INTRODUCTION
Feed safety (i.e., safe feeds) is an essential prerequisite for producing and supplying animal products that are safe for consumers, animals, and the environment [1]. First, the demand for animal products is increasing due to the rapid increase of world population. Therefore, it is necessary to produce safe animal products for consumers because more animal products are required. Moreover, failure of controlling feed safety can lead consumers to have an increasing distrust of animal products, which will have a devastating effect on the livestock industry. Second, feed safety for animals needs to maintain animal welfare and health. Safe feeds can lead to appropriate animal production as well as animal health. Third, environmental pollution is an important issue because some feed materials are excreted to the environment such as soil and water. Therefore, safe feeds can minimize environmental pollution. Fourth, contamination of mycotoxins in feed can increase due to recent global warming. For this reason, safety management of mycotoxins in feed is important in hot and humid summers. Finally, the utilization of alternative feed resources is increasing because of rising feed ingredient prices. Therefore, it is necessary to secure safety of alternative feed resources. These problems are increasing the importance of safety management of feed due to changes in environmental conditions and the livestock industry. Therefore, all three factors relating to consumers, animals, and the environment are major issues in feed safety.
FEED SAFETY SYSTEMS IN VARIOUS COUNTRIES
Various countries have adopted their own feed safety regulations to produce safe animal feeds. Feed safety regulations are mainly focused on heavy metals, mycotoxins, and pesticides that frequently contaminate feed ingredients and become public concerns. Each country has different safe levels of hazardous materials in feed. Tables listed below show target hazardous materials and their safe levels for specific animals or general livestock from various countries such as Republic of Korea (Table 1), Japan (Table 2), Canada (Table 3), EU (Table 4), and US (Table 5).
1) Data from Ministry of Agriculture, Food and Rural Affairs [91].
1) Data from Canadian Food Inspection Agency [94].
1) Data from Official Journal of the European Communities [95].
TYPES OF FEED HAZARDS
Feed hazards such as heavy metals, pesticides, and melamine are toxic to animals and even humans after consuming contaminated animal products. Mycotoxins in feed have been extensively issued around the world.
Heavy metals include arsenic (As), lead (Pb), mercury (Hg), cadmium (Cd), chromium, zinc (Zn), manganese (Mn), cobalt (Co), and so on. Essential heavy metals, such as Zn, Mn, and Co are required for biological metabolism as a cofactor in enzymes for humans and animals [2]. On the other hand, some heavy metals such as As, Pb, Hg, and Cd are harmful to humans and animals when they consume high amounts of these heavy metals [3-5]. Moreover, WHO [6] has reported that As, Pb, Hg, and Cd are among the 10 hazardous chemicals of major public health concerns. These heavy metals are hazardous materials for animals due to the fact that they are not readily metabolized or excreted from the body [7,8]. Therefore, these heavy metals can lead to serious diseases and even death of humans and animals [6,9,10]. In general, As is easily found and distributed in rocks and soils. It is extensively used as pigments, glass, rodenticides, pesticides, and fungicides [11]. Water-soluble forms of As can be easily absorbed by the digestive system [12]. Thus, As is accumulated rapidly in tissues such as the liver, kidney, lung, and gastrointestinal tract. Until now, Pb is widely used in industrial materials such as pesticides, paint pigments, crystal glass production, and plumbing. However, Pb is one of the environmental pollutants that threaten humans and animals. In addition, Pb can cause biochemical problems, physiological problems, and behavioral dysfunctions in humans and animals [13]. When animals consume Pb-contaminated feeds, excretions of Pb are considerably slower than those of other heavy metals [7]. Moreover, Pb is usually deposited in the kidney, bone, and liver because of its slow excretion [7,12,14]. High concentrations of Pb in the bone are likely to be associated with the fact that Pb shows a great affinity for the bone by substituting calcium in the bone [7,15]. Thus, Pb might induce substantial adverse effects on performance and health. Also, Pb can accumulate in the organ of animals when animals consume high amounts of Pb [4,16,17]. Commonly, Hg is a highly toxic material and is widely used for industrial materials such as fluorescent lamps, thermometers, electronics, and medicines. Recently, consumers have increased concerns on poisoning from Hg in agriculture. Excessive Hg exposure can induce disorders in renal function, hepatic functions, and nervous systems [18]. The major toxic effects of Hg often occur in the kidney because Hg causes necrosis of proximal tubular cells and demethylation [19,20]. In addition, Hg easily can enter the animal body via the gastrointestinal tract, respiratory system, and skin [21]. Thus, prolonged Hg ingestion or excessive exposure could induce detrimental effects on animal performance and health [3,22]. The Cd is produced through industrial activities, waste disposal, and fertilizer production. Humans and animals can be easily contaminated by Cd-contaminated food, feed, and soil. Excessive or chronic Cd exposure can induce histopathological damage, kidney and liver damage, and performance reduction in animals [9,23]. Thus, Cd might cause negative effects on animal performance and health when animals consume high concentrations of Cd in diets.
