INTRODUCTION
Sodium chloride (NaCl) has been used to enhance sensory acceptability in various foods, and the Na+ cation and Cl− anion provide saltiness and flavor [1]. Additionally, in meat products, NaCl improves protein functionality and gel properties through the solubilization of salt-soluble proteins [2,3]. However, high dietary sodium intake can cause hypertension and induce stroke and cardiovascular diseases [4]. Accordingly, the demand for processed meat products with low sodium content is increasing. Therefore, NaCl substitutes have been developed to satisfy the consumer demand for healthy food and maintain the high quality of meat products [2,4].
NaCl substitutes used in the meat industry include other chloride salts, such as potassium chloride (KCl), calcium chloride (CaCl2), and magnesium chloride (MgCl2), and non-chloride salts, such as phosphate [4,5]. KCl, CaCl2, and MgCl2 are commonly used as alternatives to NaCl owing to their ease of use and low cost [6]. Most studies have reported that monovalent salts, such as KCl, have similar effects to NaCl, and that divalent salts degrade the properties of meat products, such as water retention and texture [7]. However, in some studies, the intermolecular bonding and microstructure of the gel according to each salt have been reported differently. Therefore, it is assumed that the difference in the effect of each salt is due to not only the difference between the monovalent and divalent cations but also the characteristics of each constituent ion.
Myofibrillar proteins (MPs) compose 55%–65% of the total meat protein [6], and myosin is the main protein that forms the gel. Myosin forms a gel that can trap water and fat through denaturation and aggregation by head–head or tail–tail interactions upon heating. Previous studies found that the solubility of myosin was affected by the different chloride salts that resulted in the different quality properties of heat-induced gel [8–10]. In addition, some studies reported that the structure of myosin in meat could be affected by the processing conditions, and that was an important factor in the gel formation of myosin [6,11,12]. Therefore, observing the myosin structure changed with different chloride salts can support the understanding of the mechanism in the changes of gel quality. However, there are few studies on the changes in the myosin structure with different chloride salts. Therefore, this study aimed to investigate the effects of different chloride salts on the solubility and structure of pork myosin and their relationship with the gel properties.
MATERIALS AND METHODS
MP was extracted as previously described [13], with slight modification. Fresh pork picnic shoulder meat was procured from a local market (Daejeon, Korea). The excess fat and connective tissue were removed, and the meat (80%) was minced with ice (20%) and L-ascorbic acid (0.03%) using a silent cutter (12VV, Sirman SpA, Curtarolo, Italy). The ground muscle was mixed with five volumes of cold isolation buffer (2 mM MgCl2, 0.1 M KCl, 1 mM ethylene glycol tetraacetic acid [EGTA], 10 mM potassium phosphate, pH 7.0) and stirred at 4°C for 3 h, and the mixtures were centrifuged at 2,090×g for 10 min at 4°C (ScanSpeed 1580R, Labogene ApS, Lillerød, Denmark). This process was repeated thrice. The pellets were washed twice with three volumes of distilled water.
The washed MP pellets were mixed with tetrasodium pyrophosphate (0.3%) and homogenized for 1 min using a food mixer (ITB-400H, Guangdong Xinbao Electrical Appliances Holdings, Guangdong, China). Each chloride salt was added to the samples of the salt-added group, except the control sample, according to the fixed ionic strength (0.34 M) to the MPs (NaCl: 2% NaCl; KCl: 2.55% KCl; CaCl2: 1.27% CaCl2; MgCl2: 1.08% MgCl2). Additionally, the MPs of all groups were homogenized (ITB-400H, Guangdong Xinbao Electrical Appliances Holdings) for 30 s, vacuum-packed, and stored at 4°C until use.
The MP samples (2 g) were mixed with 10 mL of distilled water and stirred at 4°C for 2 h. The suspension was centrifuged at 2,090×g for 15 min (ScanSpeed 1580R, Labogene ApS), and the protein content of the supernatant was determined using a colorimetric Bio-Rad protein assay (#5000006, Bio-Rad Labsoratories, Hercules, CA, USA). The protein solubility was calculated as the percentage of protein content in the supernatant to the total protein content in the 2 g samples.
The surface hydrophobicity of the sample was determined as described by Lee et al. [14] using bound bromophenol blue (BPB). First, 1 mL of diluted samples (1 mg/mL) were reacted and mixed with 200 μL of BPB (1 mg/mL in distilled water). After reacting the sample with BPB, the supernatant was diluted 10-fold with 0.1 M potassium phosphate buffer (pH 7.4). The absorbance was measured at 595 nm using a plate reader (Varioskan LUX, Thermo Fisher Scientific, Waltham, MA, USA) against a blank of potassium phosphate buffer. A control containing no sample was prepared by reacting BPB with phosphate buffer. The bound BPB is expressed as:
SDS–PAGE was performed as previously described by Lee et al. [15]. The supernatant and pellet obtained during protein solubility measurements were used as samples. Polyacrylamide gels with a concentration of 12.5% were used. The samples were mixed with equal volumes of 2× sample buffer (125 mM Tris-HCl, 20% glycerol, 2% SDS, 2% mercaptoethanol, and 0.02% BPB). The mixed samples were heated to 95°C for 90 s on a heating block. An aliquot (10 μL) of the sample (20 μg protein) and 5 μL of the protein ladder (3454A, Takara Bio, Shiga, Japan) were loaded. Electrophoretic separation was performed using an AE-6531 mPAGE system (ATTO, Tokyo, Japan), applying 20 mA for 120 min. The proteins in the gel were stained using a staining solution containing 0.1% Coomassie brilliant blue and de-stained with a 10% acetic acid solution. The stained gels were scanned using a GS-710 densitometer (Bio-Rad Laboratories). The scanned gel image was analyzed using Image Master 2D Platinum v5.0 (GE Healthcare, Seoul, Korea).
Myosin was extracted using a method reported by Lee et al. [14] with slight modification. First, 3 g of the control and salt-added MPs was mixed with 15 mL of G-S buffer (0.4 M NaCl, 5 mM MgCl2, 5 mM Na4P2O7, and 150 mM Na3PO4, pH 6.0) and stirred for 20 min, followed by centrifugation at 2,090×g for 10 min. The pellet was collected, and the supernatant was filtered through a 1.0 mm mesh strainer. Thereafter, 10 mL of distilled water was added to both the pellet and filtrate of the supernatant, followed by stirring for 10 min and centrifugation at 2,090×g for 10 min. Each pellet was treated with 0.1 M potassium phosphate buffer (pH 7.4) and diluted to a protein concentration of 0.13 mg/mL. The myosin extract was used to measure the myosin denaturation pattern using circular dichroism (CD).
