INTRODUCTION
Obesity, which is a multifaceted metabolic disorder characterized by a high body mass index (BMI) and the excessive accumulation of fat, has escalated into a global public health crisis. According to the World Obesity Federation Atlas 2023, >v2.6 billion people were overweight (BMI ≥ 25kg/m2), and 1 billion people were obese (BMI ≥30kg/m2) in 2022. This number is expected to be approximately 4 billion people by 2035 [1]. The prevalence of obesity is projected to increase by 18% in men and 21% in women by 2025 [2] and a total of 24% by 2035 [1]. This worldwide epidemic has resulted in several epidemiological studies, which have revealed a complex relationship between genetic, environmental, and behavioral risk factors that propel the onset and progression of obesity [3–6]. There are several health repercussions associated with obesity, including cardiovascular disease, type 2 diabetes, and the development of certain cancers, which place considerable strain on public health systems [7–10]. Current therapeutic strategies have failed to deliver sustained weight loss or address the physiological nuances of obesity, which highlights the need for novel treatment approaches. Adipose tissue is the central hub for lipid storage and is important in determining whole-body insulin sensitivity. The development and progression of obesity involve the white and brown adipose tissues, which are the predominant focus of obesity research [11]. The mouse-derived preadipocyte 3T3-L1 cell line is a widely used model for obesity research because of its robust and consistent differentiation into mature adipocytes. This makes it a suitable in vitro model for studying adipogenesis, insulin response, lipid metabolism, and therapeutic development[12,13]. The differentiation of preadipocytes into mature fat-storing cells is important to adipose tissue dynamics and function. Therapeutic targeting of this pathway offers significant potential in obesity management. Adipogenesis and lipogenesis are key processes that are important for managing fat storage and the conversion of excess energy into lipids within the liver and adipose tissues. This intricate process is associated with the expression of a myriad of genes that contribute to adipogenesis and lipogenesis [14]. The regulation of these genes and their ability to up- or downregulate adipogenesis and lipogenesis in various organs represent a significant area of study. Understanding the mechanisms underlying their regulation may reveal novel targets and strategies for treating obesity and its metabolic complications [15].
Natural products are major repositories for bioactive compounds that may be exploited for allopathic drug development [16]. Numerous well-established drugs, either isolated from or semisynthesized based on natural structures, are an important resource of potential therapeutic agents [17,18]. For example, metformin, a first-line drug for type 2 diabetes, was derived from a natural compound guanidine found in the French lilac [19]. Similarly, the weight loss drug orlistat is related to lipstatin, a natural product derived from the bacterium Streptomyces toxytricini [20]. With respect to obesity, several natural products have demonstrated promise, with certain compounds exhibiting a significant impact on the maturation of 3T3-L1preadipocytes by regulating various adipogenic and lipogenic genes [12,21,22].
Elsholtzia fruticosa (EF) is an aromatic plant belonging to the Lamiaceae family, which is the sixth-largest family of flowering plants [23]. This plant grows in elevated regions of the Himalayas, spanning Pakistan, Nepal, and China, at altitudes ranging from 1,800 m to 3,300 m [24, 25]. EF and its congeneric species have been used in traditional medicinal practices throughout the region. The essential oil of EF contains several bioactive compounds, such as eucalyptol, perillen, γ-terpinene, caryophyllene, limonene, p-cymene, 1,8-cineole, and carbofuran . These molecules contribute to its complex chemical profile and therapeutic activities. EF oil exhibits antiparasitic effects on Ditylenchus destructor and antibacterial properties [24–30]. The anti-inflammatory and antimicrobial effects have already been demonstrated [29,30]; however, their role in obesity management remains unclear.
In this study, EF promoted preadipocyte maturation in vitro and increased the expression of adipogenic genes, such as peroxisome proliferator-activated receptor gamma (PPARγ), CCAAT enhancer-binding proteins (C/EBPα and C/EBPβ), fatty acid binding protein 4 (FABP4), adiponectin, as well as lipogenic genes, such as fatty acid synthase (FAS), stearoyl-CoA desaturase (SCD1), SCD2, and acetyl- CoA carboxylase (ACC), which play a significant role in preadipocyte maturation. Thus, EF has the potential as a natural therapeutic for the treatment of obesity.
MATERIALS AND METHODS
The methanol extract of EF was procured from the International Biological Material Research Center at Korea Research Institutes of Bioscience and Biotechnology, Daejeon, Korea. The deposit number is FBM198-050.