For many years, pesticides have been used for agricultural farming around the world. Up to now, pesticides are widely used in crop production, insect control, and forest protection to minimize infestations from insect pests in agriculture. In the animal industry, pesticides have been used to control insects. For instance, poultry red mite (Dermanyssus gallinae) is a blood-sucking pest that often causes problems for laying hens [24]. These mites show excessive multiplication and cause constant stress in laying hens, which can lead to decreased egg production and even death. In the case of broiler chickens, workers clean and disinfect the inside after completely emptying the broiler chicken house. Therefore, pesticides are needed to produce good quality crops, increase animal productivity, and improve animal health and welfare. However, excessive use of pesticides on animals can lead to contamination animal products such as meat, milk, and eggs by pesticides. Because pesticides are lipophilic, they can accumulate easily in fat depots of animals and their products [25]. Thus, broiler chickens are likely to be contaminated with pesticides through disinfection of the broiler chicken house, bedding materials, and contaminated feed [26,27]. For this reason, broiler chicken meat contaminated with pesticides can cause major problems in meat safety and human health. In addition, many countries face serious problems of eggs contaminated by pesticides such as fipronil [28]. Currently, many poultry scientists are making efforts to find ways of raising laying hens without using pesticides to control poultry mites.
Mycotoxins are secondary metabolites produced by fungi in human foods and animal feeds [29]. More than 200 types of mycotoxins have been identified. Aflatoxin (AF) and deoxynivalenol (DON) are well-known mycotoxins that frequently contaminate animal feeds [30]. Animal feeds contaminated by these mycotoxins can exert adverse effects on animal health, productivity, and mycotoxin residues in tissues [29]. Especially, mycotoxin residues in livestock tissues can cause problems for human health when humans consume contaminated livestock products [29]. Fungal growth and subsequent mycotoxin contamination are determined by various factors, including seasons, drought, location of grain cultivation, and harvesting time [31]. Mycotoxins contamination alone or in combination with pathogens has been associated with a variety of diseases, called “mycotoxicoses” [32]. The AF is produced by the genus Aspergillus [33]. Toxigenic Aspergillus flavus can produce AF B1 and B2 [34]. Toxigenic Aspergillus parasiticus can produce AF B1, B2, G1, and G2 [34]. Negative effects of AF on hepatic necrosis through free-radical production, lipid peroxidation, and inhibition of RNA and protein synthesis have been reported in various animals [35]. The DON is a secondary metabolite produced by Fusarium graminearum or Fusarium culmorum [36]. The DON mainly contaminates corn and wheat during growing and harvesting periods [37]. Although poultry is less sensitive to DON than other animals, feeding DON-contaminated corn to poultry could cause growth depression, health problems, and reproductive failures. The DON inhibits protein synthesis, and thus, cells and tissues with high protein synthesis are more damaged by DON contamination. These tissues include the small intestine, liver, bone marrow, lymph nodes, spleen, thymus, and intestinal mucosa [36,38,39].
