INTRODUCTION
Constipation, a common gastrointestinal disorder characterized by difficulty in passing stool, infrequent bowel movements, and hard or dry stools, has significant implications for the health and well-being of livestock [1]. Dairy cows experiencing constipation develop ruminal acidosis, which is characterized by an overproduction of acid in the rumen, resulting in decreased milk production and quality, as well as lameness and reduced fertility [2]. In pigs, constipation increases the risk of constipation-related diseases, including rectal prolapse, hernias, and urinary tract infections, ultimately leading to decreased feed intake and weight gain, negatively impacting production [3,4]. In chickens, constipation results in the buildup of fecal matter in the cloaca, causing bacterial infections and egg contamination, affecting egg production and quality [5,6]. Various factors can cause constipation in livestock, including inadequate fiber intake, dehydration, lack of mobility and movement during confinement, hormones and antibiotics, and underlying medical conditions [7].
Moreover, constipation is a significant problem among aging livestock, with various impacts on animal health, productivity, and welfare [8]. The reduced feed intake caused by constipation among aging livestock ultimately leads to lower weight gain, productivity, and economic gains for farmers and the livestock industry [9]. Additionally, constipation increases the risk of gastrointestinal diseases such as colic among aging livestock, which negatively impacts health and productivity, resulting in expensive veterinary treatments [10]. Constipation also causes significant discomfort and pain for livestock, leading to changes in their welfare and behaviour, such as discomfort, restlessness, or irritability, which further exacerbate the problem [11]. Furthermore, constipation impacts the absorption of nutrients in the gut, leading to nutritional deficiencies in aging livestock, which negatively impacts lifespans and mortality [12].
Constipation is a prevalent gastrointestinal disorder in livestock, with variations in prevalence depending on the species, age, and management practices [13]. In dairy cattle, prevalence ranges from 2-17%, with higher rates in confined housing systems and during heat stress [14]. In pigs, constipation is more significant in weaned piglets, ranging from 2%–28%, with higher rates in low-fiber diets and increasing with aging [15]. Aging also increases the prevalence of constipation in dairy cows, with rates as high as 21% in cows over 4 years old, and in sows, with rates as high as 30% in sows over 3 years old [16,17].
Lactic acid bacteria (LAB) are a group of bacteria that are commonly found in the gastrointestinal tract, as well as in many fermented foods such as yogurt, kefir, and sauerkraut [18]. Strains of LAB have been shown to have probiotic properties, with the potential to confer various benefits to the hosts varying from gut health to immunity enhancement [19,20]. LAB strains from various isolation sources have been documented to improved digestive health, enhance immune system, reduced risk of gut diseases such as inflammatory bowel disease, colorectal cancer, reducing hypertension and allergies [21,22]. Past studies have shown that certain strains of LAB are beneficial in alleviating constipation both in humans and livestock [23]. LAB strains that have been studied for their potential to improve bowel function, alleviate constipation and modulate gut microbiota to improve the balance of bacteria in the gut, all leading to improved digestive function and regularity of bowel movements [24]. Gut metabolites from LAB strains have been shown to stimulate intestinal contractions and promote bowel movements [25]. However, the evidence on the effectiveness of LAB in alleviating constipation remains limited, and more research is needed to fully understand the potential benefits and mechanisms of action of these bacteria [26]. The effectiveness of LAB is also dependent on the host and specific strains used [27]. Recently, LAB strains have been reported to contribute towards healthy ageing, ranging from the preservation of gut health to maintenance of brain activities amid aging [28]. This has heightened a hypothesis that LAB strains may be beneficial towards constipation amid aging.
Loperamide is a medication used to treat diarrhea but can also induce constipation by slowing down intestinal motility [29]. By inducing constipation in rats, the condition of reduced gut motility and other age-related changes in the digestive system that can lead to constipation can be simulated [30]. Although rats cannot directly replace livestock models due to differences in digestive systems and gastrointestinal anatomy, they provide valuable insights into the mechanisms of constipation and potential treatment options [31]. Rats are often used as a model for initial testing of potential treatments before testing in livestock or other larger animal models, given their accessibility, easy housing, and controlled and tractable system for studying various aspects of animal physiology, nutrition, or disease relevant to livestock [32,33].
The present study aims to evaluate the modulatory effect of dairy-based LAB administration on laxative attributes using rats. This includes observing changes in the fecal profile, gut metabolites, gastrointestinal motility, and intestinal morphology upon LAB administration. The results of this study could have implications for the potential use of LAB as a treatment for constipation in livestock, with the hope of improving the health and quality of livestock production.
MATERIALS AND METHODS
Strains of Limosilactobacillus fermentum USM 4189 (L. fermentum USM 4189) and Lactiplantibacillus plantarum USM 4187 (L. plantarum USM 4187) were isolated from milk of cows from Penang, Malaysia. Stock cultures were preserved in 20% glycerol at −20°C. Each strain was grown and activated in sterile Man-Rogosa-Sharpe (MRS) broth (Biomark, Maharashtra, India) for three successive times using 10% (v/v) inoculum, incubated at 37°C in an aerobic atmosphere without agitation prior to use.
All animal experiments were approved by the USM Animal Care and Use Committee (USM/Animal Ethics Approval/724) and were carried out under Good Laboratory Practice (GLP) condition and facility (Animal Research and Service Centre, USM Advanced Medical and Dental Institute) according to the National Institutes of Health (NIH) Public Health Service Policy. Male Sprague–Dawley rats at 8 weeks of age were used in this study. Animals were housed with alternating 12 hrs light and dark cycles with free access to regular chow (Altromin, Lage, Germany). Food intake and body weight were recorded once a week. The adverse events (including signs of illness or mortality) of animals were monitored daily, and no adverse effects were observed throughout the study.
Upon a week of acclimatization to their environmental enrichment, the rats were divided into six groups according to their body weight, and each group was randomly assigned to one of the experimental groups (n = 6) as follows: (1) Young rat as naïve group; where animals were not subjected to any induction or intervention, (2) Aged rat; D-galactose induced aging as the negative control group, (3) Aged-constipated rat; D-galactose induced aging and loperamide induced constipation as the negative control group, (4) Aged-constipated-laxative rat; D-galactose induced aging and loperamide induced constipation treated with laxative as the positive control group, (5) Aged-constipated-4189; D-galactose induced aging and loperamide induced constipation treated with L. fermentum 4189, and (6) Aged-constipated-4187; D-galactose induced aging and loperamide induced constipation treated with L. plantarum 4187.
D-galactose (D-gal) was used to induce premature aging in rats [34]. All experimental groups received 600 mg/body weight (kg) D-gal (Sigma Aldrich, St. Louis, MO, USA) prepared using 0.9% sterile saline via subcutaneous injection daily except for the naïve group (young rat), which received only 0.9% sterile saline. Constipation was induced in rats through intragastric administration of loperamide daily. All experimental groups received 5 mg/body weight (kg) loperamide hydrochloride (Sigma Aldrich) via oral gavage using stainless steel, 21-gauge round-tip feeding needle. The naïve group (young rat) and aged rat received only 0.9% sterile saline. The laxative group as a positive control group received loperamide-induced constipation in the same way but treated daily with a commercial laxative. Lactulose syrup was administered through oral gavage using stainless steel, 21-gauge round-tip feeding needle with a standard dosage of 1 g/body weight (kg). Probiotic treatment groups received 1 ×10 Log CFU/day live L. fermentum 4189 or L. plantarum 4187 cells, daily via oral gavage.
The experimental animals were given ad libitum access to cereal-based, 10 mm pellets standard maintenance diet for rats made of mainly soy, wheat, and corn (Altromin, Lage, Germany) and filtered water. Body weight (g) and feed intake (g) were measured weakly where changes in experimental animal body weight (g) and feed efficiency (%) were observed across 12 weeks of treatment, calculated as the ratio of body weight gain (g) over the amount of feed intake (g) × 100 [35]. Animal were maintained in a 0.086 m2 (floor area) transparent polycarbonate cage with stainless steel wire bar led, cage dimensions were; 48.26 cm, long × 25.4 cm, wide × 20.32 cm, deep. Corncob bedding (Biocob, Biosys, Singapore) substrate was used with schedule cleaning twice per week on Monday and Thursday. Humidity and room temperature regulated (55 ± 10%; 21 ± 2°C), 12-hours light/dark cycle with lights off at 19:00 h and no twilight period. After 12 weeks of experimental periods, animals were sacrificed by means of carbon dioxide inhalation inside a transparent polycarbonate euthanasia chamber after 12 hours of fasting, until beyond visible cessation of breathing.
Trunk blood was collected by cardiac puncture into clot activator tube, K2EDTA tube, and sodium fluoride tube for subsequence analysis. All blood samples were kept at 4°C and processed within 24hrs after collection. The whole blood samples in clot activated tube were used for blood serum biochemical analysis in an MS ISO 1589 certified Advance Diagnosis laboratory (Advanced Medical and Dental Institute, USM, Malaysia). The blood sample was centrifuged at 1,500×g at 4°C for 15 min to separate the serum and immediately collected into a new tube. Blood serum was analyzed within 48hrs after extraction, using an auto-analyzer AU5822 (Beckman Coulter, Brea, CA, USA) according to the protocol recommended by International Federation for Clinical Chemistry (IFCC). Liver function profile (albumin, total protein, globulin, albumin/globulin ratio [A/G], alanine aminotransferase [ALT], alkaline phosphatase [ALP] and aspartate aminotransferase [AST]), renal function profile (calcium, chloride, creatinine, phosphate, potassium, sodium, urea and uric acid) and fasting blood sugar was measured.