The secondary structure and thermal denaturation of the myosin extract were measured as described by Shimada et al. [16] using a CD spectrometer (Chirascan VX, Applied Photophysics, Leatherhead, UK). The myosin solution was diluted (0.13 mg/mL) in 0.1 M potassium phosphate buffer (pH 7.4) and transferred to a quartz cell with a path length of 1 mm. The secondary structures of the samples were measured from 200 to 260 nm at a scan rate of 100 nm/min at 25 and 98°C. The compositions of the α-helix, β-sheet, β-turn, and random coil were estimated using CDNN software (version 2.1, Applied Photophysics). The thermal denaturation of the samples was measured by following the CD intensity (millidegree) at 222 nm from 25 to 98°C; the temperature was increased at 5.0°C/min.
The fresh pork picnic shoulder meat with excess fat and connective tissue removed (120 g) was mixed with ice (30 g), sodium pyrophosphate (0.3%), L-ascorbic acid (0.03%), and salts (control: none; NaCl: 2% NaCl; KCl: 2.55% KCl; CaCl2: 1.27% CaCl2; MgCl2: 1.08% MgCl2) using bowl cutters (C4, Sirman SpA) for 3 min. The meat batter was prepared thrice for each treatment and stored in a refrigerator at 4°C for 12 h before cooking. The gel (50 g meat batter) was molded in a stainless-steel container (55 mm × 30 mm) and heated in a water bath at 80°C for 30 min. After cooling at 25°C for 30 min, thirty gels (two gels/each treatment/each batch) were used to analyze the quality.
The pH of the raw meat batter prepared with the different chloride salts was measured. One gram of meat batter sample was homogenized in 9 mL of distilled water using a homogenizer (T25 basic, IKA®-Werke GmbH & Co. KG, Staufen, Germany). The homogenates were centrifuged at 2,090×g for 10 min (ScanSpeed 1580R, Labogene ApS) and filtered using Whatman No. 4 filter paper (Whatman, Maidstone, UK). The pH of the filtrate was measured using a pH meter (SevenEasy, Mettler-Toledo International, Schwerzenbach, Switzerland).
The cooking yield (CY) of pork gels manufactured with the different chloride salts was measured. Moisture on the surface of the cooked gel samples was removed, and the gel samples were weighed (G).
The hardness of the gel samples was analyzed using a texture analyzer (Model A-XT2, Stable Micro Systems, Godalming, UK). The cooled gel sample at 25°C for 30 min was cut (2×2×1.5 cm3) and subjected to 70% compression using a 70-mm-diameter compression platen at a test speed of 2 mm/s at 25°C. The maximum force (N) was recorded as the hardness.
The study was conducted in three iterations (three batches, two samples/each treatment/each batch), and the results were statistically analyzed using a mixed model with a randomized complete block design (batch as a block). The results were expressed as the least-square mean with SEM, and the significance of the main effect was tested using Tukey’s multiple comparison test (p < 0.05). Statistical analyses were performed using the SAS program (version 9.4, SAS Institute, Cary, NC, USA).
RESULTS AND DISCUSSION
Solubilized proteins, particularly myosin, can form desirable heat-induced gels with captured moisture and fat by aggregating and forming interfacial protein films in comminuted meat products [7]. In this study, the MP samples were mixed with various chloride salts at the same ionic strength after the dissociation of actomyosin by sodium pyrophosphate. The solubility of the MPs was higher with NaCl than with KCl (p < 0.05, Fig. 1). Additionally, the divalent cation salts, such as CaCl2 and MgCl2, exhibited a lower solubilization ability on MPs than that exhibited by the monovalent cation salts (NaCl and KCl). Gordon and Barbut [7] reported that when NaCl, KCl, CaCl2, and MgCl2 were added to chicken breast meat batter with the same ionic strength, the extracted protein amount increased in the order of NaCl, KCl, MgCl2, and CaCl2. The higher ability of Na+ than that of K+ to solubilize MPs can be explained by the Hofmeister series, ordering the specific ionic effect on proteins in terms of the interaction among salt ions, water, and proteins. These ions are divided into “kosmotropes,” which have a salting-out effect on protein with the stabilization of the protein structure, and “chaotropes,” which have the opposite effect [17]. According to the Hofmeister series, K+, Na+, Mg2+, and Ca2+ in this order, move from kosmotropes to chaotropes. Although MgCl2 and CaCl2 are more chaotropic salts than NaCl and KCl, various studies have reported that the protein solubility of the divalent salt-added meat products was lower than that of the monovalent salts [7,18]. Salting out of various food proteins with divalent metal ions has been reported in previous studies. Arii and Takenaka [19] reported Mg2+- and Ca2+-associated soy proteins using salt bridges with protein carboxyl groups. In general, for NaCl, Cl− anions selectively permeate the myofibrils to increase the negative charge and intramolecular electrostatic repulsion. Thereafter, myofibrils swell, and more water is trapped in the heat-induced gel matrix. Additionally, Na+, a monovalent cation, can form an “ion cloud” around the myofibrils and increase repulsion, causing an increase in protein solubility. However, the divalent cation of the divalent salt can make two cross-links, unlike the monovalent cations, such as Na+. They can attach to two other protein molecules to cause protein aggregation. Zhu and Damodaran [20] found that whey protein was precipitated by aggregation in a salt solution containing MgCl2 or CaCl2. In this study, the solubility of MPs was significantly higher in Mg2+ than in Ca2+. Gordon and Barbut [7] found that CaCl2 exhibited a lower solubility of chicken MPs than that exhibited by MgCl2. These results might be related to the different effects of divalent cations, Mg2+ and Ca2+, on the conformational changes of proteins with different properties and binding sites of divalent cations on protein [20]. Mg2+ is smaller than Ca2+, closer to Na+ in size, and more electronegative than Ca2+; furthermore, it has different interaction properties on proteins [10]. Gordon and Barbut [9] reported that CaCl2 and MgCl2 reduced the pH of raw meat batter compared with NaCl at the same ionic strength. In this study, the pH levels of the meat batter manufactured with CaCl2 and MgCl2 were 5.79 and 6.36, respectively, both lower than that of the meat batter containing NaCl (6.52) at the same ionic strength (Fig. 1). The solubility of the MPs decreased with a decrease in the pH of the meat system near the isoelectric point of the MPs [21]. Therefore, the decline in pH may be a reason for the low solubility of MPs with divalent cations, particularly Ca2+. Although the control group had the significantly highest pH among treatments (Fig. 1), the MP solubility of control was similar to those of the CaCl2 and MgCl2 groups because the ionic strength of control was the lowest among treatments in the absence of chloride salt.