Melamine is a 6-nitrogen-containing organic compound and contains 67% nitrogen. Melamine is widely used for various industry such as varnishes, paints, glues, and plastic packages. Therefore, melamine can be exposed to human food and animal feed. Previous research has reported the occurrence of kidney problem and deaths in infants and animals after eating foods and feeds contaminated by melamine [40-42]. In 2007, renal failure in dogs and cats fed melamine-contaminated pet food happened in US [40]. In 2008, a rapid increase in the number of renal failures in infants was also reported in China due to melamine fraudulently added to human foods and animal diets to increase their apparent Crude protein concentrations [42]. Therefore, melamine is known to be an extremely hazardous material worldwide. Melamine can be eliminated through urine in animals [43]. In addition, more than 90% of ingested melamine can be excreted within 24 h [43-45]. When humans and animals have prolonged melamine ingestion or excessive exposure, melamine might exert extremely negative effects on humans’ health and animals’ performance and health [40,46,47]. The reason for these symptoms is that melamine can be combined with cyanuric acid to form crystals in the kidney, resulting in renal failure in humans and animals [48]. In addition, high melamine intake can increase diarrhea, polyuria, and proteinuria in animals with renal problems [41].
NEGATIVE EFFECTS OF FEED HAZARDS
The body weight (BW), body weight gain (BWG), feed intake (FI), feed efficiency (FE, BWG/FI), and feed conversion ratio (FCR, FI/BWG) are sensitive and useful indicators of general animal health. In addition, mortality is one of the toxic indicators that can detect severe toxicity. Regarding heavy metals, Jahromi et al. [17] observed that 129-d-old male broiler chickens fed diets containing 200 mg/kg Pb had less BW, FI, and FE than those fed the control diet. Seven et al. [16] demonstrated that feeding diets containing 200 mg/kg Pb decreased BWG, FI, and FE of 42-d-old broiler chickens. However, Kim et al. [4] reported that BW, BWG, FI, FE, and mortality of 35-d-old broiler chickens were not influenced by increasing inclusion levels of Pb from 0 to 400 mg/kg in diets. In addition, Erdoğan et al. [49] reported no significant difference in FI and FCR of 42-d-old broiler chickens fed diets containing 200 mg/kg Pb. Kim et al. [3] reported that increasing inclusion levels of Hg from 0 to 500 mg/kg in diets decreased BW, BWG, FI, and mortality of 35-d-old broiler chickens. Parkhurst and Thaxton [22] observed that 35-d-old broiler cockerels fed drinking water containing greater than 250 mg/kg Hg had less BW and FI compared with those drinking water containing less than 250 mg/kg Hg. Feeding diets containing 2.5 µg/kg AF decreased BW of 21-d-old broiler chickens [50]. Kim et al. [51] observed that increasing inclusion levels of DON-contaminated distiller’s dried grains with soluble (DDGS) in diets decreased BW, BWG, and FE of 28-d-old broiler chickens. Previous research has reported that feeding diets containing more than 10,000 mg/kg melamine decreased BW and FI of 21-d-old boiler chickens [52]. In addition, broiler chickens fed diets containing more than 25,000 mg/kg melamine had greater mortality than less than those fed diets containing less than 20,000 mg/kg melamine. Kim et al. [47] reported that feeding diets containing 10,000 mg/kg melamine decreased BW, BWG, and FI of 35-d-old male and female broiler chickens. Therefore, approximate toxic effects of hazardous materials can be determined based on changes in the growth performance of broiler chickens.
