43,44]. Maternal mineral and vitamin status influence hormonal regulatory pathways linking maternal metabolism to the fetoplacental unit [45]. A calcium-rich diet has been shown to suppress calcitriol levels, thereby controlling lipogenesis and lipolysis. This affects lipid and energy metabolism in sows, fatty acids and triglycerides in the umbilical cord and placenta, and mRNA expression of the SLC2A2, FAS, FAB, CD36, and SCD genes, thereby affecting lipid and energy metabolism in development. Fetus and the downregulation of agouti signaling proteins [10,46,47,].
Feeding 100 to 250 mg/kg Cu to weaning pigs improved average daily gain (ADG) and ADFI [48,49]. Lower diarrhea incidence and higher feed conversion were also observed when a high concentration of Cu was included in diets for weaning and growing pigs [50]. Adding 60 to 250 mg Cu per kg to sows’ diets during late gestation and lactation reduces pre-weaning mortality [51]. It increases the weaning weight of pigs (Wallace), presumably due to increased milk production. The higher ADFI in pigs fed Cu may be due to Cu’s role in upregulating neuropeptide Y’s mRNA expression [52], a neuropeptide considered to stimulate feed intake [53]. One of the suspected mechanisms of Cu to improve growth performance is that Cu can stimulate the activities of enzymes involved in nutrient digestion [54]. Adding high concentrations of Cu increased lipase and phospholipase A activities in the small intestine [55], which may lead to increased uptake of fatty acids and improved growth performance. In addition, Cu alters the 3-dimensional structure of bacterial proteins, which prevents bacteria from performing their normal functions [56]. In a previous study, Sterritt and Lester [57] reported that copper disrupted enzyme structures and functions of bacteria by binding to S- or carboxylate-containing groups and amino groups of proteins. A copper-rich diet did not improve the growth performance of germ-free pigs, but the copper-rich diet increased ADG and ADFI in conventionally raised pigs [58]. In addition, Wang et al. [59] found that Cu-enriched diets for weanling pigs decreased the number of enterococci in the stomach and increased the lactobacillus population in the cecum of young pigs. The addition of 150 mg/kg Cu in the form of Cu hydroxychloride to diets for growing pigs also decreased microbial protein concentrations, probably due to the ability of Cu to inhibit the growth of microbes in the digestive tract of pigs [50]. This suggests that the observed improvement in growth performance in pigs fed Cu-supplemented diets is due to better digestibility and the presence of good bacteria (lactobacillus).
CONCLUSIONS
Dietary mineral concentrations are acceptable as long as the diet is palatable, does not restrict feed intake, and has the advantage of being simple and relatively constant. However, required dietary mineral concentrations are influenced by the efficiency of utilizing organic components in the diet. The total phosphorus requirement of the livestock might increase as production begins, but the proportion in the diet remains the same, while the calcium concentration required increases about 10-fold. In addition, Mg supplementation is essential to enhance farm animals’ productive and reproductive performances. Regardless of whether the requirement is expressed in quantity or concentration, it may be significantly affected by factors limiting mineral uptake and utilization, remains debatable, and requires more detailed study.