Mouse pre-adipocyte 3T3-L1 cells were obtained from the American Type Culture Collection (ATCC, USA) and cultured in Dulbecco’s Modified Eagle’s Medium (DMEM; Welgene, Gyeongsan, Korea) supplemented with 10% bovine calf serum, 100 U/mL penicillin, and 100 μg/mL streptomycin at 37°C in 5% CO2. Cell differentiation began 2 days after seeding at 100% confluence (Day 0). Cell differentiation as performed as described before [31]. Initially, they were stimulated with a hormone cocktail (0.1 × MDI) consisting of 0.05 mM 3-isobutyl-1-methylxanthine (IBMX), 0.1 μM dexamethasone, and 0.1 μg/ml insulin in 10% fetal bovine serum (FBS)-containing DMEM medium for two days in the presence or absence of EF. The differentiation medium was replaced with DMEM supplemented with 10% FBS and 1 μg/mL insulin every 2 days until day 6. The preadipocytes were transformed into mature adipocytes that were rounded and filled with oil droplets as determined by microscopy.
The WST-8 Cell Viability Assay Kit (BIOMAX, Guri, Korea) was used to assess cell viability as described previously [31]. The assay measures the capacity of live cells to convert the WST-8 tetrazolium salt into formazan by mitochondrial dehydrogenases. The 3T3-L1 preadipocytes were seeded at a density of 1 × 104 cells/well in 96-well plates and cultured for 24 h. The cells were exposed to varying concentrations of EF extract and incubated for 24, 48, and 72 hours. The WST-8 reagent was then added and incubated for 4 h at 37°C. The absorbance of the resulting formazan was measured at 450 nm using an iMarkTM microplate reader (Bio-Rad Laboratories, Hercules, CA, USA). Cell viability was determined relative to untreated cells, which were considered 100% viable.
Cells were seeded into 12-well plates and subject to various treatments. Oil red O staining was performed as described previously [32]. Briefly, cells were rinsed twice with phosphate-buffered saline (PBS) and fixed with 4% paraformaldehyde for 1 h at room temperature. After washing, 100% isopropanol was added to each well and incubated for 25 min. Subsequently, the cells were stained with Oil-Red O solution for 30 min and repeatedly washed with distilled water until clear. The stained lipid droplets were observed with a NIB410 microscope (Nexcope, Ningbo, China) at 100× magnification.
The expression of various genes associated with lipogenesis and adipogenesis in 3T3-L1 cells was analyzed by qRT–PCR. Total RNA was extracted using the RiboExTM reagent (GeneAll Biotechnology, Seoul, Korea). The RNA was reverse-transcribed into cDNA using the ReverTra AceTM qPCR RT kit (Toyobo, Japan). qRT–PCR was carried out using the SYBR Green PCR Master Mix on the CFX Connect Real-Time PCR system (both from Bio-Rad Laboratories). The mRNA levels of 36B4 were used as an internal control. The specific primer sequences are listed in Table 1.
Western blot analysis was used to measure protein expression in 3T3-L1 cells following EF treatment. The cells were lysed using the Mammalian Protein Extraction Reagent (Thermo Fisher Scientific, Waltham, MA, USA) containing an Xpert protease inhibitor cocktail (GenDEPOT, Katy, TX, USA). After centrifugation at 12,000 rpm for 10 min at 4°C, the supernatants were electrophoresed on precast gels (Bio-Rad Laboratories) and transferred to polyvinylidene fluoride (PVDF) membranes. The membranes were blocked with blocking buffer (BIOMAX, Seoul, Korea) for 5 min and probed overnight at 4°C with antibodies against PPARγ (Cat. No. 2443, Cell Signaling Technology, Danvers, MA, USA), FABP-4 (Cat. No. SC-271529, Santa Cruz Biotechnology, Dallas, TX, USA), Mouse anti-CHOP IgG2a (L63F7) (Cat. No. 2895, Cell Signaling Technology), and HSP90 (Cat. No. SC-13119, Santa Cruz Biotechnology). After washing, the membranes were incubated with mouse and rabbit HRP-conjugated secondary antibodies (1:4,000 dilution, Bio-Rad Laboratories), washed, and the protein bands were detected using an ECL system (Bio-Rad Laboratories).
Following treatment with EF in 3T3-L1 cells, the cells were washed twice with Dulbecco’s PBS (DPBS) and fixed with 4% formaldehyde at room temperature for 30 min. The cells were rinsed twice with DPBS and stained with 1 g/mL Hoechst 33342 for 10 min. After DPBS washing, cell morphology was assessed using a microscope at a magnification of 100×. Images were captured and the nuclei were counted manually to assess cell proliferation. The number of cells was measured using ImageJ software.
The results are shown as the mean ± SEM, and n denotes the number of wells analyzed. Statistical analyses were performed using Prism 9 software (GraphPad Software, Boston, MA, USA). Data were analyzed using a two-way ANOVA followed by Tukey’s post hoc test at α = 0.05. A p-value < 0.05 was considered statistically significant.