The liver and kidney are known as the main organs with accumulation of hazardous materials [53,54]. In addition, these two organs are responsible for the metabolism and excretion of ingested hazardous materials [54]. The feather has also been widely used as an indicator for the assessment of hazardous materials [55-57]. Moreover, meat is one of the most important target organs for human foods. It can be a big problem because humans eat meat that might have been directly contaminated by hazardous materials. A previous study has reported that 42-day-old boiler chickens fed diets containing 200 mg/kg Pb show increased Pb residues in the liver and kidney [49]. Kim et al. [4] observed that increasing inclusion levels of Pb from 0 to 400 mg/kg in diets increased Pb residues in the liver, breast meat, and feather of 35-day-old broiler chickens. Kim et al. [3] reported that increasing inclusion levels of Hg from 0 to 500 mg/kg in diets increased Hg residues in the liver, breast meat, and feather of 35-day-old broiler chickens. Melamine residues in the kidney and breast are increased in broiler chickens fed diets containing greater than 10,000 mg/kg melamine than those fed a control diet [52]. Kim et al. [47] reported that feeding diets containing 10,000 mg/kg melamine increased melamine residues in the kidney and breast meat of 35-day-old male and female broiler chickens.
The blood concentrations of aspartate aminotransferase (AST), alanine aminotransferase (ALT), gamma-glutamyl transferase (GGT), alkaline phosphate, total protein, albumin, and globulin have been widely used as indicators for liver functions [58,59]. The blood concentrations of creatinine and uric acid have been commonly applied as indicators of health status of the kidney [59,60]. Seven et al. [16] demonstrated that 42-day-old Ross broiler chickens fed diets containing 200 mg/kg Pb had greater the blood concentrations of AST than those fed a control diet. Kim et al. [4] reported that the serum concentrations of AST, creatinine, and uric acid were not influenced by increasing inclusion levels of Pb from 0 to 400 mg/kg in broiler diets. Ding et al. [61] reported that birds fed diets containing 100 mg/kg melamine and 33.3 mg/kg cyanuric acid had greater the serum concentrations of uric acid than those fed diets containing less than 100 mg/kg melamine and/or 33.3 mg/kg cyanuric acid. The serum concentrations of albumin, total protein, globulin, AST, and GGT were increased for 21-day-old broiler chickens fed diets containing 30,000 mg/kg melamine than for those fed diets containing less than 25,000 mg/kg melamine [52]. However, Kim et al. [47] observed that the plasma concentrations of ALT, AST, GGT, and total protein were not influenced by increasing inclusion levels of melamine from 0 to 10,000 mg/kg in broiler diets.
Reactive oxygen species are produced when tissue’s oxidant and antioxidant balance is disrupted [62]. To protect against oxidative stress, all living organisms have evolved with antioxidant systems of both enzymatic and non-enzymatic mechanisms [63]. Major antioxidant enzymes are superoxide dismutase, catalase (CAT), and glutathione peroxidase (GPx), whereas glutathione concentration (GSH) is a non-enzymatic antioxidant [64]. Seven et al. [16] reported that birds fed diets containing 200 mg/kg Pb had greater CAT activities in the liver and kidney compared with those fed a control diet. In addition, feeding diets containing 200 mg/kg Pb increased GSH activities in the plasma and heart of broiler chickens compared with feeding the control diet. The GPx activities in the liver were increased in 42-day-old broiler chickens fed diets containing 10 mg/kg Pb compared with those fed a basal diet [65].
METHODS FOR TOXICITY TESTING
Acute toxicity testing includes various protocols for LD50 testing, eye irritancy testing, skin irritancy testing, and other organ testing [66]. Short-term toxicity studies with rodents and non-rodents [67,68] are commonly performed for 14 or 28 days. The OECD guideline [69] recommends acute toxicity testing with a stepwise toxicity testing method using three animals of only one sex (male or female). Results of acute toxicity testing include measurements (e.g., BW) and daily detailed observations (e.g., pathology). Lipnick et al. [70] reported that females were commonly more sensitive to a conventional LD50 test than males. However, males can be more sensitive if we have information on higher sensitivity of males to structural and toxicological properties of hazardous materials. Therefore, it is desirable to use only one sex for toxicity testing. Acute toxicity testing is usually the initial step of testing toxic effects of hazardous materials. Its main objective is to provide information on potentially toxic effects of hazardous materials during their short-term exposure [71]. The first survey can be performed to predict the extent of toxicity range. Acute toxicity is achieved by administering one or a few doses of hazardous materials to animals. In addition, at least 2 to 4 times repeated-steps might be required to determine the acute toxicity of hazardous materials [69,71].