An aliquot of 100 μL whole blood, collected in K2EDTA tube from each individual rat was used for genomic DNA extraction. Firstly, blood samples were lysed with 250 μL red blood cell (RBC) Lysis Buffer (1 mM ethylenediaminetetraacetic acid [EDTA=, pH 8.0), vortexed, and incubated at 25°C for 2 min. The samples were centrifuged at 8,000×g at 4°C for 5 min. The supernatant was discarded, then the pellet was lysed again with RBC Lysis Buffer, vortexed, and followed by centrifugation at 8,000×g at 4°C for 5 min. The white pellet was resuspended in 250 μL of 1 M sodium chloride solution, vortexed vigorously then added with 500 μL of Cell Lysis Buffer (26 mM EDTA, 17.3 mM of 0.5% sodium dodecyl sulfate [SDS], 10 mM Tris-HCl, pH 7.3) and 2 μL of 4 mg/mL RNase A solution. The mixture was vortexed then incubated at 37°C for 60 mins to allow complete rupture of blood cell and degradation of RNA, followed by the addition of 200 μL of 3M sodium acetate solution. The sample was mixed properly then 200 μL of phenol-chloroform-isoamyl alcohol (25:24:1 v/v; Sigma Aldrich, USA) was added, vortexed, and followed by centrifugation at 10,000×g at 25°C for 10 mins. Approximately, 700 μL of the clear upper layer containing DNA material was collected into a new tube and added with 700 μL of 100% isopropanol. The mixture was then inverted 25 times to facilitate DNA precipitation and incubated overnight at 4°C, followed by centrifugation at 10,000×g at 25°C for 5 mins. The supernatant was discarded, DNA pellet was washed twice with 1 mL 70% (v/v) ethanol and centrifuged at 8,000×g at 25°C for 2 mins. Finally, the DNA pellet was air-dried in the laminar flow cabinet for 5 to 15 mins and resuspended in 200 μL of Tris-EDTA (TE) buffer.
Telomere length quantification of genomic DNA extracted from whole blood using the quantitative polymerase chain reaction (qPCR) method was conducted as previously described [36] with minor modification. A total volume of 25 μL PCR reaction cocktail was prepared, containing 10.5 μL of 20 ng DNA, 12.5 μL of SensiFAST SYBR® mix (2 x; Bioline, London, UK), 1 μL of both 22.5 μM single-copy gene (SCG) and telomere primers. The primer sequence for SCG (albumin) and telomere is as listed in (Table 1).
The thermal cycling profile for telomere measurement was as follows: Stage 1: 1 cycle at 95°C for 3 mins; Stage 2: 2 cycle at 94°C for 15s and 49°C for 15s; and Stage 3: 40 cycle at 94°C for 15s, 84°C for 10 s and 88°C for 15s for SCG with the signal acquisition or Stage 3: 40 cycle at 94°C for 15s, 62°C for 10 s and 74°C for 15s for telomere with the signal acquisition. Standard curves for both SCG (albumin) and telomere were constructed using reference DNA sample (the ‘Standard DNA’) with final DNA concentrations ranges from 0.073 ng/μL to 17.65 ng/μL. The telomere length for an experimental DNA sample is expressed as T/S ratio, where T represents the number of reference DNA (Standard DNA) in nanogram that matches the telomere template’s copy number of experiment sample and S represents the number of reference DNA (Standard DNA) in nanogram that matches the SCG’s (albumin) copy number of the experimental sample. The average T/S ratio is expected to be proportional to the average telomere length per cell.
Individual fecal samples from each rat were collected after 12 weeks of experimental periods. Four to five fresh fecal pellets were collected directly from each individual rat by attaching a sterile microcentrifuge tube to the animal’s anus. Fresh fecal pellet excreted from each rat was immediately weighted using an electrical balance, as fecal wet weight in (g). Then, fecal samples were lyophilized overnight and weighed again to determine the dry weight in (g) [37]. Fecal moisture content in percentage (%) was calculated as follows:
Rectal fecal samples from each experimental group were collected over the course of 24 h, after intragastric administration of probiotic treatment. Prior to fecal collection, the cage was mounted with stainless steel wire grid floor approximately 2.54 cm from the bottom of the cage. A freshly changed corncob bedding substrate was covered with absorbent paper under the wire grid to take up urine and spilled drinking water. Fecal excreted from each experimental group were collected using forceps into a polypropylene plastic container after removing most of the diet debris with a strainer. The fecal samples were arranged sequentially on a scaled board to assist the quantitative morphology appearance, in terms of fecal form and compared with the Bristol Stool Form Scale (BSF scale). The quantitative morphology appearance of these samples was rated on a 7-point scale, where all the observation and scoring were done by a single investigator [38,39].
Approximately 10–20 mg of lyophilized fecal samples were suspended in 90 μL of freshly made Milli-Q water, 10 μL of crotonic acid (2 mM) was added as the internal standard. The samples were vortexed to homogenize, then added with 50 μL HCL and 200 μL diethyl ether using an auto pipettor. The samples were homogenized again by vortex, and later centrifuged at 3,000×g; room temperature for 10 mins. The mixture will be separated into three layers, where the topmost layer consists of organic acids (approximately 80 μL) was transferred to a glass vial. Next, 16 μL of N-tert-Butyldimethylsilyl-N-methyltrifluoroacetamide (MTBSTFA; Sigma Aldrich) was added into each vial and sealed properly. The samples were incubated at 80°C for 20 mins, then left at 25°C for 48 hrs before gas chromatography-mass spectrometer (GC-MS) analysis. The standard mix of 20, 200, and 2,000 μM which includes acetate, butyrate, formate, hexanoate, isobutyrate, isovaletrate, lactate, propionate, succinate, valerate (pentanoate), as well as blank, were prepared in the same manner as the samples [40].
Approximately 5 – 10 mg of lyophilized fecal samples were added with 60 μL chloroform, 135 μL of freshly made Milli-Q water, 150 μL methanol, and 15 μL of 2-propyl maleric acid (1 mM) as the internal standard. The samples were vortexed to homogenize, then followed by 30 mins incubation at 37°C with agitation at 1,200 rpm. Subsequently, the samples were centrifuged at 16,000×g at 4°C for 5 mins. Approximately, 250 μL of the top aqueous layer were collected into a new tube, then added with 200 μL of freshly made Milli-Q water. The samples were vortexed to homogenize, then centrifuged at 16,000×g at 4°C for 5 mins. An aliquot of the supernatant, 250 μL was transferred to a screw-cap tube and dried at 40°C for 20 mins, then snapped frozen in liquid nitrogen. The frozen samples were lyophilized overnight and stored at −80°C until further analysis. Prior to GC-MS analysis, lyophilized samples were added with 40 μL methoxamine (20 mg/mL; Sigma Aldrich) in pyridine, followed by sonication for 20 mins. The mixture was incubated at 37°C for 90 mins with agitation at 1,200 rpm. Next, 20 μL of the silylation agent N-Methyl-N-(trimethylsilyl) trifluoroacetamide (MSTFA; GL Science, Tokyo, Japan) was added into each tube and incubated at 37°C for 30 mins with agitation. The samples were centrifuged at 16,000×g at 4°C for 5 mins. Finally, the supernatant was transferred to a glass vial and sealed properly [41].
The measurement of SCFA and water-soluble metabolites concentration in rat’s fecal sample were analyzed using 6890N Network GC System (Agilent Technologies, Santa Clara, CA, USA). The capillary column HP-5MS (0.25 mm × 30 m × 0.25 mm; fused silica) was used together with 5973 Network Mass Selective Detector (Agilent Technologies). The column oven was programmed as follows: 60°C held for 3 mins, 60°C–120°C (5°C per min), 120°C–300°C (20°C per min). Grade 5 Helium (99.999%) constantly flowing at 1.2 mL/min was used as the carrier gas, with 1 μL of sample injected into the system and ran for 30 mins. The concentration of the metabolites in the sample was quantified by comparing their peak areas with standards mix via LabSolution Insight software.
Carmine (C.I. 75470, Natural red 4), which cannot be digested or absorbed from the gut lumen was used as an index of total gastrointestinal transit time analysis to study gastrointestinal motility. A cocktail of 10 mg/body weight Carmine red (Merck KGaA, Darmstadt, Germany) was prepared together with 5% (w/w) gum Arabic as a vehicle. The total gastrointestinal transit time of carmine throughout the alimentary canal was measured, from the beginning of administration until the excretion of the first colored fecal pellet. Rats were separated in an individual transparent polycarbonate cage and fasted but with ad libitum access to drinking water, for 8 h prior to gastrointestinal transit time analysis. Carmine egestion by each individual rat was observed and recorded at 1 h time interval for 24 h [42].