RESULTS
When searching for natural products for anti-obesity treatment, the primary challenge is to assess their potential cytotoxicity, as complete plant extracts may contain toxic compounds. Therefore, we performed a cell viability assay to assess the effect of EF treatment on the viability of 3T3-L1 cells. As shown in Fig. 1, EF did not exhibit cytotoxic effects even up to concentrations of 100 µg/mL.
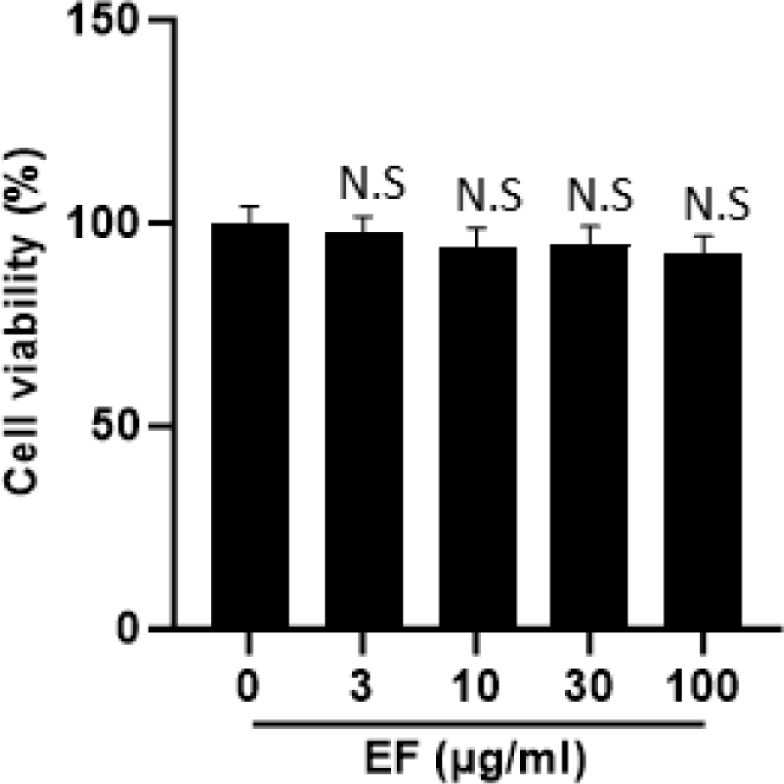
Next, we performed Oil-Red O staining following treatment of the cells with EF in differentiation media to determine its effect on the differentiation of 3T3-L1 preadipocytes into mature adipocytes (Fig. 2). EF treatment significantly increased lipid accumulation in 3T3-L1 cells and resulted in robust differentiation in a dose-dependent manner compared with the untreated control cells (Fig. 2A). These findings suggest that EF effectively promotes the maturation and differentiation of 3T3-L1 preadipocytes into mature adipocytes without causing cytotoxicity.
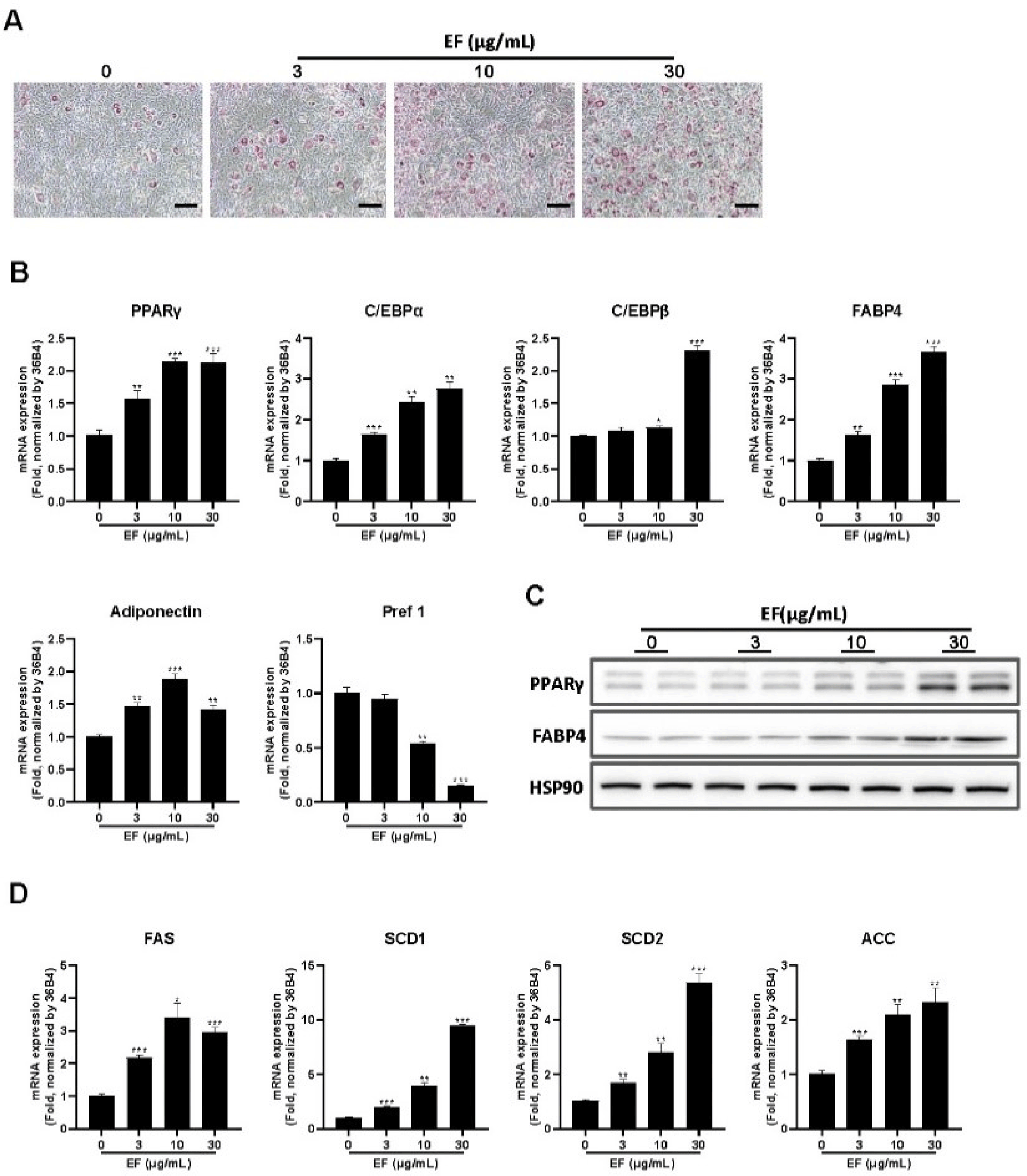
Adipogenesis and lipogenesis are fundamental mechanisms in preadipocytes that are essential for their differentiation into mature adipocytes [33]. This process is mediated by various proteins and hormones, each playing a vital role in driving this transformation. Because increased lipid accumulation was observed in adipocytes differentiated with EF (Fig. 2A), we determined whether this was associated with the modulation of adipogenesis and lipogenesis-related genes in 3T3-L1 cells. A qRT–PCR analysis revealed a dose-dependent increase