Sub-chronic toxicity testing is normally conducted for 90 days [72,73]. This test is required to study adverse effects of repeated exposure over a relatively long term of an experimental animal. Although target compounds are not toxic based on acute toxic tests, some compounds might be toxic after a long-term exposure at a low dose due to a potential increase in accumulation, changes in enzyme levels, and disruption of physiologic and biochemical homeostasis. The OECD [74-76] has reported detailed methods for sub-chronic toxicity testing. Sub-chronic oral, dermal, or inhalation toxicity may be determined based on initial information on toxicity identified in acute toxicity tests. At least 20 animals (10 males and 10 females) should be allotted to each group. At least three graded concentrations of hazardous materials should be applied. Hazardous materials are administered to animals via feeding, drinking water, or gavage. Results of sub-chronic toxicity testing include general measurements (e.g., BW, BWG, FI, and water intake) and daily and detailed observations (e.g., ophthalmological examination, haematology, clinical biochemistry, urinalysis, pathology, and histopathology).
Chronic toxicity testing is performed by administering hazardous materials for more than 12 months [77]. Chronic toxicity testing checks mutagenic, teratogenic, and carcinogenic effects [78]. Chronic toxicity testing offers prediction with regard to long-term toxic effects of hazardous materials in animals, and it may be concluded to the human safe level of the hazardous materials. The OECD [79] has reported detailed methods of chronic toxicity testing. Chronic toxicity testing can be performed using oral, dermal, and inhalation routes. In general, chronic toxicity testing is performed through oral administration. Hazardous materials are commonly administrated to animals via feeds, drinking water, or gavage. In particular, nutritional imbalance should not occur. Hazardous materials are administrated to experimental animals for approximately 12 months. The experimental period can change to be shorter (e.g., 6 or 9 months) or longer (e.g., 18 or 24 months). The experimental period can be adjusted based on requirements of particular regulatory regimes or for specific biological mechanisms. Results of chronic toxicity testing include general measurements (e.g., weighing at least once a week, FI and water intake, and FE) and daily detailed observations (e.g., ophthalmological examination, haematology, clinical biochemistry, urinalysis, and pathology).
FDA’s guidelines for toxicity studies are shown in Table 6. Short-term toxicity studies with rodents and non-rodents [67,68] are commonly carried out for 14 or 28 days. Results of the toxicity study could provide initial information for further sub-chronic or chronic toxicity studies. Hazardous materials are administered to animals via feed, drinking water, or gavage. Subchronic toxicity studies with rodents and non-rodents [72,73] are usually carried out for 90 days. Results of subchronic toxicity studies can also be used for predicting target doses of hazardous materials for future chronic toxicity studies. Chronic toxicity studies with rodents [77] should be conducted for at least 12 months. Results of chronic toxic study can be used to identify the toxicity of a food ingredient when the ingredient is fed to humans or other animals for a long period of time. Both control and treatment groups should have at least 20 rodents per sex per treatment group. In all studies, the same number, sex, species, and strains should be used. Excessive mortality due to poor animal management should be avoided. If there is a very high mortality, the study is required to be repeated. Experimental animals should be weighed at least once a week. FI and water intake should be recorded and measured weekly during the whole toxicity study. Clinical problems including opthalmological examination, hematology, clinical chemistry, urinalyses, neurotoxicity testing, and immunotoxicity can be examined.
OECD guidelines for toxicity testing of hazardous materials (chemicals) have a set of globally established specifications (Table 7). OECD guidelines refer to acute toxicity testing including procedures for acute oral toxicity [80], acute dermal toxicity: fixed dose procedure [81], acute inhalation toxicity [82], acute dermal irritation/corrosion [83], acute eye irritation/corrosion [84], acute oral toxicity – fixed dose procedure [85], acute oral toxicity – acute toxic class method [69], and acute oral toxicity: up-and-down procedure [86]. Detailed methods are presented in Table 7.