Charcoal meal test was used to measure the charcoal propulsion along the small intestinal section of the alimentary canal. A standard black charcoal meal was prepared by combining 10% (w/w) activated charcoal suspension and 5% (w/w) gun Arabic. Rats were fasted but with ad libitum access to drinking water, for 12 h prior to charcoal meal test. Each rat was administered with the black charcoal meal by intragastric gavage using stainless steel, 21-gauge round-tip feeding needle. After 30 mins of black charcoal administration, each rat was sacrificed by means of carbon dioxide inhalation [43]. A midline laparotomy was performed, where the entire gastrointestinal tract was carefully removed with gastro-esophageal junction, pyloric sphincter, and ileocecal junction were ligated. The propulsive distance of black charcoal meal traveled between pylorus to caecum, under a tension-free state was measured in (cm). The entire length of small intestine was measured for each rat, then gastrointestinal motility was expressed as a percentage (%) distance traveled by the black charcoal meal in ratio to the entire length of small intestine [44].
Animals were sacrificed by anesthetic inhalation of carbon dioxide after 12 h of fasting. A midventral incision was performed, to expose the animal’s abdominal viscera and the gastrointestinal tract was exteriorized. The entire length of the small intestine and large intestine was measured. The visual appearance of the gastrointestinal tract was compared with the control group for any visual pathological changes in the structure.
A portion of colon was collected from each individual rat. Colon tissue was cleaned by flushing out the gut contents with 0.9% sterile saline and cut into approximately 1 cm segments. The colonic segment was slit longitudinally and fixed in 10% neutral buffered fixative formalin solution at room temperature for 12 to 48 hrs. After fixation, the colonic segment was properly trimmed to an adequate size and orientation for subsequence procedures. Next was pre-embedding to replace water content in tissue samples with wax material by infiltration of liquid paraffin. The sequential process of dehydration of tissues in increased alcohol concentration solutions, then gradual replacement of alcohol by liquid paraffin solvent using tissue processor (Excelsior™ AS Tissue Processor, Thermo Fisher Scientific, Waltham, MA, USA). Consequently, the colonic segment was embedded into acetal polymer cassette after carefully position it to the correct orientation inside a Shandon™ stainless-steel base mold (Thermo Fisher Scientific). The solidified paraffin block was trimmed and fashioned for the tissue sectioning step. The tissue block was properly mounted on the rotator microtome (Histo-Tek® SRM™ II, Sakura Finetek, Torrance, CA, USA) to produce 4 – 5 μm-thick colonic tissue section. The ribbon of colonic tissue section was collected and floated on a water bath maintained at 45°C–50°C to stretch the paraffin section. The ribbon was gently cut into an individual section and placed on a microscope glass slide (Thermo Scientific Menzel-Gläser Superfrost® Plus, Thermo Fisher Scientific) then delicately removed from the water bath. Then, the tissue section was allowed to dry over a hot plate at 60°C for at least 60 mins. Next was Hematoxylin and Eosin (H & E) staining procedure. The paraffin-embedded sections were deparaffinized in xylene for 2 mins, twice and subsequent passage through a descending series of ethanol concentration at 95%, 80%, 70%, and 50% (v/v), each for 2 mins duration and washed with tap water. Staining was performed by submerging the glass slides containing colonic section in Hematoxylin for 10 mins, rinsed through running tap water for 2 mins followed by counterstained with alcoholic Eosin (pH 3.5) for 20 mins. The slides were treated with 95% (v/v) ethanol for 30 dips and 6 mins in absolute ethanol to remove water. Then cleaned by submerging the glass slide in xylene for 6 mins. The colonic section was mounted under a glass coverslip with dibutyl phthalate-polystyrene-xylene (DPX) mountant. Stained colonic sections were examined under a digital microscope (HumaScope Classic, Wiesbaden, Germany), and generated photomicrographs were saved for further analysis [45]. The number of mucus-producing cells (cell/mm2 of colonic mucosa) were quantified histomorphometrically via an automated image analyzer [37].
Data were analyzed using the SPSS statistical (version 22.0; SPSS, Chicago, IL, USA) and the results were expressed as mean ± SEM). Differences among experimental groups were analyzed using one-way analysis of variance (ANOVA), with a significant difference level at p < 0.05. Mean comparisons were assessed by post-hoc Turkey’s test.
RESULT
The final body weight, body weight gain, total feed intake across experimental periods, and feed efficiency were documented as shown in (Fig. 1). There was a significant difference (p = 0.006) in the final body weight of aged rats compared to aged rats with loperamide-induced constipation, while there was no significant difference between naïve rats. There was no significant difference in the final body weight between all the treatment groups compared to aged rats with loperamide-induced constipation (Fig. 1A). However, aged rats showed a lower (p = 0.029) in body weight gain when compared to naïve young rats and a higher (p < 0.001) body weight gain when compared to aged rats with loperamide-induced constipation. Lactulose, L. fermentum 4189, and L. plantarum 4187 treatment groups showed a higher (p < 0.001) body weight gain when compared to aged rats with loperamide-induced constipation (Fig. 1B). Although there was no significant difference in feed intake between naïve young rats and aged rats, the feed efficiency was 4.48% higher (p = 0.011) in naïve young rats. There was a higher (p = 0.001) in feed intake and 3.31% higher feed efficiency between aged rats compared to aged rats with loperamide-induced constipation. A higher feed intake in lactulose and L. fermentum 4189 treatment groups were observed compared to aged rats with loperamide-induced constipation (p < 0.001 and p = 0.003, respectively), also a higher (p = 0.001) feed efficiency. L. plantarum 4187 treatment group showed 6.58% higher (p < 0.001) feed efficiency compared to aged rats with loperamide-induced constipation although no significant difference in feed intake (Figs. 1C and 1D).
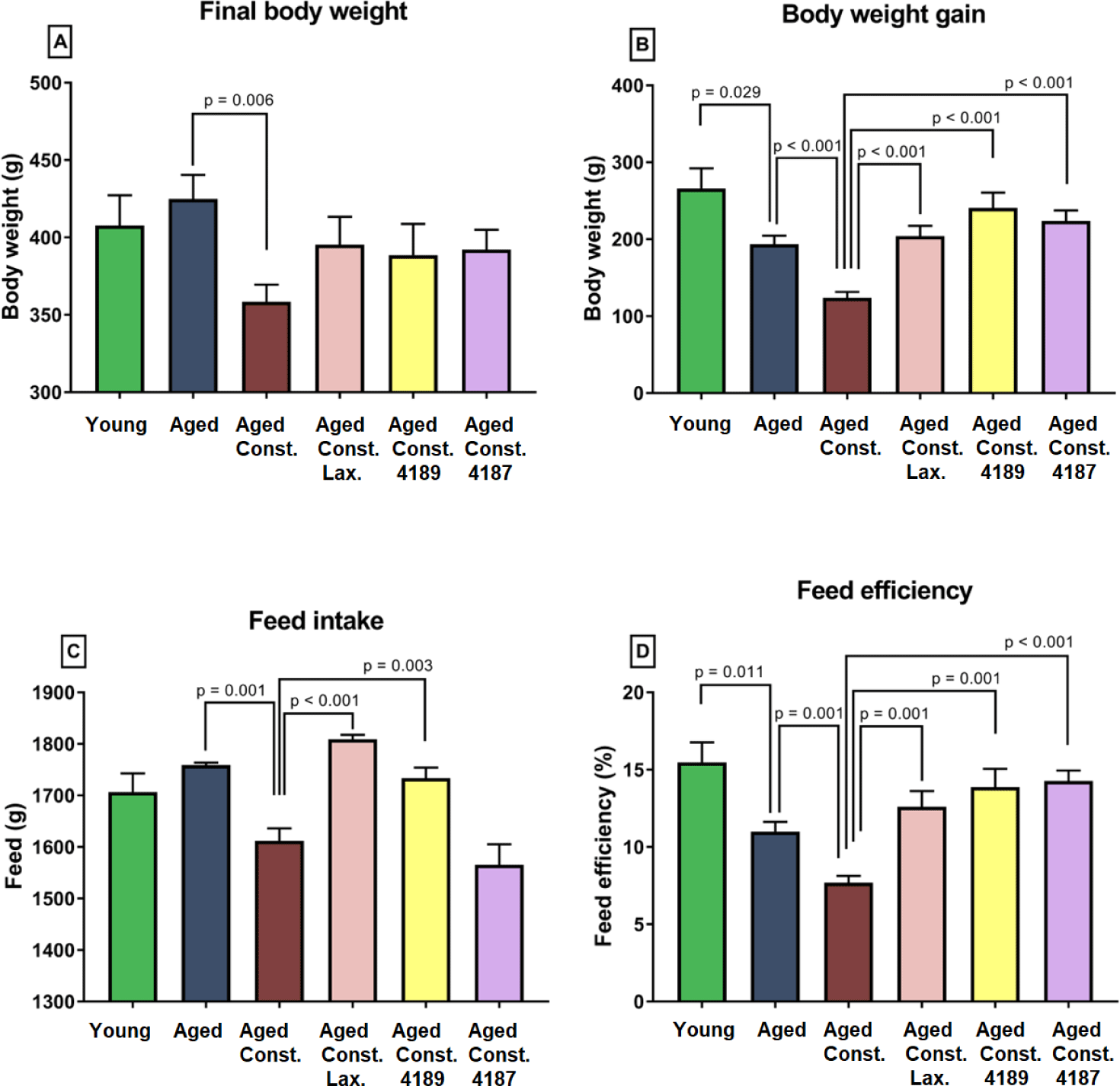
Liver chemistry profile measured from rat’s serum collected upon 12 weeks of experimental periods as results from liver function test, which include total protein, albumin, globulin, A/G ratio, total bilirubin, AST, ALT, and ALP is shown in (Fig. 2). The result from the test showed that the administration of lactulose, L. fermentum 4189, or L. plantarum 4187 did not cause any prevalent changes in majority of liver function properties. There was a lower in total protein (p = 0.024) and albumin (p = 0.027) between aged rats administered with lactulose compared to aged rats with loperamide-induced constipation group (Figs. 2A and 2B). L. plantarum 4187 treatment group has a lower (p = 0.037) total protein as compared to the aged rats with loperamide-induced constipation (Fig. 2A). The total bilirubin was higher (p < 0.001) in aged rats compared to naïve young rats while lower (p = 0.004) when compared to aged rats with loperamide-induced constipation. L. plantarum 4187 treatment group has a lower (p = 0.001) in total bilirubin as compared to the aged rats with loperamide-induced constipation (Fig. 2E). Administration of L. fermentum 4189 had a higher (p = 0.009) AST concentration compared to aged rats with loperamide-induced constipation (Fig. 2F). ALT concentration in aged rats with loperamide-induced constipation was higher (p = 0.018) compared to aged rats (Fig. 2G). The renal function profile measured from rat’s serum collected upon 12 weeks of experimental periods which include sodium, potassium, chloride, urea, creatinine, uric acid, calcium, and phosphate as shown in (Fig. 3). The result from the test showed that the administration of lactulose, L. fermentum 4189, or L. plantarum 4187 did not cause any prevalent changes in the majority of renal function properties. Potassium concentration in naïve young rats was higher (p = 0.024) compared to aged rats (Fig. 3B). A higher (p = 0.009) urea concentration in aged rats compared to naïve young rats was observed while lower (p = 0.006) as compared to aged rats with loperamide-induced constipation (Fig. 3D). Administration of L. plantarum 4187 had a higher (p = 0.011) creatinine concentration when compared to aged rats with loperamide-induced constipation (Fig. 3E). Meanwhile, the administration of L. fermentum 4189 or L. plantarum 4187 had a higher (p = 0.001) phosphate concentration as compared to aged rats with loperamide-induced constipation (Fig. 3H).