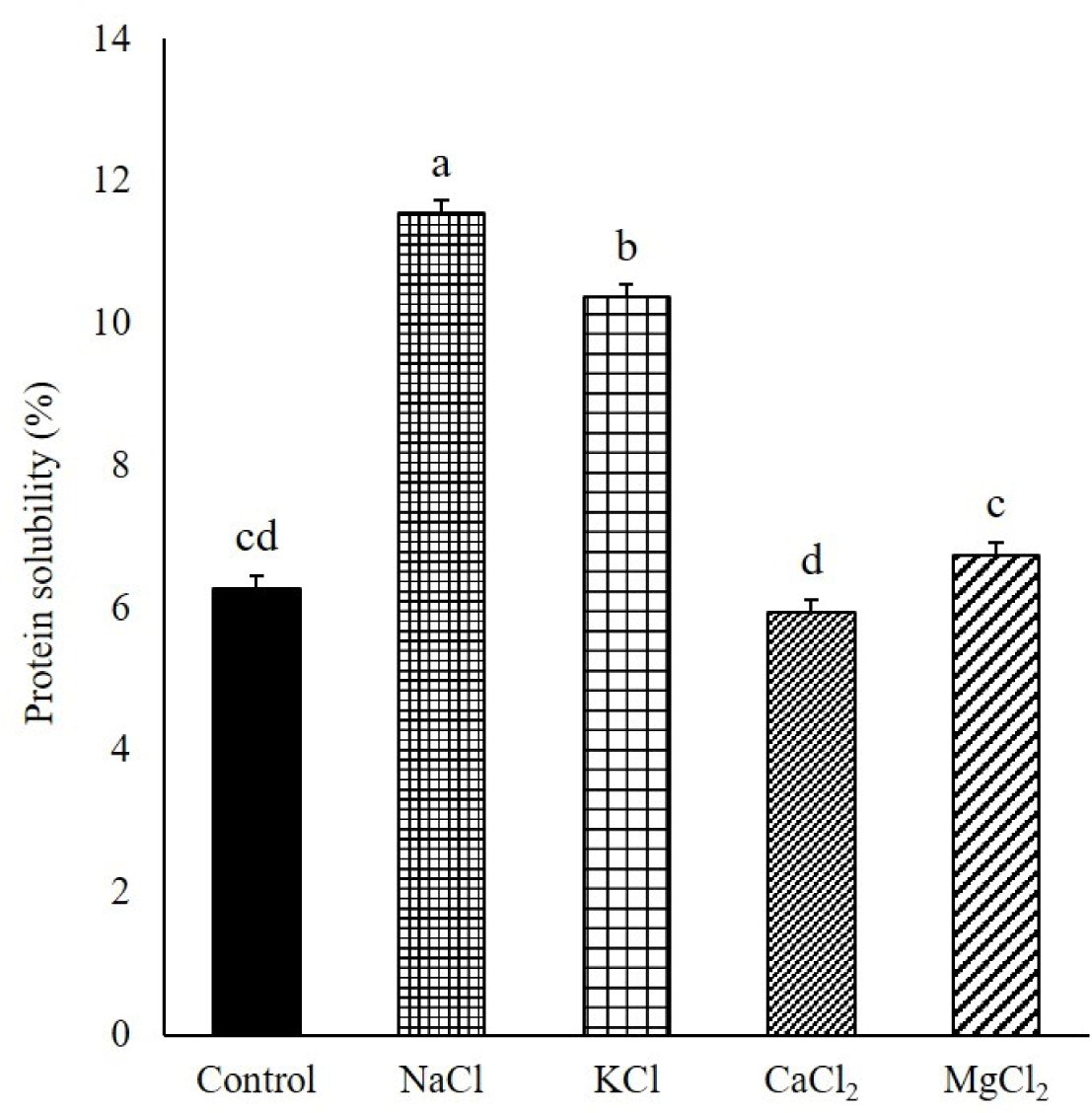
In this study, the MPs were homogenized with sodium pyrophosphate (0.3%) before mixing with chloride salts. Therefore, myosin heavy chain (MHC, approximately 200 kDa), actin (approximately 46 kDa), tropomyosin, and myosin light chain-1 were identified during the SDS–PAGE of the control because of the dissociation of actomyosin by phosphate (Fig. 2) [22]. Unlike the control, c-protein was found in the MPs solubilized with chloride salts. The c-protein is a constituent element of myosin filaments (myosin oligomers). Therefore, the solubilization of the c-protein indicated the dissociation of myosin oligomers to myosin monomers. Myosin oligomers and monomers can form gels; however, their gel properties are different [23]. The addition of NaCl and KCl to the MPs resulted in an increase in MHC intensity compared to that of the control, and myosin was the predominant protein among the solubilized MPs. Munasinghe and Sakai [24] confirmed that the extraction of myosin increased when NaCl or KCl was added to lean pork meat as a protein extractant. The protein profiles of the solubilized MPs changed with divalent salts (Fig. 2). The band intensity of MHC was decreased in the solubilized MPs with CaCl2 and MgCl2, and the decrease was severe with Mg2+, compared to that of the monovalent salts. In this study, the solubility of MPs did not change after the addition of CaCl2 and MgCl2 compared with the control. Therefore, this result indicated that the solubilized myosin with phosphate (control) might be aggregated and partially insolubilized with Ca2+ and Mg2+. The predominant protein in the MPs solubilized by CaCl2 was tropomyosin, which was different from that of other chloride salts. The solubilization of tropomyosin by CaCl2 may be caused by the selective binding of Ca2+ to troponin, which is connected to tropomyosin on the surface of actin filaments [25]. In this study, troponin C and I were detected in the MPs solubilized with CaCl2. Additionally, the selective binding of Ca2+ to troponin reduced the Ca2+ that could aggregate myosin; therefore, the band intensity of MHC was higher than that with Mg2+. Although the MHC band was faintly visible, other predominant protein bands, compared to other treatments, did not appear on the SDS–PAGE of MgCl2. This result might imply that the MPs solubilized by MgCl2 contained the solubilized myosin oligomer that could not undergo the SDS–PAGE because of its large molecular weight. Zhu and Damodaran [20] reported that protein aggregation might be caused by the cross-linking of proteins using the ionic bridges of divalent cations or hydrophobic interactions with an increase in the hydrophobicity of the protein via the strong binding of divalent cations. The protein profiles of MPs solubilized with MgCl2, except for myosin, were similar to those solubilized with NaCl and KCl. However, the surface hydrophobicity of the MPs solubilized with MgCl2 was significantly lower than that of the MPs solubilized with NaCl and KCl (Fig. 3). Therefore, Mg2+ aggregated myosin by hydrophobic interactions between myosin, and Ca2+ had a similar effect on myosin. Additionally, the relative band intensities of the MHC of the insolubilized MPs in the control, NaCl, KCl, CaCl2, and MgCl2 were 22.12, 15.69, 16.26, 24.54, and 21.39, respectively (Fig. 4). The band intensity of the MHC of NaCl and KCl was lower than that of the other treatments (p < 0.05). However, MgCl2 and CaCl2 showed a similar band intensity of MHC to the control despite the lower band intensity of MHC in solubilized MPs compared to the control (p > 0.05). This result was due to the formation of large molecular aggregates of MHC by MgCl2 and CaCl2 that could not undergo the SDS–PAGE. The results showed that the monovalent cation salts, NaCl and KCl, increased the solubility of myosin; however, the divalent cation salts, CaCl2 and MgCl2, did not. Rather, the divalent cation salt precipitated the myosin solubilized by phosphate.