EFSA [87] has reported technical guidelines regarding tolerance and efficacy studies in target animals. The experimental design should be set based on hazardous materials and animal species. In addition, the experiment should be carried out considering health status and husbandry conditions of animals. The number of animals and replicates should be set within the permissible range of statistical analysis. The experimental design of a tolerance study should have at least three dietary groups: (1) a control group that should not contain hazardous materials, (2) a treatment group 1 that contains hazardous materials at the maximum concentrations in diets and/or water, and (3) treatment group 2 that contains 10-fold higher concentrations in diets and/or water as compared to treatment group 1. If 100-fold higher concentrations are tolerable, haematology or routine blood chemistry is not mandatory. If hazardous materials are tolerable only at a lower concentration, the study should be designed with a margin of safe levels for hazardous materials. The EFSA [87] has also set the experimental period for a tolerance study. The proposed period of the tolerance study is presented in Table 8. EFSA’s technical guidance also includes bioavailability and bioequivalence testing methods for digestion studies, balance studies, and palatability studies.
Linear regression analysis is used to calculate the intercept and slope. Based on linear regression analysis (Fig. 1), anybody can estimate safe levels of hazardous materials by regressing dietary concentrations of hazardous materials to concentrations (i.e., residue) of animal products such as meats, milk, and eggs. For example, Kim et al. [47] have carried out a regression analysis and indicated a linear relationship between dietary melamine concentrations (x variables) in broiler diets and melamine concentrations in breast meat (y variables). Melamine was added to the basal diet at a concentration of 0, 250, 500, 750, or 1,000 mg/kg melamine. Results indicated that the linear regression was Y = 0.003X + 0.0583 (R2 = 0.968; p < 0.01). Based