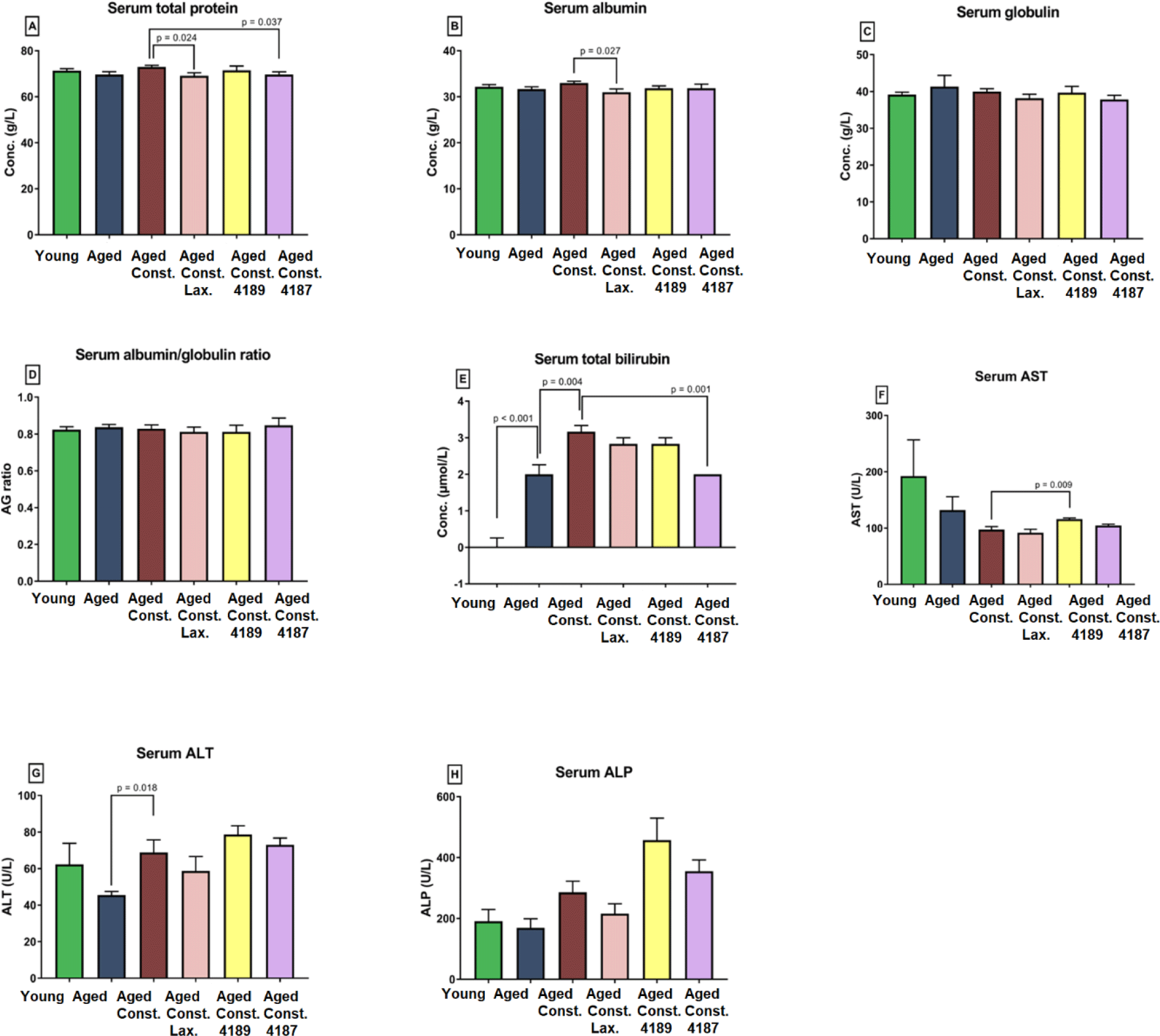
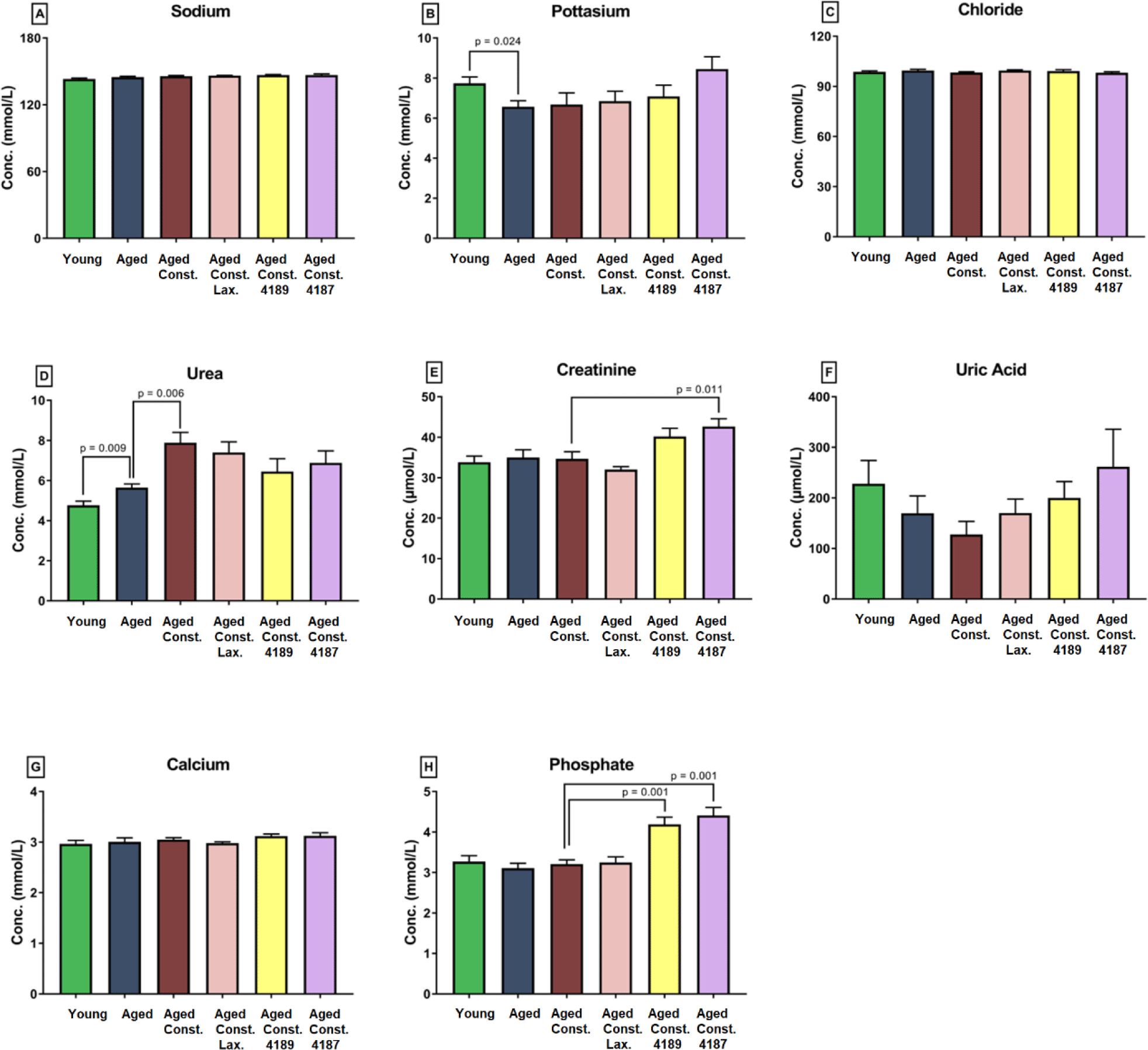
Aged rats have a shorter (p = 0.025) telomere length as compared to naïve young rats after D-galactose-induced aging injection (Fig. 4). Meanwhile, there was no significant difference telomere length in all treatment groups when compared to aged rats with loperamide-induced constipation.