in the expression of several adipogenic genes, including PPARγ, C/EBPα, C/EBPβ, adiponectin, and FABP4, in EF-treated cells relative to the controls (Fig. 2B). Conversely, the expression of preadipocyte factor-1 (Pref-1; a marker gene for preadipocytes) was significantly decreased (Fig. 2B). To determine the relationship between these mRNA and protein expression, we examined the levels of PPARγ and FABP4 proteins by western blot analysis. Interestingly, EF treatment also upregulated the expression of PPARγ and FABP4 in a dose-dependent manner (Fig. 2C).
Next, we measured the expression of lipogenesis-related genes in 3T3-L1 cells following EF treatment. As shown in Fig. 2D, EF enhanced the expression of genes, including SCD-1, SCD-2, ACC, and FAS in a dose-dependent manner compared with the control group. These results indicate that EF promotes 3T3-L1 preadipocyte differentiation by up-regulating the expression of adipogenesis- and lipogenesis-related genes.
To determine the specific phase of adipocyte differentiation influenced by EF, preadipocytes were cultured to post-confluence followed by treatment with EF according to the schedule outlined in Fig. 3A. Treatments ranged from untreated cells (group 1) to cells treated from days 0 to 6 (group 7). Oil-Red O staining (Fig. 3B) indicated that early treatment (groups 2, 5, and 7) resulted in the most significant lipid accumulation within adipocytes, followed by treatment during the intermediate phases (groups 3 and 6), and was the least effective when administered late (group 4), compared with the untreated group.