on this equation, safe level of melamine concentrations in broiler diet was estimated to be 814 mg/kg when safe concentrations of melamine in the breast meat were set to be 2.5 mg/kg according to the international safe limit [46,88]. Therefore, a linear regression analysis can identify the safe level of livestock products based on hazardous material residues in animal products and the value (i.e., safe limits) set for food safety regulations.
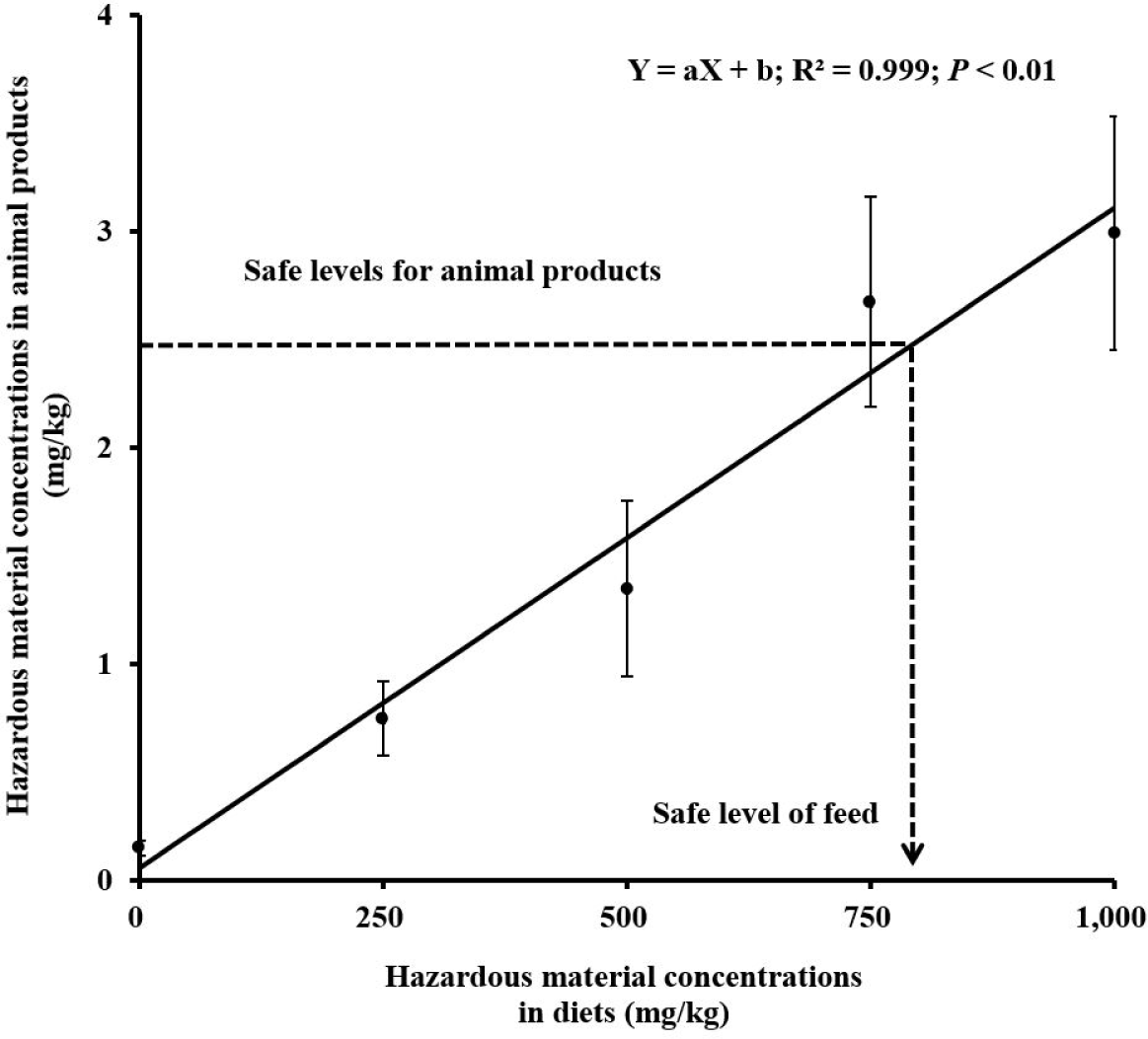
A one-slope broken-line analysis [89,90] can estimate toxic levels of hazardous materials based on animal performance (Fig. 2). The first line remains in the plateau state and then shows breakpoint. At the breakpoint, the second line indicates a decreasing slope. This breakpoint provides the toxic level of a diet or water. In our previous experiment, for example, seven broiler diets were formulated to provide increasing melamine concentrations of 0, 250, 500, 750, 1,000, 5,000, or 10,000 mg/kg in diets. Results indicated that birds fed diets containing 10,000 mg/kg melamine had less BWG compared with those fed other diets. This result showed a toxic level of only 10,000 mg/kg melamine in diets for the productivity of broiler chickens. However, this result did not provide an accurate toxic level in diets based on animal performance because the toxic level was solely determined by how we set treatment levels. Thus, we predicted the toxic level of melamine in broiler diets based on BWG using a nonlinear regression (NLIN) procedure of SAS (SAS Institute., Cary, NC, USA). The one-slope broken-line analysis was as follows: Y = L + U × (X – R), where L was the maximum value of BWG (asymptote), U was the slope, X was melamine concentration in diets, and R was the toxic level of melamine in diets (breakpoint x value). Results indicated that the equation was Y = 1,851 – 0.0404 × (X – 4,292). Based on this equation, the toxic level of melamine in broiler diets was predicted to be 4,292 mg/kg. Therefore, the one-slope broken-line analysis could be used to obtain accurate toxic levels of hazardous materials.