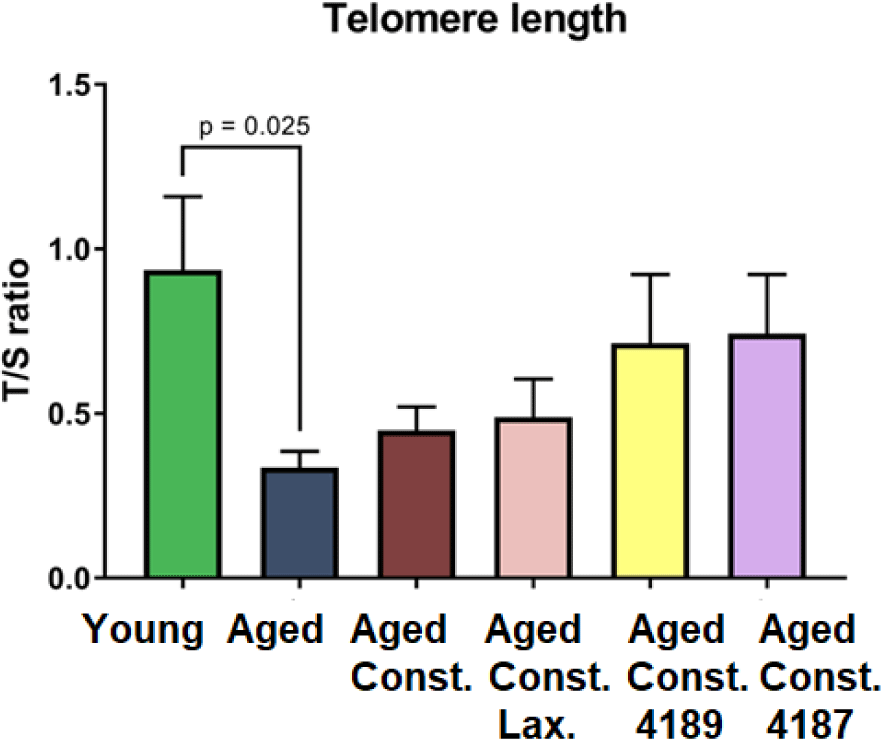
There were no significant changes in fecal moisture content in aged rats as compared to naïve young rats after D-galactose-induced aging injection (Fig. 5). A lower (p = 0.039) fecal moisture content was observed in aged rats with loperamide-induced constipation as compared to aged rats. Administration of lactulose, L. fermentum 4189, or L. plantarum 4187 were able to ameliorate the adverse effect of loperamide-induced constipation, with a higher (p < 0.001, p = 0.014, and p = 0.001, respectively) fecal moisture content as compared to aged rats with loperamide-induced constipation. Changes in fecal morphological and consistency of fecal matter in all experimental groups were evaluated based on the Bristol Stool Chart (Fig. 6B). Fecal from aged rats with loperamide-induced constipation are categorized under types 1 and 2, which indicated constipation. Meanwhile, the administration of lactulose, L. fermentum 4189, or L. plantarum 4187 were categorized under types 3 and 4, which represented a normal healthy fecal morphology (Fig. 6A). The loperamide-induced experimental group produced mainly a dry, hard, and small fecal matter, which corresponded to a lower fecal moisture content as mentioned above.
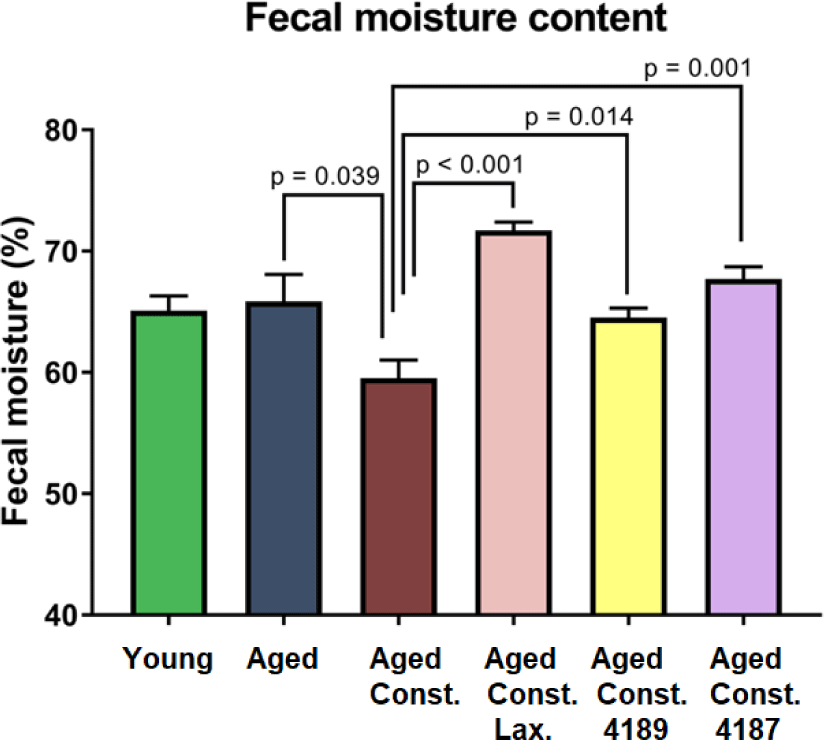
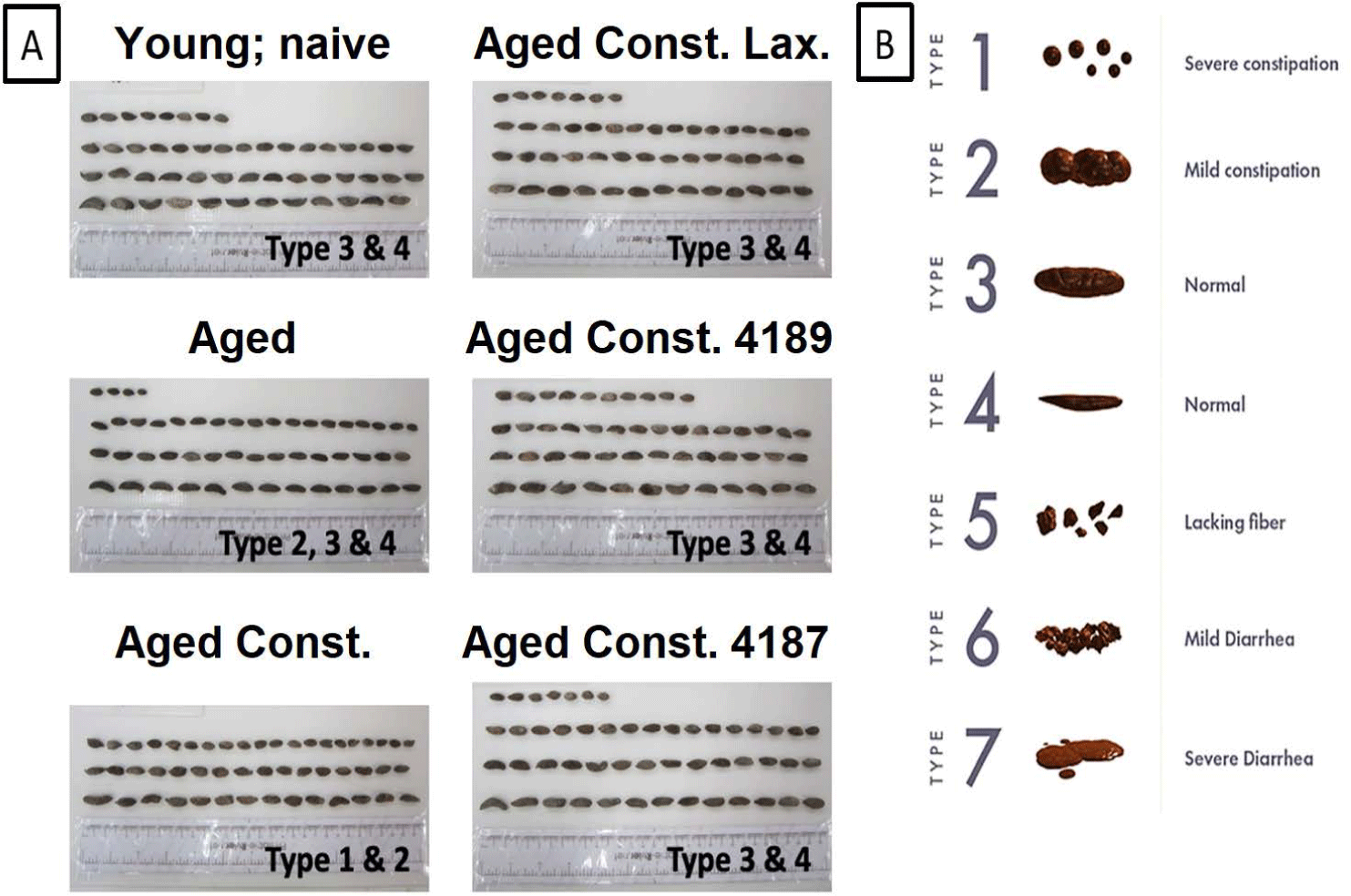