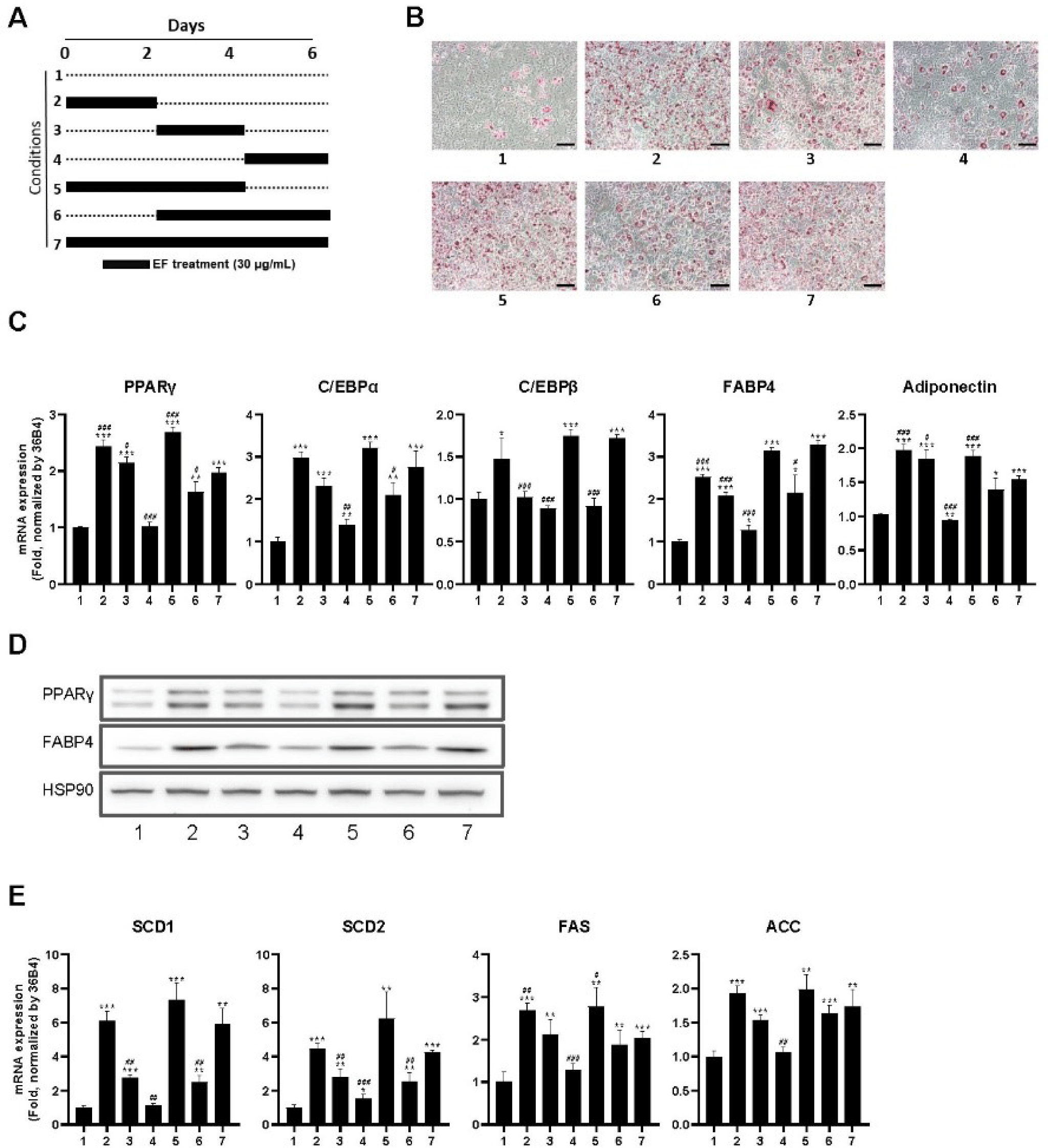
Subsequently, a qRT–PCR analysis of adipogenesis-related gene expression (Fig. 3) revealed that the expression of PPARγ, C/EBPα, C/EBPβ, FABP4, and adiponectin was all significantly increased in groups 2, 3, 5, 6, and 7, with group 4 exhibiting minimal to no increase in some genes compared with the untreated group. The translation of these mRNAs into protein was subsequently verified by western blot analysis (Fig. 3D). The data confirmed that the expression of adipogenic markers, PPARγ and FABP4, was consistent with the mRNA expression patterns, with a marked elevation in groups 2, 5, and 7, followed by groups 3 and 6, and to a lesser extent, group 4.
The effect of EF on lipid metabolism was determined by a qRT–PCR analysis of genes associated with de novo lipid synthesis pathways, including SCD1, SCD2, FAS, and ACC (Fig. 3E). EF markedly upregulated the expression of these genes in the early and intermediate treatment phases, which was consistent with the expression patterns observed for the lipogenic genes. These results demonstrate that EF significantly promotes adipocyte differentiation, particularly when administered at the early and intermediate stages. The consistent findings between lipid staining, mRNA expression, and protein expression suggest that EF is an effective agent for preventative therapy of obesity and highlights its efficacy at enhancing preadipocyte maturation and lipid synthesis.
C/EBP homologous protein (CHOP) and mitotic clonal expansion (MCE) are critical factors in the maturation of preadipocytes and the development of obesity [34]. CHOP is typically associated with the inhibition of adipocyte differentiation, whereas MCE is necessary for cell cycle reentry that precedes the differentiation process [35]. With these roles in mind, we used the most effective concentration of EF identified from previous assays to conduct a time-dependent study on gene expression modulation during adipogenesis.