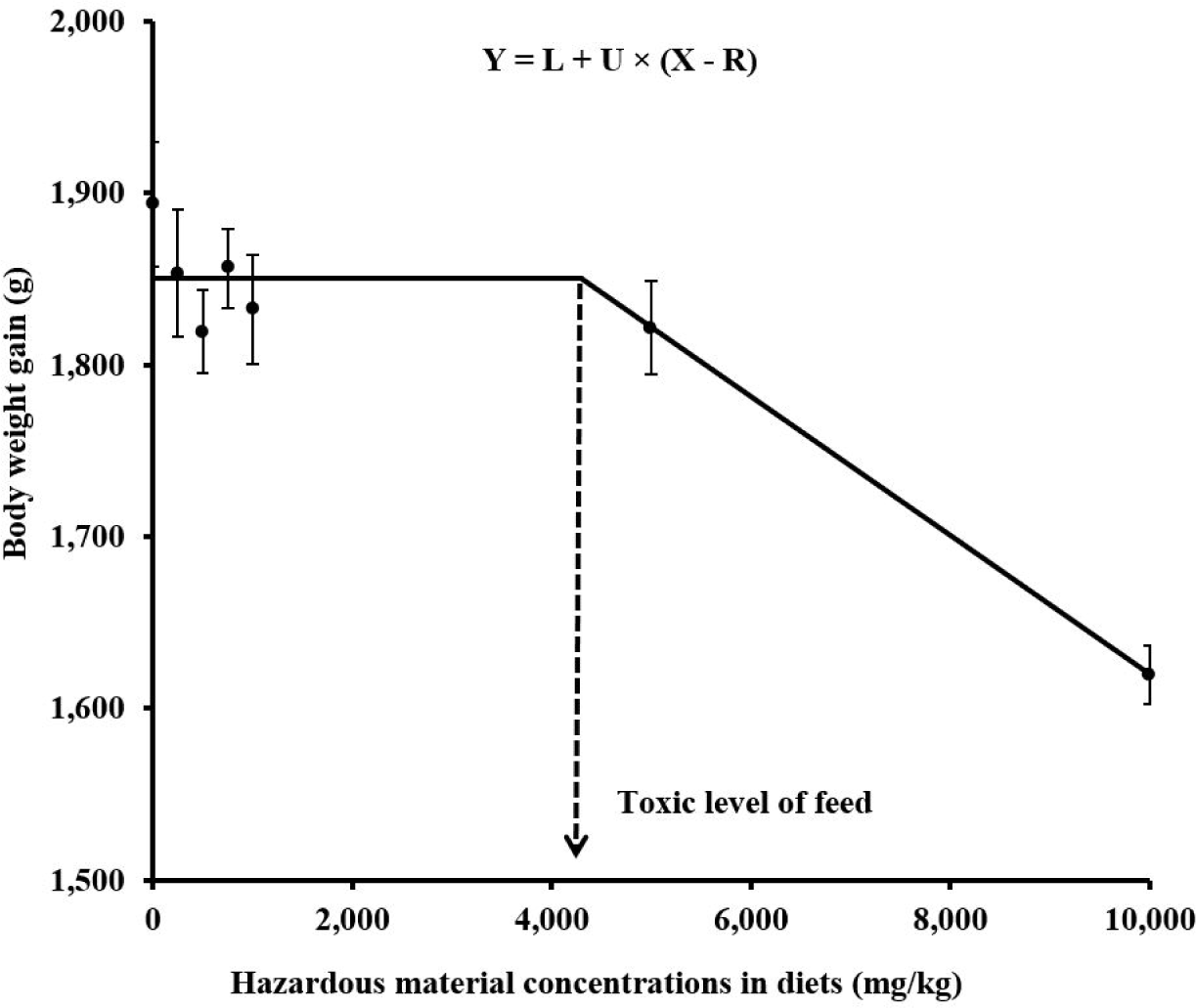
In conclusion, feed safety is an essential requirement for protecting humans, animals, and the environment. Institutions of various countries are responsible for protecting and promoting public health through animal feed safety regulations. There are many hazardous materials that threaten the safety of feed in the world. Hazardous materials that negate animal and human health include heavy metals, pesticides, mycotoxins, and others. The chance of outbreaks of new hazardous materials is increasing. To set up appropriate toxic levels (or safe levels), various toxicity methods can be used to determine toxic levels of hazardous materials for humans and animals. Appropriate toxic testing methods should be developed and used to accurately set up and identify toxicity and safe levels of hazardous materials in food and feed.