A distinct SCFA heatmap profile pattern was observed between the experimental group, which include acetate, propionate, isobutyrate, butyrate, isovalerate, valerate, hexanoate, lactate (Fig. 7A). The individual concentration of SCFA was analyzed by independent T-test however showed no significant difference between experimental groups (Figs. 7B, 7C, 7D, 7E, 7F, 7G, 7H, 7I and 7J), except for acetate and butyrate. Acetate concentration (Fig. 7B) was significantly higher (p = 0.039) in aged rats compared to naïve young rats. Also, butyrate concentration (Fig. 7E) was significantly lower (p = 0.012) in aged rats with loperamide-induced constipation compared to aged rats. A total of 256 water-soluble compounds were detected with district profiles between treatment groups (Fig. 8A). The metabolites were grouped into three major clusters, where the concentration of metabolites of cluster 1 was observed to be lower in naïve young rats. Concentration of metabolites in cluster-2 were higher in aged rats with loperamide-induced constipation, but were higher in cluster-3 from naïve young rats. The concentration of threonine was identified to be different between experimental groups (Fig. 8B). The administration of lactulose, L. fermentum 4189, or L. plantarum 4187 showed a reduction in fecal threonine concentration as compared to aged rats with loperamide-induced constipation (p = 0.013, p < 0.001 and p < 0.001 respectively).
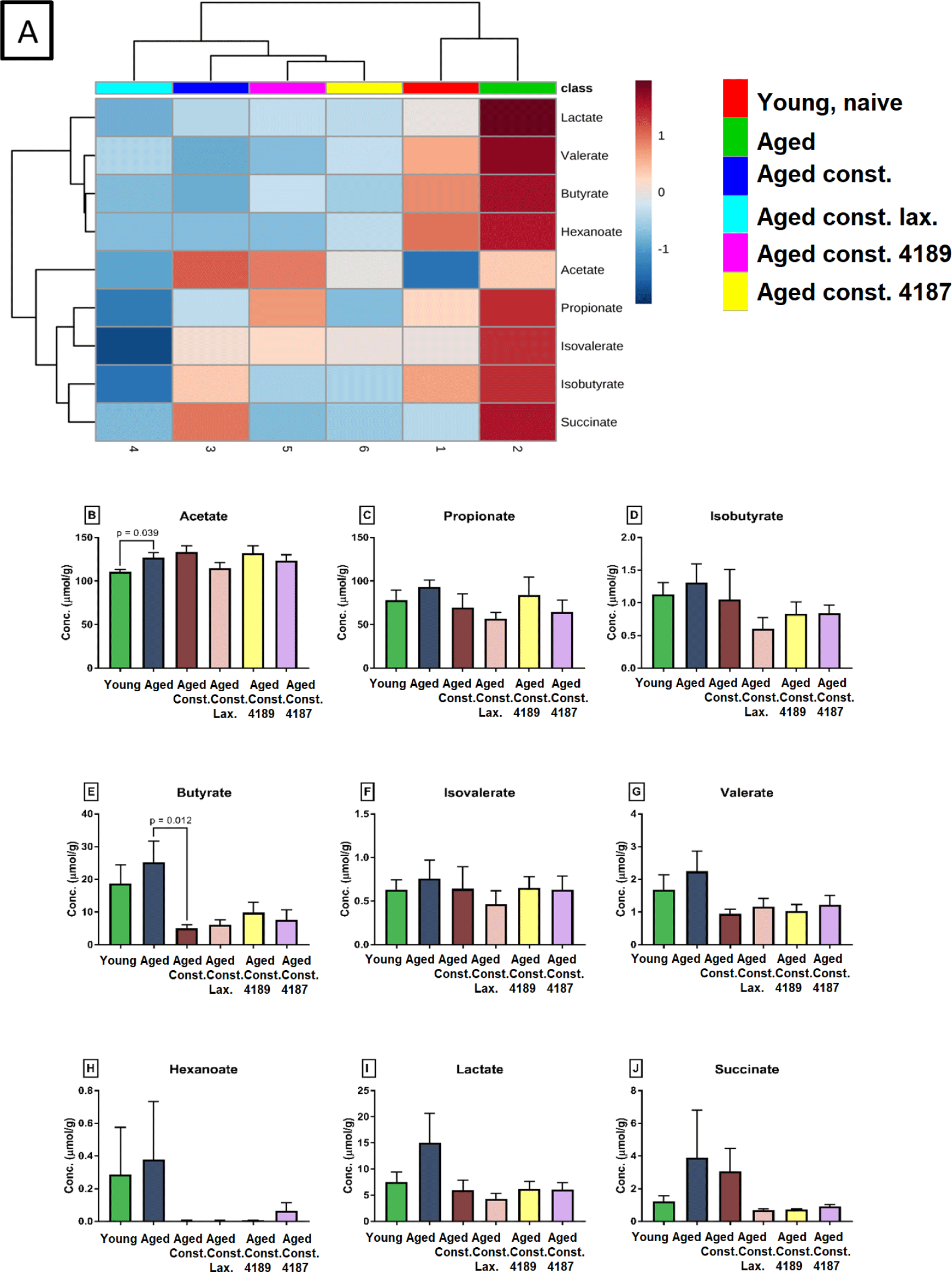
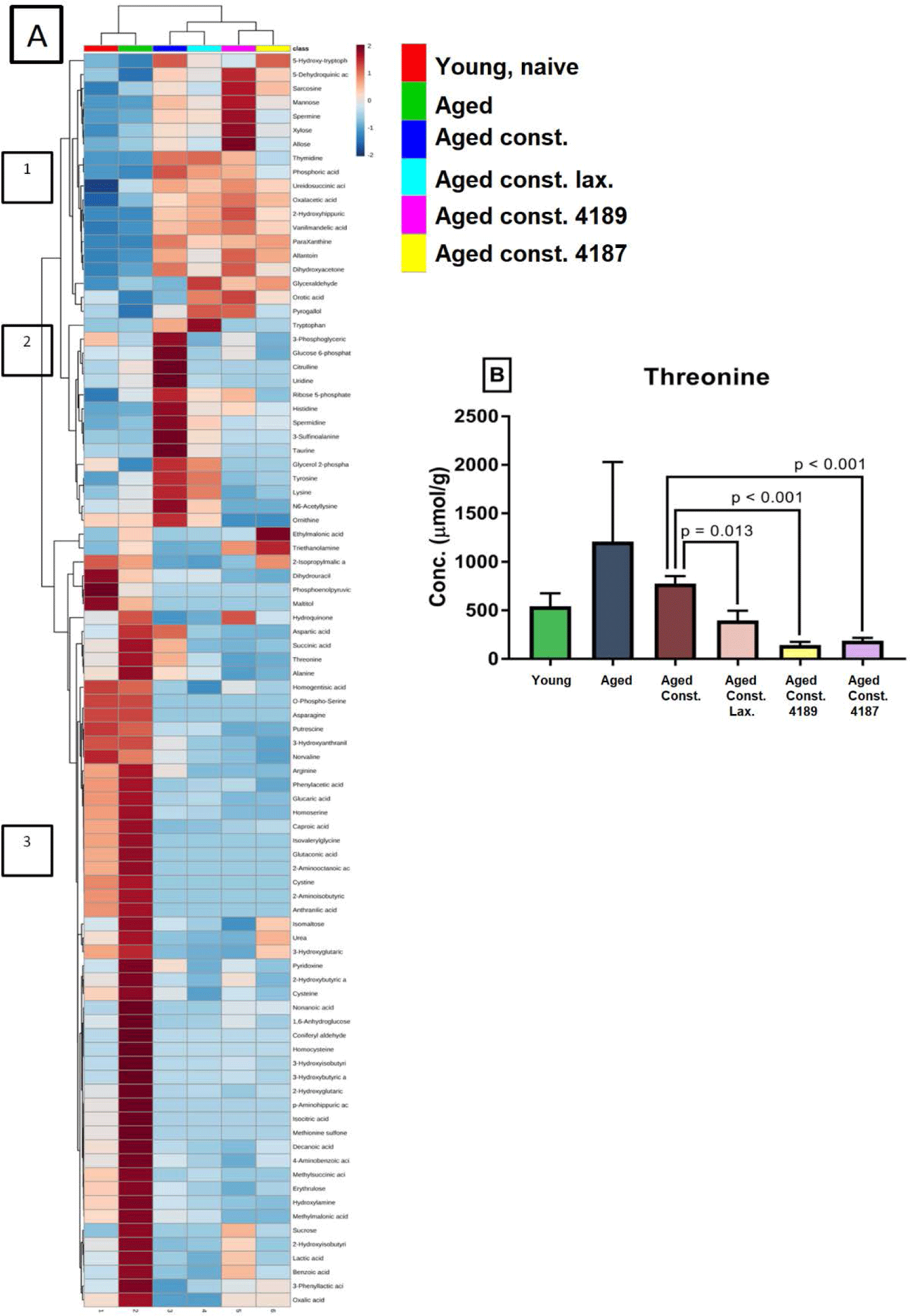
Gastrointestinal motility as quantified with carmine travel and charcoal meal propulsion test showed that the administration of lactulose, L. fermentum 4189, or L. plantarum 4187 did not cause any significant changes in the gastrointestinal motility of rats (Figs. 9A and 9B).