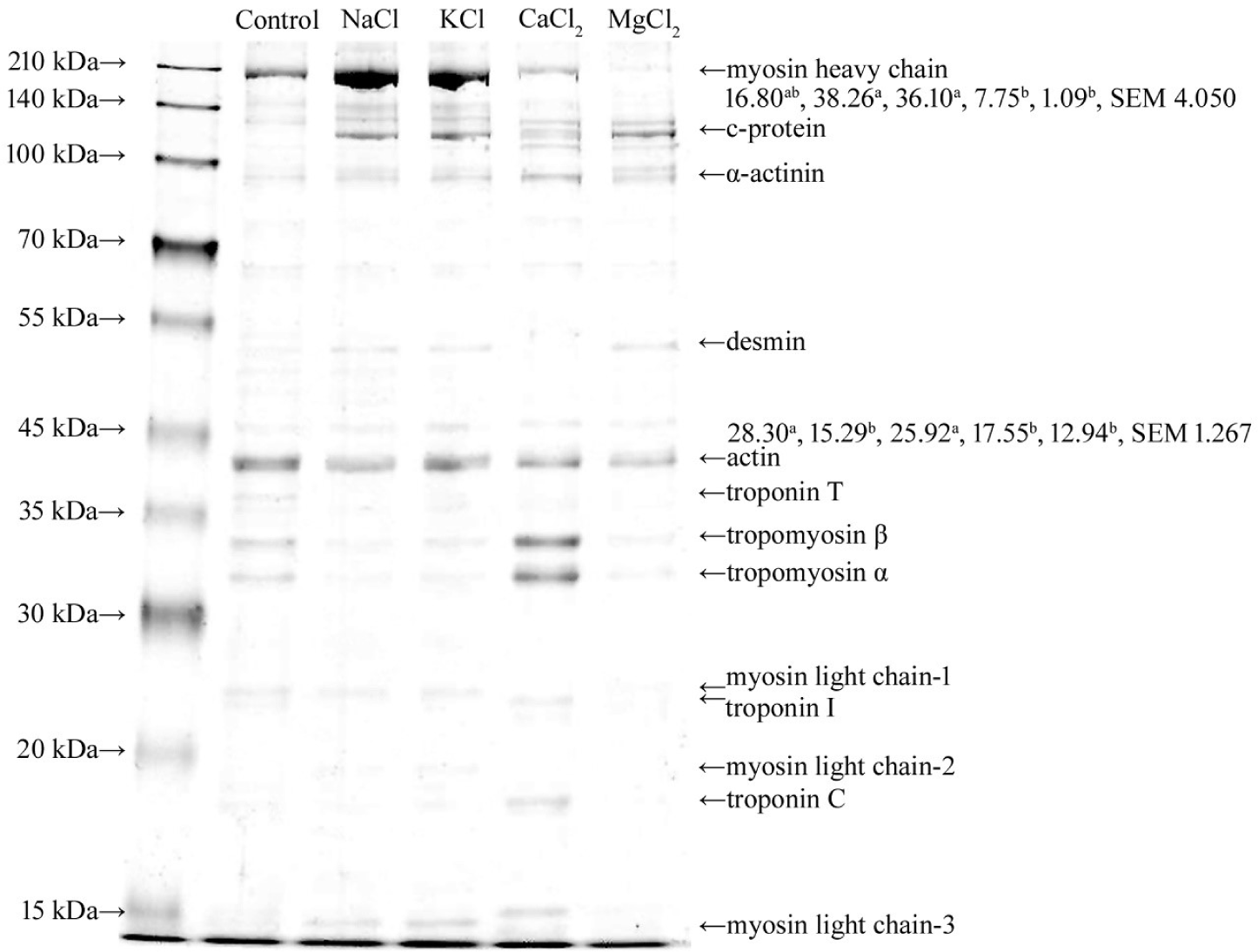
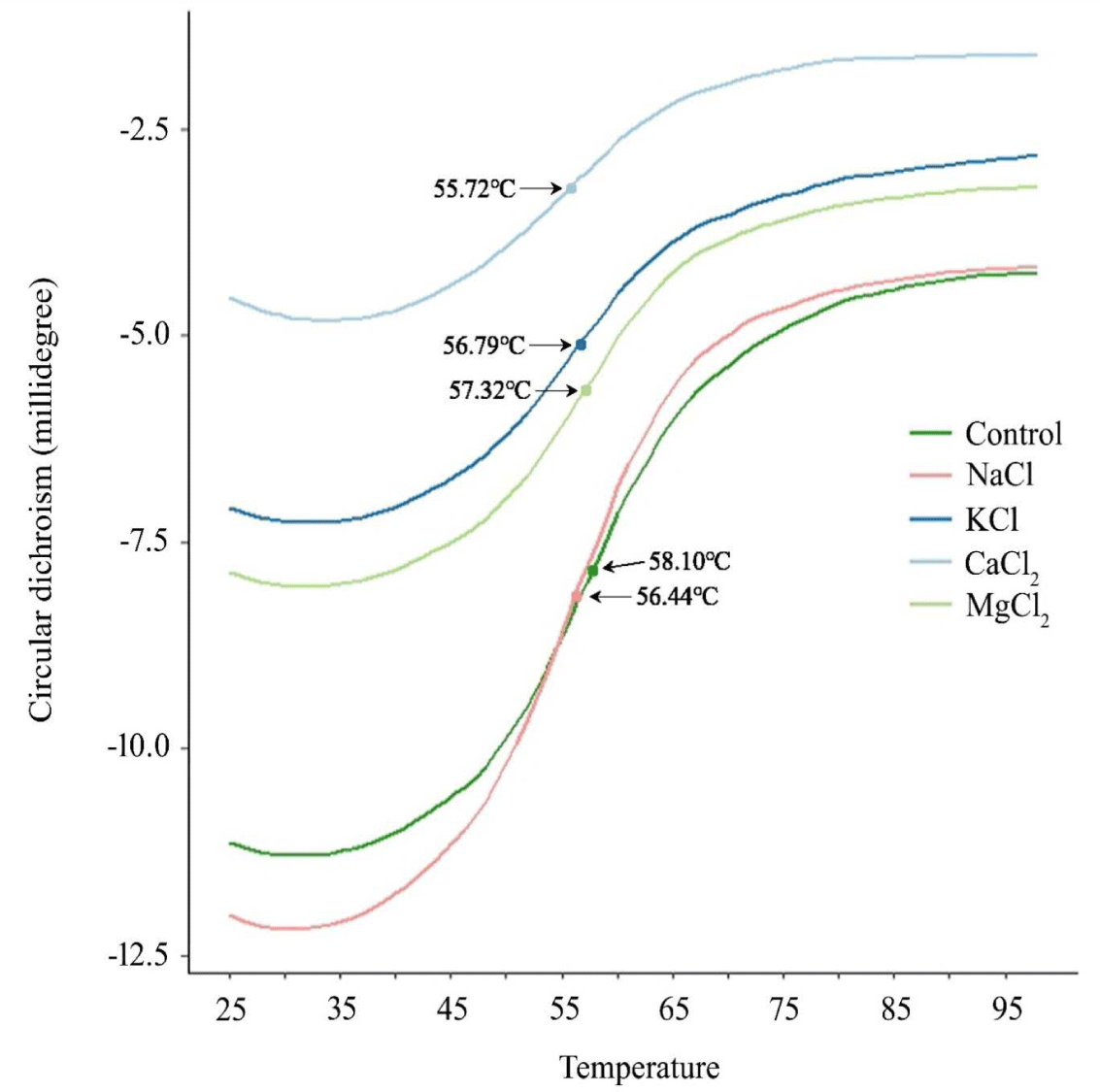
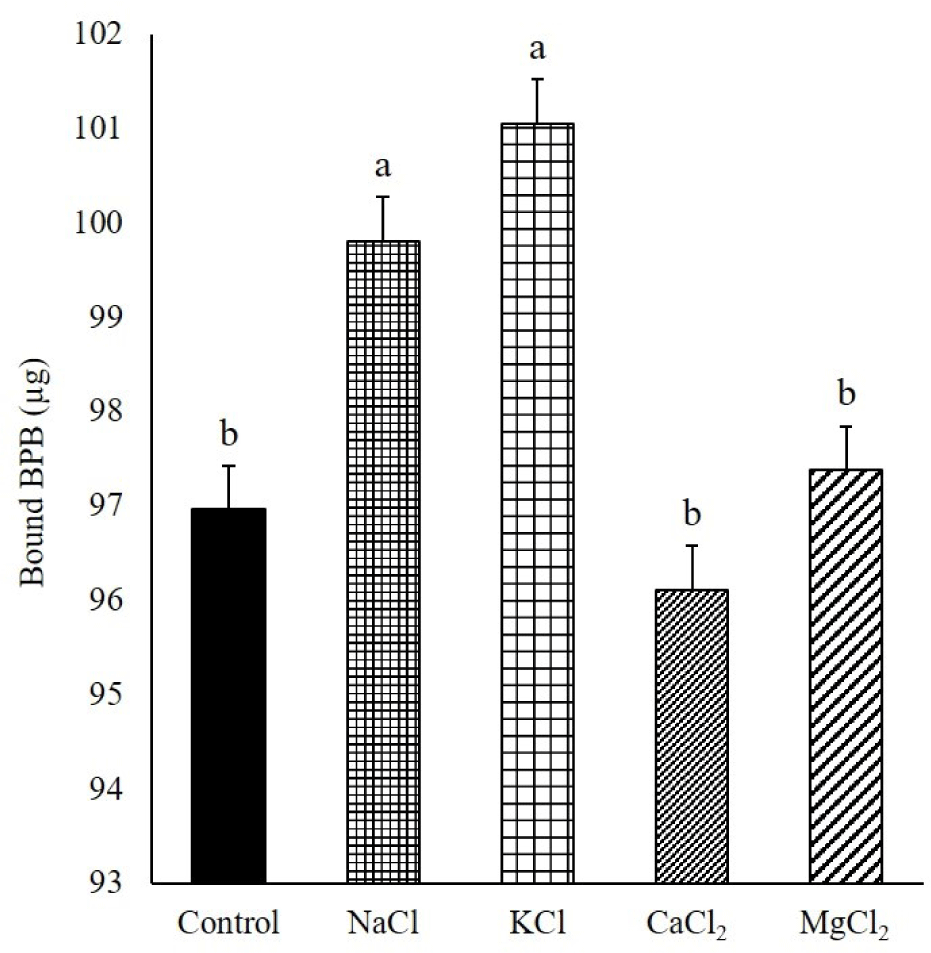
Proteins can bind to various metal ions, resulting in conformational changes in the protein. The secondary structures, such as the α-helix, β-sheets, β-turns, and random coils, of the myosin extracted with chloride salts, except for CaCl2, were not different from those of the control (Table 1). However, the α-helix content of the myosin extracted with KCl, MgCl2, and CaCl2 was significantly lower than that of the myosin extracted with NaCl; in contrast, other secondary structures, such as β-sheets, β-turns, and random coils were relatively higher in the myosin extracted with KCl, MgCl2, and CaCl2 than in the myosin extracted with NaCl (p < 0.05).
1) Control, myosin extract of myofibrillar protein extract with 0.3% sodium pyrophosphate; NaCl, myosin extract of myofibrillar protein extract with 0.3% sodium pyrophosphate and 2% NaCl; KCl, myosin extract of myofibrillar protein extract with 0.3% sodium pyrophosphate and 2.55% KCl; CaCl2, myosin extract of myofibrillar protein extract with 0.3% sodium pyrophosphate and 1.27% CaCl2; MgCl2, myosin extract of myofibrillar protein extract with 0.3% sodium pyrophosphate and 1.08% MgCl2.
The cations of KCl, MgCl2, and CaCl2 can be bound to proteins by interactions with the carbonyl oxygen and nitrogen of the backbone and the carbonyl oxygen, carboxylate anion, aromatic ring, etc., of the side chain [26,27]. The monovalent metal ions have a lower binding affinity and preference for specific amino acids of proteins than the divalent metal ions [28]. Additionally, there are differences in the binding affinity and preference for amino acids between