EF treatment suppressed CHOP mRNA expression and concomitantly elevated the mRNA levels of key adipogenic transcription factors and enzymes including PPARγ, C/EBPβ, and C/EBPα (Fig. 4A). A western blot analysis confirmed that while PPARγ protein levels were increased, CHOP protein expression was significantly attenuated in a time-dependent manner when exposed to 30 µg/mL of EF, with HSP90 serving as a loading control (Fig. 4B).
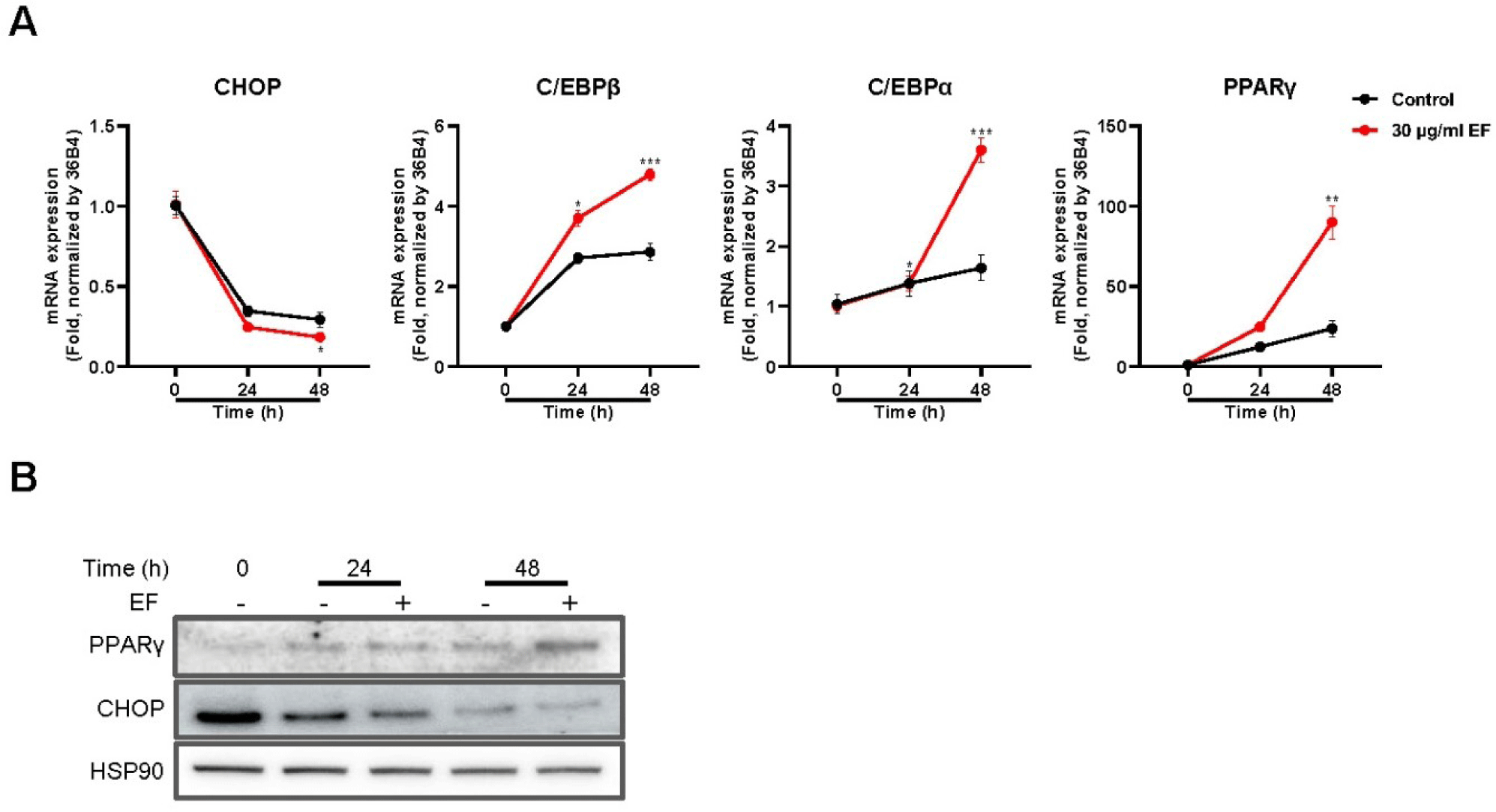
The cell cycle is an important process as it facilitates the MCE necessary for preadipocyte differentiation [36]. Upon examining the effect of EF on cell cycle-associated genes in 3T3-L1 preadipocytes, a distinct expression pattern was evident (Fig. 5A). Cyclin E mRNA levels increased at 24 h and decreased in the 48 h treatment group. A consistent biphasic pattern of mRNA expression was observed for cyclin-dependent kinase 2 (CDK2), whereas reduced cyclin D1 mRNA levels were observed compared with the control, followed by a slight increase at the 48-h time point compared with the 24-h mark. In addition, EF activated MCE during adipogenesis along with an increase in cell number (Figs. 5B and 5C). These results suggest that EF exerts a dual modulatory effect on adipocyte differentiation by downregulating CHOP, a negative regulator of adipogenesis, and inducing initial increases in cell cycle progression, which is necessary for preadipocyte maturation (Fig. 5D). These findings highlight the potential of EF as an adipogenesis modulator, with implications for the treatment and prevention of obesity.