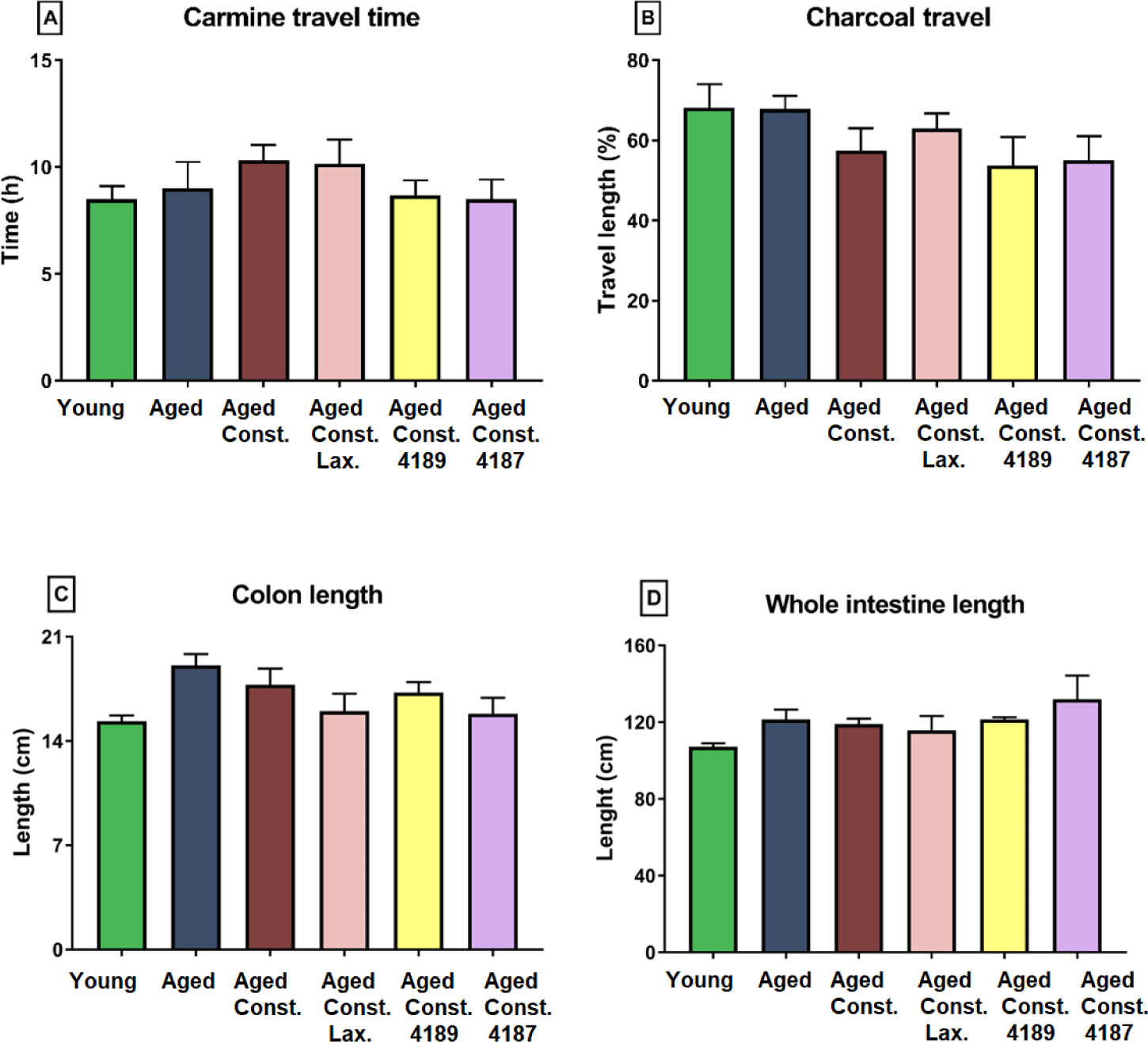
Length of colon and whole intestine was not significantly different between groups administered with lactulose, L. fermentum 4189, or L. plantarum 4187 (Figs. 9C and 9D). Similarly, loperamide-induced constipation also did not alter any changes attributed to length of colon and whole intestine. Aged rats had a lower (p = 0.018) goblet cell count compared to young naïve rats, while higher (p = 0.003) as compared to aged rats with loperamide-induced constipation (Fig. 10A). The administration of lactulose, L. fermentum 4189, or L. plantarum 4187 were able to ameliorate the adverse effect of loperamide-induced constipation, accompanied by a higher (p = 0.007, p = 0.014, and p < 0.001, respectively) goblet cell count as compared to aged rats with loperamide-induced constipation. The structure of goblet cells in aged rats and aged rats with loperamide-induced constipation are less dense compared to other groups. The goblet cells in groups that administered with lactulose, L. fermentum 4189, or L. plantarum 4187 are packed and dense that creates compact mesh-like network of viscous, permeable, mucin, which provides the frontline host defense against endogenous and exogenous irritants (Fig. 10B).
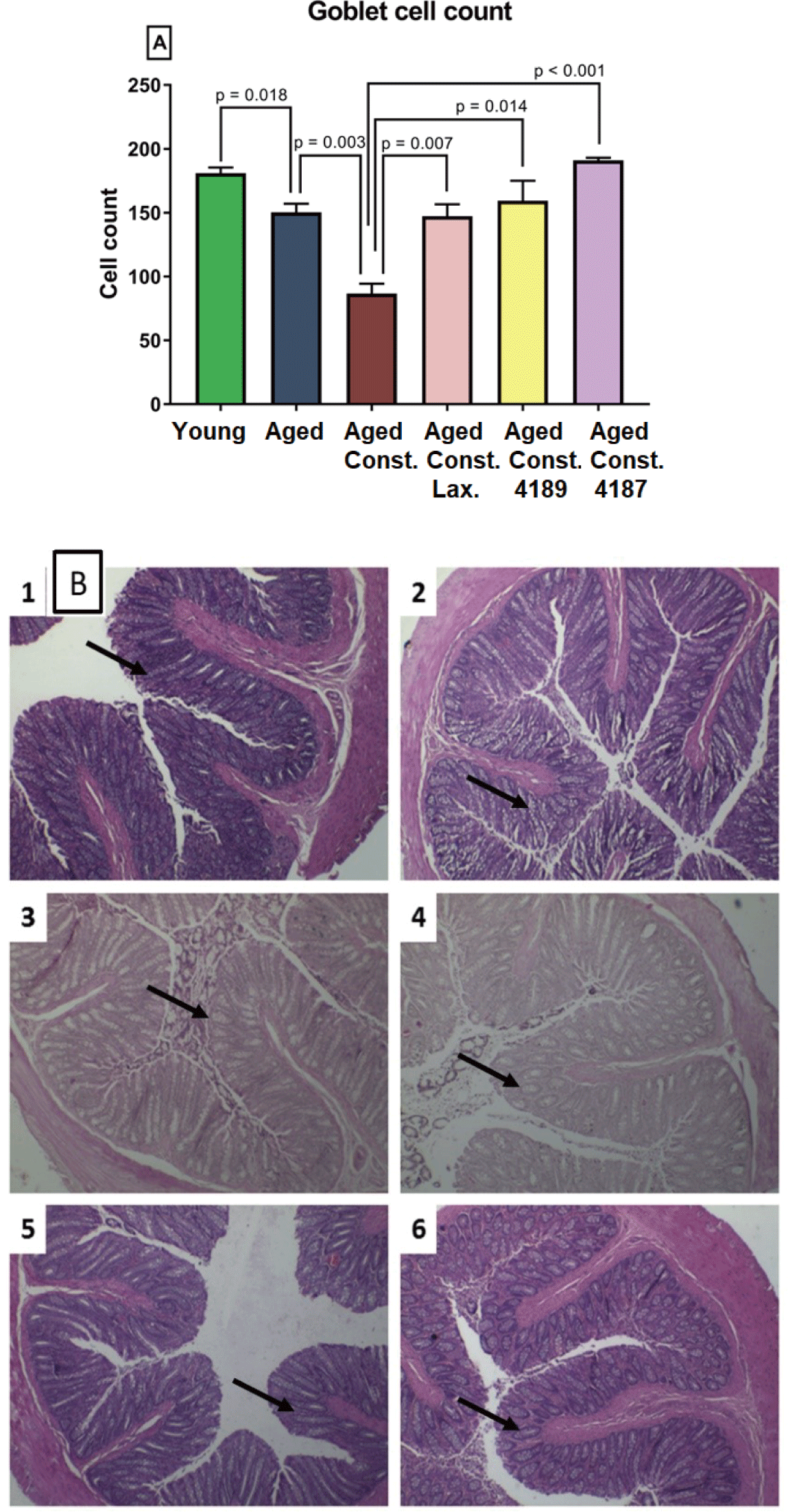
DISCUSSION
Constipation is not a disease but rather a gastrointestinal symptom that is variably defined and involves difficulty in the defecation process, a condition frequently more prevalent amid aging. Strains of LAB has been reported to improve gut health during its transient passage across the gastrointestinal system in humans, companion animals and livestock [24].