Na+ and K+ [26,27]. A previous study found that the binding affinity of Na+ on the surface of proteins was approximately two times higher than that of K+ in all tested proteins. This difference was mainly caused by the high binding affinity of Na+ to the charged carboxylic (COO−) groups within the side chains of aspartic acid and glutamic acid [27]. Additionally, Heaton and Armentrout [26] reported that the bond distance between metal ions and ligands was longer in K+ than in Na+, and the stronger binding of Na+ led to the geometric distortions of the neutral ligands for the stable binding conformers. Therefore, the binding of K+ on the surface of myosin might not favor the α-helix conformation compared to the binding of Na+; consequently, the helix–coil and α–β transitions are generated.
Mg2+ and Ca2+ have higher binding affinities and selectivity for amino acids than those of Na+ and K+ when they interact with proteins [28]. Mg2+ and Ca2+ are mainly bound to aspartic acid and glutamic acid in proteins [28]. Additionally, two or more amino acids act as ligands to maximize the binding efficiency of divalent metal ions, unlike monovalent metal ions. Glutamic acid is the most abundant amino acid in myosin and is approximately twice as abundant in α-helix than in β-sheets [29]. Therefore, the binding of Mg2+ and Ca2+ to myosin may lead to more conformational changes, particularly in the α-helix structure. Additionally, the myosin extracted with CaCl2 had the lowest α-helix and the highest β-sheet, β-turn, and random coil contents among the treatments. A previous study reported that the addition of calcium unwound the myosin head in the thick filament, and consequently, the α-helix content was reduced [30].