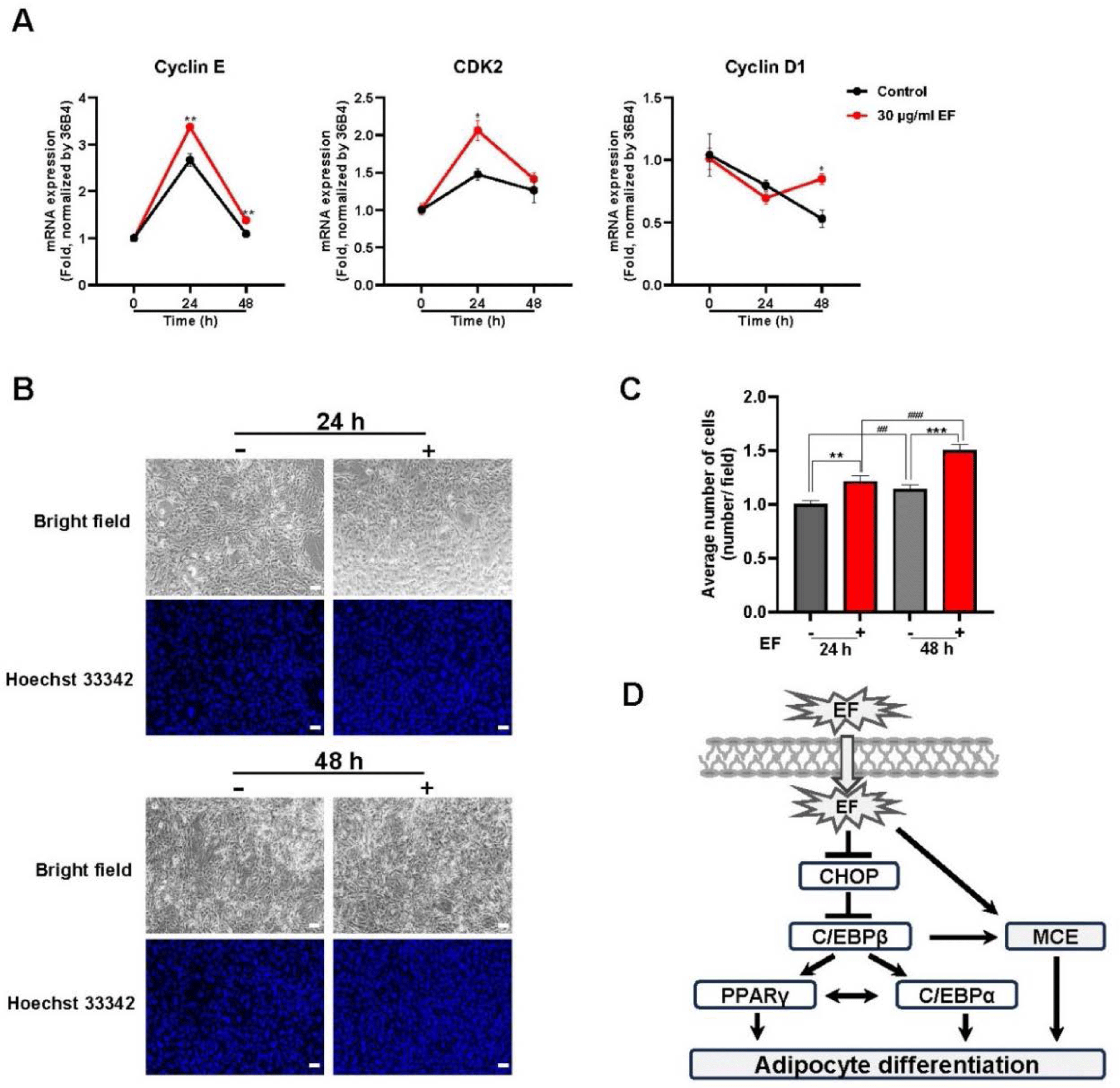
DISCUSSION
Obesity is a significant global public health issue that is often linked to a sedentary lifestyle and a high-calorie Western diet [37]. It is a risk factor for various metabolic disorders, such as type 2 diabetes mellitus, cardiovascular disease, and certain cancers [38,39]. Adipose tissue is involved in the onset and progression of obesity and influences lipid storage, energy expenditure, and the development of insulin resistance [40,41]. 3T3-L1 cells are a cornerstone for obesity research because of their ability to differentiate into adipocytes. Thus, they are an invaluable model for understanding the intricacies of adipogenesis and its role in obesity and diabetes [42,43].