In this study, rats were given D-galactose to induce aging, and administration of loperamide as a constipation inducer. In general, all treatments did not show any deleterious effect while no mortality cases were reported. The administration of lactulose, L. fermentum 4189, and L. plantarum 4187 had shown a similar trend of body weight gain when compared with young rats, whereas loperamide and D-gal induced a significant reduction in body weight gain. As a synthetic non-absorbable disaccharide composed of fructose and galactose, lactulose has been used as a laxative for constipation treatment. Unlike sodium picosulphate which is commonly used as a stimulant laxative to treat constipation, lactulose is an osmotic laxative [37]. Sodium picosulphate has been shown to reduce body weight gain due to its strong laxative/purgative activity. In contrast, lactulose reaches the lower gastrointestinal tract which increases water retention through osmosis to exert an osmotic effect [46,47]. Similarly, the subcutaneous injection of loperamide had been reported previously to cause a reduction in body weight gain. The mechanism of loperamide to reduce feed intake however remain unclear. Previous study reported that constipation episodes may diminish appetite [48]. In addition to dry and hard fecal matter, constipation adversely affects nutritional status by causing abdominal pain, bloating, and nausea that elicits loss of appetite [49].
The administration of LAB strains in rats was demonstrated to be safe as seen by the biochemical liver and renal profiles, despite amid constipation induction with loperamide. Blood serum chemistry test which includes liver and renal parameter changes are the recommended nonclinical toxicity and studies in laboratory animals [50–52]. The liver and kidneys are vital visceral organs of detoxification, metabolism, storage including excretion of xenobiotics, and their metabolites [53]. Both organs are especially vulnerable to damage upon exposure to toxins matter where usually the abnormality attributes could indicate potential organ toxicity [53]. Data from our study suggested that the selected LAB strains did not impose deleterious health risk and toxicity.
The liver chemistry profile showed that the administration of L. plantarum 4187 had significantly reduced serum bilirubin concentration in aged rats with loperamide-induced constipation. A high bilirubin level in serum represents a good prognostic measure of liver injury [54]. A yellowish compound, bilirubin is a natural waste product from the breakdown and destruction of abnormal and/or aged blood cell hemeprotein in the systemic circulation [55]. In which it is a vital product that is eliminated via the liver from the body with diagnostic values. Therefore, these heme catabolic metabolites represent a good indicator to evaluate liver functional capacity [56].
Renal function profile showed that the administration of L. plantarum 4187 had significantly increased the serum creatinine concentration in aged rats with loperamide-induced constipation. Serum creatinine is usually produced at an adequate constant rate in the body from the breakdown of creatine phosphate in muscle [57]. The elevated serum creatinine in L. plantarum 4187 groups may due to its significant higher in body weight gain as compared to aged rats with loperamide-induced constipation. Creatinine level in the blood is correlated with total skeletal muscle mass as its creation materializes almost exclusively by the muscle [58,59]. A lower serum creatinine indicates skeletal muscle mass loss, which is also demonstrated to be positively associated with an increased risk of dysglycemia episode [57].
D-galactose subcutaneous injection has been used to mimic the metabolic imbalance during the natural aging process. Low dose injection of D-galactose has been demonstrated to accelerate the aging process in which imitating aging effect [60]. D-galactose exposure was used to induce an aging model by increasing oxidative stress accumulation in laboratory animals [61]. Monosaccharide reducing sugar, D-galactose is a physiological nutrient that reacts with free amines of amino acid in proteins via nonenzymatic glycation to form advanced glycation end-products. However, chronic systemic exposure of D-galactose contributes to the production of endogenous reactive oxygen species (ROS) through D-galactose oxidative metabolism and glycation end-products [62,63]. Therefore, chronic systemic exposure of D-galactose is hypothesized to shorten the telomere length, which gives rise to an artificial accelerated aging model. Data from our study showed that D-galactose had significantly shortened the telomere length of aged rats when compared to naïve young rats.
In the present study, loperamide-induced experimental group marked a significantly lower fecal moisture content. Administration of lactulose, L. fermentum 4189, or L. plantarum 4187 had prevented fecal moisture loss after loperamide-induced constipation, which able to ameliorate the antisecretory effect of loperamide induced constipation that inhibit intestinal water secretion [64]. Previously, positive effects of the LAB administration had been proven to significantly increase the fecal softness also the frequency of the defecation [65,66]. The dry, hard, and small fecal matter could derive from the antisecretory effect of loperamide [67]. The use of Bristol stool chart to describe fecal morphology improvement with ingestion of beverage containing LAB had been previously reported [68]. An increase in fecal bulk and soften fecal attribute is considered a beneficial physiological effect, provided LAB treatment does not result in diarrhea [69]. In line with these scientific requirements, fecal consistency accessed with Bristol stool chart exhibit an increased fecal bulk and slight soften fecal upon administration of L. fermentum 4189, or L. plantarum 4187.
Intestinal microbiota has a great influence on the variations of metabolites, which subsequently play important roles in a myriad of diseases and health disorders [70]. Gut microbiota ferment complex polysaccharides and proteins that escape digestion or absorption in the small intestine to produced primarily SCFA compounds includes acetate, butyrate, and propionate [71,72]. These volatile fatty acids which characterize by their fewer than six carbons backbone had previously reported having distinct physiological effects on the host. SCFA play a paramount role in shaping the gastrointestinal environment, influencing colonic physiology, and participating in different host-signaling mechanisms [73]. SCFA compounds can also be utilized as energy sources by host local cells and the intestinal microbiota, while strains of LAB have been reported to alter SCFA profiles [74].
In the present study, the oral administration of L. fermentum 4189, or L. plantarum 4187 did not impose any significant alteration on the SCFA concentration in aged rats with loperamide-induced constipation. Likewise, the administration of L. casei strain Shirota had been reported to significantly reduced the episode of hard and lumpy fecal in healthy populations without affecting the levels of SCFAs [75,76].
Threonine plays important role in intestinal mucosal integrity and barrier function. The protein fundamental of intestinal mucins has a distinctive amino acid composition, in which threonine comprises 25% of total amino acid residue in rat colonic mucins [77,78]. As threonine is used as a building block to produce mucin, while a lower concentration of threonine was found in fecal samples of rats administered with lactulose, L. fermentum 4189, or L. plantarum 4187 as compared to the aged-constipated control, we postulate that lactulose, L. fermentum 4189, and L. plantarum 4187 prevented mucosal degradation while preserving integrity during aging.
Goblet cells play a vital part in protecting the mucous membrane by maintaining its mucus layer, especially in the colonic intestinal epithelium. The number of these mucus-producing cells can be detected via histopathological examination [37]. A high number of goblet cells were observed in the lactulose, L. fermentum 4189 and L. plantarum 4187 groups as compared to aged rats with loperamide-induced constipation, indicating better mucosal protection during constipation. Constipation is usually associated with reduced mucus production in the colonic mucosa which results in a decrease in colonic mucosa thickness and the number of goblet cell count [79]. In addition, loperamide had previously shown to extent the evacuation time, inhibit fluid secretion and reduce colonic mucus [80,81].
CONCLUSION
Dairy-isolated LAB strains were able to ameliorate the adverse effect of loperamide-induced constipation via preventing fecal moisture loss, increasing fecal bulk, softening fecal matter and preserving the number of goblet cells in the colon. As LAB strains did not affect gastrointestinal motility, we postulate that the rescue effect on loperamide-induced constipation may not be attributed to bowel movement, which may be beneficial for the development of gut probiotics for osmotic laxative effect without risks of causing diarrhea.