The decrease in the α-helix and the increase in the β-sheet, β-turn, and random coil contents of myosin secondary structure could indicate an increase in the degree of disorder of the myosin structure. The change in the myosin structure to a disordered structure is a general phenomenon associated with the heat denaturation of myosin [31]. A previous study reported decreasing α-helix and increasing β-sheet, β-turn, and random coil contents within the myosin after heating [32]. Further, this phenomenon was observed in this study. The α-helix and β-sheet of myosin heated up to 98°C were decreased and increased, respectively, compared to those at 25°C (data not shown). From these results, it can be observed that KCl, MgCl2, and CaCl2 disordered the structure of myosin, like denatured myosin, compared to NaCl.
Conformational changes in protein structure could change the heat denaturation temperature (Td), and increased denaturation rate indicates the reduced thermostability of the protein. The Td is the temperature of two-state transition from native to unfolded states [16]. Shimada et al. [16] found that below the Td, the soluble fraction contained only monomeric myosin, and above the apparent Td, aggregates were formed through the unfolding of myosin monomers, head–head interaction, and tail–tail cross-linking. The CD signal of the extracted myosin at 222 nm increased from approximately 35 to 70°C and plateaued with an increase in temperature until the end temperature in all treatments (Fig. 5). The Td values of the control group, NaCl, KCl, MgCl2, and CaCl2 were 58.10, 56.44, 56.99, 57.32, and 55.72°C, respectively, and there was no significant difference among the treatments (p > 0.05). In a previous study, an increase in the transition temperature due to stabilization through molecule-to-filament association [33] and an increase in thermal stability accompanied by an increase in random coils were reported [12]. However, in this experiment, the change in the secondary structure did not significantly affect the thermal sensitivity.
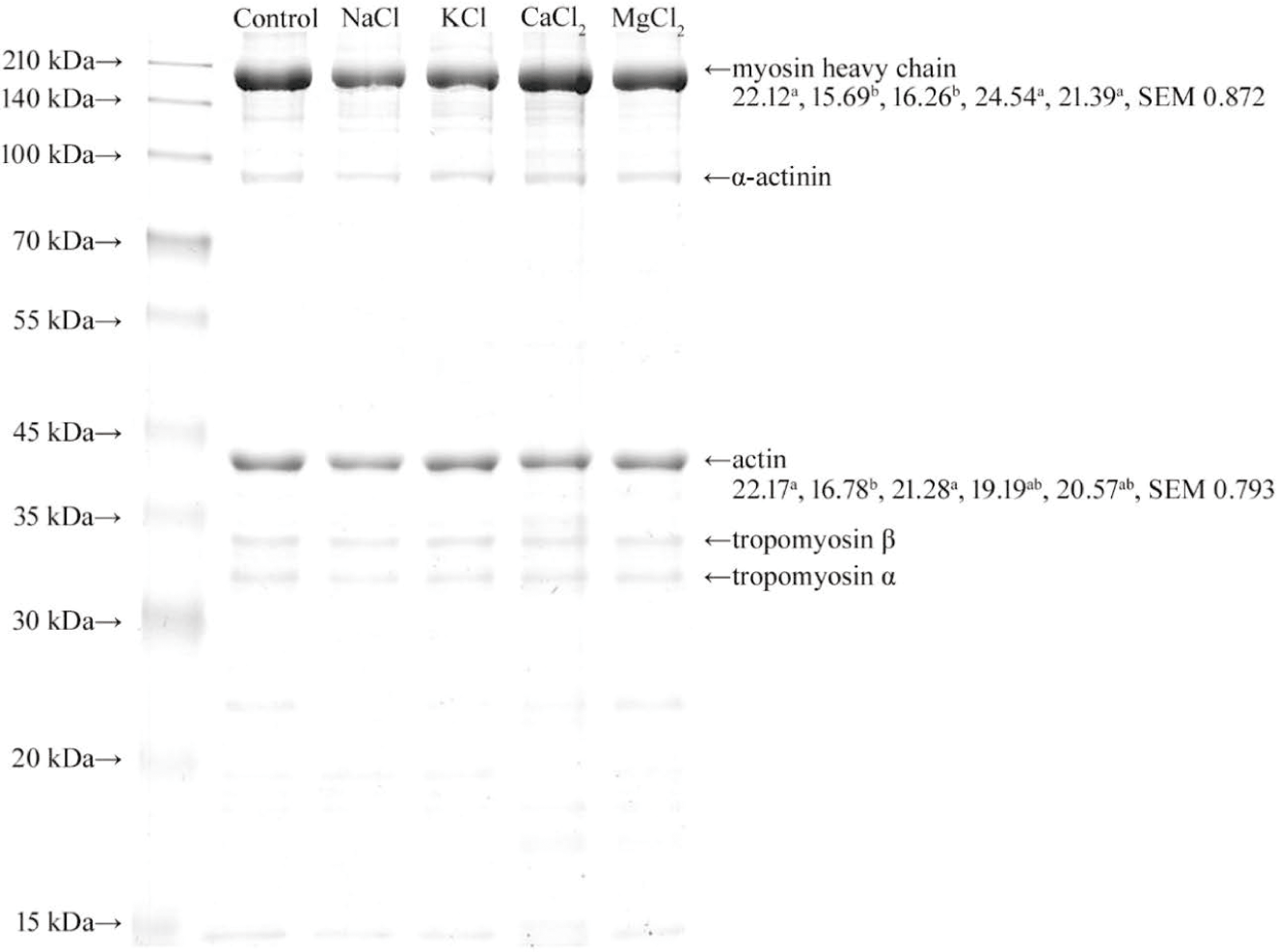
In this study, chloride salts, such as NaCl, KCl, MgCl2, and CaCl2, showed different effects on myosin solubility and secondary structures because of their different cations. KCl showed a similar solubility to that of NaCl; however, the secondary structure of myosin was different between KCl and NaCl. The divalent metal salts, such as MgCl2 and CaCl2, exhibited a lower ability to solubilize myosin than that exhibited by the monovalent metal salts, and the myosin solubility was the lowest in CaCl2. Additionally, MgCl2 and CaCl2 changed the secondary structure of myosin compared with NaCl.
The pH of meat batter is a factor that affects protein solubility and water-holding capacity; therefore, high pH can contribute to the formation of a stable gel with a desirable texture [34]. The pH of the meat batter was significantly different depending on the type of salt added (Fig. 6A). The control had the highest pH, followed by KCl, NaCl, MgCl2, and CaCl2 (p < 0.05). These results are consistent with those of previous studies. Terrell et al. [35] reported that a significant increase and decrease in pH was observed in KCl and divalent salt groups, respectively, compared to NaCl, when NaCl, KCl, CaCl2, and MgCl2 were used for salting beef clods at the same ionic strength. Similarly, Gordon and Barbut [9] reported that CaCl2 and MgCl2 reduced the pH of raw meat batter compared with NaCl at the same ionic strength. The decrease in pH in the meat batter may be related to the binding affinity of the cations of the added salt on the protein, particularly the negatively charged side chains of aspartic acid and glutamic acid. In this study, the binding affinity of the cations of the added salt on the negatively charged side chains of the protein increased in the order of K+, Na+, Mg2+, and Ca2+ [26,27]. Additionally, according to the Hofmeister series, the more chaotropic the ions, the more strongly hydrated the ions, and they strongly bind to the negatively charged aqueous carboxyl group [17]. As described above, among K+, Na+, Mg2+, and Ca2+, Ca2+ is the most chaotropic ion. The binding of the cations with the negatively charged side chain in protein molecules established a competitive relationship with hydrogen ions, which can bind to the carboxyl group of amino acids. Therefore, the hydrogen ions that cannot be bound because of cation float in the system and decrease the pH. A relatively low pH is more likely to degrade the quality of the product by decreasing the negative net charges on the surface of protein molecules. It can increase protein–protein interactions and reduce protein solubility.