Our results indicate that EF potently induces the maturation of 3T3-L1 preadipocytes, as evidenced by the accumulation of lipids by oil-red O staining. An upregulation of adipogenic and lipogenic genes and a few at the protein level was observed. In contrast, numerous studies reported the inhibition of adipocyte differentiation by inhibiting transcription factors, such as PPARγ and C/EBPα in 3T3-L1 cells [44]. Previous studies in 3T3-L1 cells showed that resveratrol treatment markedly reduced lipid accumulation and lowered the expression of C/EBPα, lipoprotein lipase (LPL), FAS, and SREBP 1c by activating AMPK [44]. The plant-derived alkaloid Berberine inhibits adipogenesis in 3T3-L1 cells by downregulating C/EBPα and PPARγ and up-regulating CHOP and basic helix-loop-helix family, member e41 (BHLHE41/DEC2), which is influenced by mitochondrial respiration [45]. The adipogenesis process involves a well-orchestrated transcriptional cascade, with key genes, such as PPARγ, C/EBPα, and C/EBPβ driving the differentiation of preadipocytes into mature fat cells, which is a process tightly linked to lipogenesis [46]. Adipogenesis involves elevated expression of adipogenic transcription factors, such as PPARγ and C/EBPα, which are the key adipogenic transcription factors that are activated during the differentiation of 3T3-L1 cells into adipocytes [47].
Natural products have long been studied for their therapeutic potential, and several, such as resveratrol, curcumin, and epigallocatechin gallate, have demonstrated significant effects on adipocyte differentiation and the regulation of adipogenic and lipogenic genes [48]. EF did not exhibit cytotoxic effects, which is an important consideration for its potential use as a therapeutic. Unlike some complete plant extracts that may contain toxic compounds, EF was well-tolerated by 3T3-L1 cells, even at high concentrations.
Furthermore, the upregulation of both adipogenic and lipogenic genes following EF treatment is noteworthy. The dose-dependent increase of PPARγ and FABP4 mRNA and protein underscores the role of EF in enhancing adipocyte differentiation. The effect of EF on 3T3-L1 preadipocyte differentiation is similar to that of well-established anti-diabetes drugs, such as pioglitazone and rosiglitazone from the thiazolidinediones group, particularly for promoting the expression of PPARγ and adiponectin [49]. Pioglitazone enhances peripheral insulin sensitivity. Pioglitazone up-regulates adiponectin receptor 2 in 3T3-L1 adipocytes, whereas rosiglitazone increases GLUT-4 expression in muscle cells [49]. Our results also highlight the unique properties of EF, including the inhibition of CHOP expression at the mRNA and protein level (Figs. 4A and 4B). CHOP, a negative regulator of adipocyte differentiation, inhibits the function of CEBP isoforms by forming dimers [50]. Its degradation enhances preadipocyte differentiation and the EF extract-mediated decrease in CHOP protein levels increases the function of C/EBPα, C/EBPβ, and PPARγ. These suggests that the role of EF in preadipocyte differentiation may mediated through CHOP protein degradation [35].
The specific molecule within EF that is responsible for enhancing preadipocyte maturation remains unknown. Eucalyptol, perillen, γ-terpinene, caryophyllene, limonene, p-cymene, 1,8-cineole, and carbofuran have been identified as the major compounds in EF essential oil. Of these, eucalyptol has a potential anti-obesity role as demonstrated by its inhibition of digestive enzymes, specifically α-amylase and pancreatic enzymes [51]. In addition, the antioxidant activity of eucalyptol has been reported [52]; however, the specific anti-diabetic effects of other compounds in the extract have not been evaluated. Further studies involving compound isolation and their activity are warranted.
The promotion of adipocyte differentiation by EF may play an important role in the development of new metabolic disease treatments. In the context of adipocyte biology, obesity is often associated with compromised insulin sensitivity and elevated blood lipid levels. This results in the ectopic accumulation of lipids in muscles, liver, and other organs, which can exacerbate metabolic complications [40]. By promoting the formation of healthy adipocytes, EF can maintain energy homeostasis and mitigate such complications. Enhancing healthy adipose tissue may provide a buffer for lipid storage to prevent lipid overflow into nonadipose tissues, which is a key factor in maintaining insulin sensitivity and metabolic health [53].
In conclusion, the ability of EF to modulate adipocyte differentiation and potentially support the development of healthy adipose tissue indicates its potential as a treatment for metabolic diseases. Future studies are needed to elucidate the precise mechanisms by which EF influences adipogenesis and lipogenesis and to determine its efficacy and safety in vivo. With the rising demand for safe and effective treatments for metabolic syndromes, natural products, such as EF, represent potential therapies.