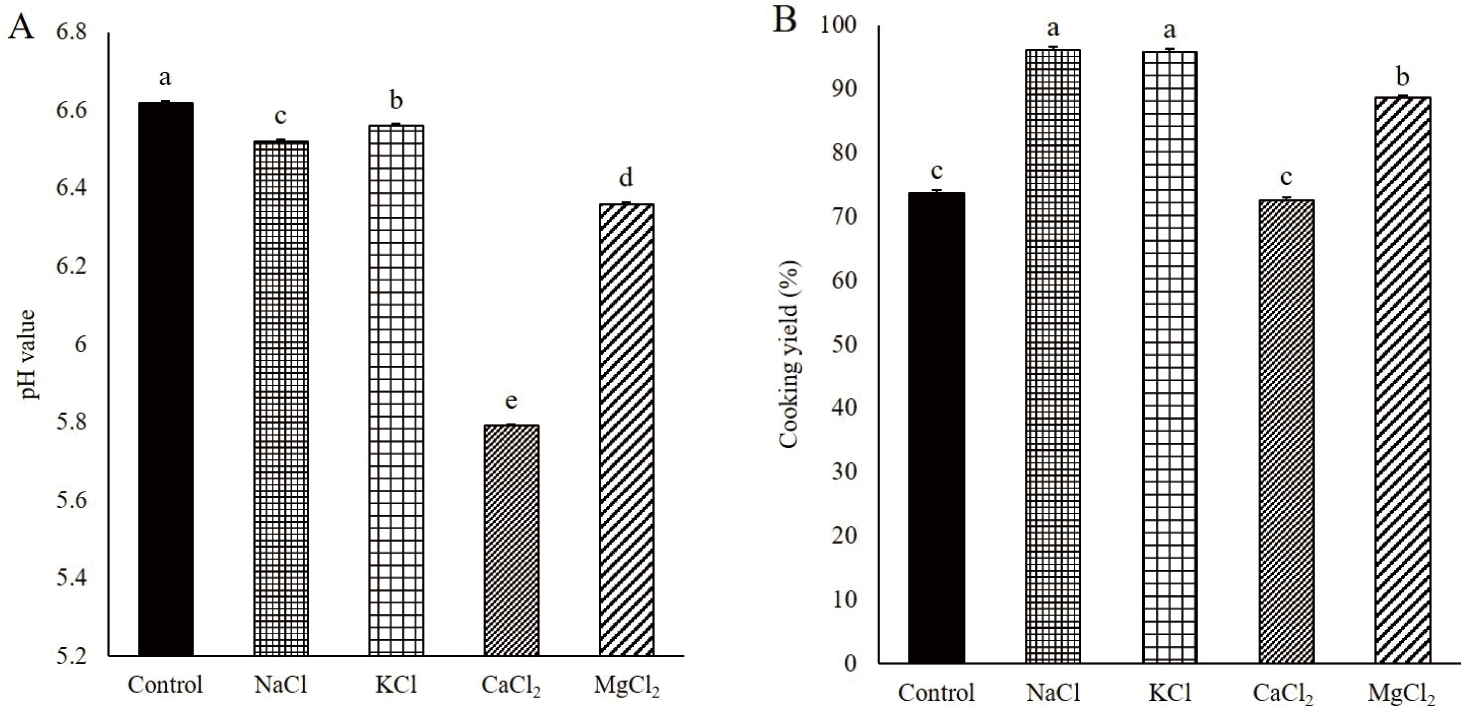
The water in the meat batter is physiochemically held in the coagulated protein gel upon heating. The low CY due to the high release of water with the low water-holding capacity of meat gel results in a low yield with poor quality due to hard texture, low juiciness, and loss of nutrients, such as proteins, vitamins, and minerals, in meat [36]. The water-holding capacity of meat gel is affected by the pH and the amount of solubilized myosin in the meat batter [1,8].
The CY of gels manufactured with NaCl and KCl was significantly higher than that of the control, CaCl2, and MgCl2 gels (Fig. 6B, p < 0.05). This result was attributed to the highly solubilized myosin in the meat batter with NaCl and KCl compared to other treatments. The solubilized myosin in the meat batter formed an aggregated-type gel with high water-holding capacity, while the myosin filament with low solubilization of myosin formed a strand-type gel with low water-holding capacity [23]. The divalent salts, CaCl2 and MgCl2, showed low CY, and the lowest CY was found in the meat gel with CaCl2. The meat batter with CaCl2 and MgCl2 contained low solubilized myosin, and therefore, it could not form a gel to effectively hold water. Additionally, there might be few spaces to hold water in the gel with CaCl2 and MgCl2 because of the low pH and the aggregation of myosin by salt bridges. The low pH of the meat batter near the isoelectric point of MPs results in a decrease in the repulsive forces between proteins [5]. Therefore, the gel is formed with closely aggregated proteins at low pH and salt bridges between the proteins. In this study, CaCl2 had the lowest ability to solubilize MPs, including myosin, and its addition resulted in the lowest pH of the meat batter. Therefore, the CY of the gel manufactured with CaCl2 was the lowest, and this was not significantly different from that of the control, which contained no chloride salts (p > 0.05). In addition to the effects of pH, solubilized proteins, and salt bridges between proteins, the secondary structure of myosin might be a factor in the water-holding capacity of the pork gel. Barlow and Poole [37] reported that the interaction of water molecules with the carbonyl oxygen of the protein backbone and side chains was higher in α-helix than in β-sheets because of the differences in the geometry of the water–CO group interaction. In this study, the α-helix and β-sheet contents of myosin were lower and higher, respectively, in CaCl2 and MgCl2 than in NaCl.
KCl had a lower MPs solubility than that of NaCl. Additionally, the α-helix and β-sheet contents of myosin extracted by KCl were lower and higher, respectively, than those of myosin extracted by NaCl. However, the CY of the pork gel was not significantly different between KCl and NaCl (p > 0.05). The low water-holding capacity of the pork gel with KCl in terms of low protein solubility and the secondary structure of myosin might be compensated by the higher pH of the meat batter than that of NaCl.
The hardness of the pork gel was significantly higher in the NaCl and CaCl2 treatments than in the control, KCl, and MgCl2 treatments (p < 0.05, Fig. 7). This result was consistent with a previous study, in which the hardness of frankfurters manufactured with NaCl was the same as that of frankfurters manufactured with CaCl2 and higher than those of frankfurters manufactured with KCl and MgCl2 at the same ionic strength [8].
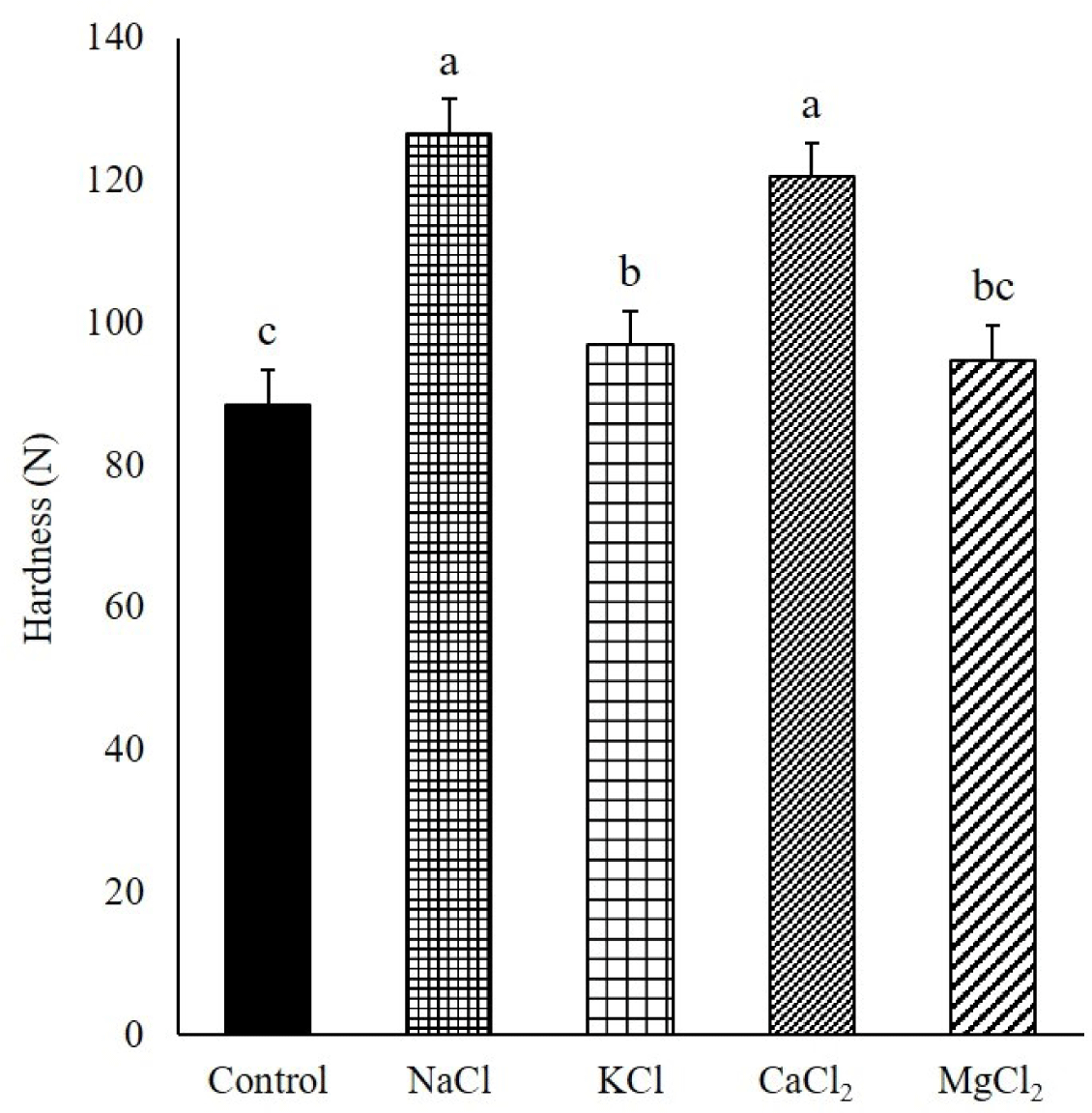
Gordon and Barbut [8] reported that the hardness of the frankfurters was affected by the different types and amounts of solubilized proteins. Therefore, the lower hardness of pork gel in KCl than in NaCl was attributed to the relatively low MPs solubility, although the profiles of the solubilized MPs were similar between NaCl and KCl in this study. Additionally, the α-helix content of myosin with KCl was lower than that of myosin with NaCl in this study. A previous study reported that the transition of α-helix to β-sheet, β-turn, and random coil was a general phenomenon in gelation with the formation of protein networks by heating, and the β-sheet structure formed during gelation was the major factor affecting gel strength [38]. Therefore, the transition of α-helix to β-sheet of myosin during gelation in KCl might be lower than that in NaCl because of the pre-transition of α-helix to β-sheet before heating, affording the low hardness of pork gel with weak protein networks, compared to that of NaCl [11].
Although CaCl2 showed the lowest MPs solubility, including less solubilized monomeric myosin, the hardness of the pork gel with CaCl2 was similar to that of the pork gel with NaCl. The high hardness of CaCl2 could be due to the formation of a woven gel with a tightened structure by closely aggregated proteins holding low amounts of water (Fig. 6B) [9].
A previous study found that the pork MPs gel with NaCl exhibited a regular and ordered cross-linked structure because of the aggregation of monomeric myosin [39]. However, a disordered and irregular structure of the gel was formed because of the coarse cross-linking of fibrous filaments when the pork gel was prepared with the partial substitution of NaCl by a mixture of KCl and MgCl2 or CaCl2, and the gel strength was affected by the gel structure [39]. In this study, KCl, MgCl2, and CaCl2 caused changes in the secondary structure of myosin compared with NaCl, and these changes resulted in an increase in the degree of disorder in the myosin structure by the transition of ordered α-helix into disordered β-turn and random coil. Therefore, it might be considered that the disordered and irregular gel structures found in the gel with KCl, MgCl2, and CaCl2 were related to the degree of disorder in the myosin structure.
CONCLUSION
NaCl, KCl, CaCl2, and MgCl2 exhibited differences in the solubility of MPs, including myosin, and the structure of myosin. The solubility of the MPs was higher in NaCl than in KCl, and the solubility of divalent salts was lower than that of monovalent salts. KCl, CaCl2, and MgCl2 decreased the α-helix content compared with NaCl. From the differences in the solubility and the structure of myosin, the CY of the gel manufactured with monovalent salts was significantly higher than that of the gel manufactured with divalent salts, and the hardness of the pork gel was lower with KCl than with NaCl. Therefore, the changes in the solubility and the structure of myosin with the different chloride salts led the different quality properties of pork gel. The results of this study can be helpful for understanding the quality properties of low-salt meat products manufactured by replacing sodium chloride with different chloride salts.