INTRODUCTION
Myofibrillar proteins play a significant role in the development of gel matrix in meat emulsion products and have functionality as water and fat binder in restructured meat products [1]. Before emulsification, the water and fat molecules in the mixture or batter of restructured meat are not well homogenized. After emulsification and during heat-induced gelatinization, myofibrillar proteins develop matrix to bound water and fat molecules to form uniform and consistent gel [2]. In order to optimizing the development of emulsion gel, myofibrillar proteins should be extracted from the meat structure [3]. Myofibrillar proteins comprise of approximately 45% myosin group and 25% actin group and build approximately 50% to 60% of meat proteins. Myosin and actin, the major meat proteins, are salt-soluble and can retain water in the matrix of meat emulsion gel [4]. When heat is added, myofibrillar proteins are being partially denatured followed by irreversible aggregation, and eventually resulting a 3-dimensional gel matrix [5,6]. The pH and ionic strength of the solution used for myofibrillar protein extraction influence the extractability significantly. The optimum pH to extract myofibrillar proteins from meat structure is approximately 6.5 to 7.0 and the ionic strength should be more than 0.3 M [3,6]. Further, the solubility of myofibrillar protein in emulsion system will optimize the interactions between protein, fat, moisture and carbohydrate found in the emulsion system to form uniform emulsion gel matrix [7].
Elephant foot yam (Amorphophallus campanulatus) is a plant that is relatively close to konjac (Amorpphophallus oncophyllus). The tuber roots of these plants are rich in starch, cellulose and dietary fibers. However, they contain poisonous crystal calcium oxalate that should be remove prior to consumption. Soaking in salt solution followed by heat treatment can eliminate crystal calcium oxalate from elephant foot yam, but further processes, e.g. using enzyme, should be performed to remove crystal calcium oxalate and optimize the extraction of glucomannan from konjac [8]. The flour of elephant foot yam can be utilized as binder, extender or filler in emulsion-type food as it contains glucomannan [9,10]. The starch and glucomannan from elephant foot yam will develop a firm gel when water and heat are added [11]. Therefore, elephant foot yam flour has a potency to be used as binder in meat emulsion products.
Starch and glucomannan from elephant foot yam may have a functionality to develop a good meat emulsion gel. Liu et al. [12] reported that the hydroxyl group in konjac oligo-glucomannan are abundant and can improve the functionality of meat products. Restructured meat products are made from ground meat, added with other ingredients that have function as emulsifier, binder, extender, and flavor enhancer. In the process of texture development, an ingredient that can absorb and retain moisture is important to be added in meat emulsion to prevent syneresis. Many studies have been reported that the utilization of konjac glucomannan, carrageenan, and alginate in meat products can enhance the acceptability of the products as those ingredients can improve texture [13–15]. There is limited information regarding the utilization of elephant foot yam flour in meat products. Therefore, the objective of this study was to observe the interactions between beef salt-soluble proteins extracted using salt solution at different concentrations and elephant foot yam flour in the development of gel matrix.
MATERIALS AND METHODS
The fresh beef from round cut was purchased 5 h post slaughter and stored at –20°C for 24 h. The frozen meat was thawed at 4°C overnight, trimmed of excess fat and visible connective tissue, ground through 6-mm plate grinder and stored at 4°C for 12 h. To prepare elephant foot yam flour, a method described by Faridah [16] was used. The skin of elephant foot yam was peeled, the peeled yam was washed twice in flowing water and sliced into 2–3 mm thick. The chips were soaked in salt solution (5% NaCl in water, w/v) for 5 min to remove crystal calcium oxalate, rinsed in flowing water twice and oven-dried at 50°C for 24 h, ground and sieved through 100 mesh-sieve, packed and stored at room temperature.
Beef protein was extracted from ground beef added with phosphate buffer (pH 7.0, 4°C) with a ratio of 1:10 according to a method described by Choi et al. [6] with modification. The mixture was then homogenized at 2,500 rpm for 2 min. The homogenized mixture was centrifuged at 4,000×g for 25 min at 4°C, the supernatant was removed, and the pellet was stored for 24 h at 4°C. The pellet was added with NaCl at different concentrations (0.5%, 1.0%, 1.5%, and 2.0%, w/w) and 20 mL of phosphate buffer (pH 7.0, 4°C), homogenized at 2,500 rpm for 2 min, and centrifuged at 4,000×g for 25 min at 4°C. The supernatant was collected and stored at 4°C for 12 h prior to analysis.
The pH of the supernatant was measured according to Hashemi and Jafarpour [17] in duplicate using a calibrated pH meter. Protein concentrations of the supernatant was determined using Bradford’s method and expressed as μg/mL [18]. The protein fractions were separated by 1D sodium dodecyl sulfate polyacrylamide gel electrophoresis (SDS PAGE) as described by Sambrook and Russell [19]. The sample aliquots (2 mg/mL in phosphate buffer) were mixed with SDS gel loading buffer (50 mM Tris-Cl, 100 mM dithiothreitol, 0.004% blue bromophenol, 10% glycerol, and 2% SDS). The mixture was denatured at 90°C for 3 min and loaded at 10 µg protein per lane onto the 10% polyacrylamide gels (Mini PROTEAN 3, Bio-Rad, CA, USA) running in Tris-glycine buffer at 200 V constant voltage for 40 min at room temperature. Gels were stained using Coomassie Brilliant Blue R-250 followed by destaining with 40% methanol and 7% acetic acid in distilled water. The SDS PAGE gels were scanned and the relative molecular weight of the protein was determined using standard marker [6,20].
Salt-soluble protein and elephant foot yam flour were mixed, homogenized at 2,500 rpm for 2 min, and incubated at 20°C for 10 min. The mixture was gelatinized at 90°C for 20 min and cooled down at 4°C for 12 h. The pH, gel strength, instrumental color, proximate composition, and microstructure of the gels were then analyzed.
Gel sample (5 g) was added with 20 mL of distilled water and homogenized at 2,500 rpm for 2 min. The pH of the slurry was determined in duplicate using a calibrated pH meter.
The sample was sliced into 10 mm thick with 25 mm diameter and placed on the center of the plate. The penetration force representing gel strength (N) was measured using a texture analyzer XT plus (Stable Micro Systems, Goldaming, UK). The gels were compressed twice to 25% of original height at a constant speed of 1.0 mm/s using a P35 probe (35 mm cylinder). The pre-test and post-test speed were 1.0 mm/s and 10 mm/s, respectively [21].
The instrumental surface color of the gels was recorded by measuring International Commission on Illumination’s system for lightness (CIE L*), redness (CIE a*), and yellowness (CIE b*) using a chroma meter (CR-400, Konica Minolta, Tokyo, Japan). The light source of illuminant C (2° observer) with 8 mm aperture and attached-closed cone was calibrated using a white plate (Y = 93.6, X = 0.3134, y = 0.3194).
SEM (TM3000, Hitachi High-Technologies, Tokyo, Japan) was used for the observation of the microstructure of the gels. Sample was sliced into 1–2 mm thick and fixed with 2.5% glutaraldehyde in 0.2 M phosphate buffer (pH 7.0) for 2 h, washed and soaked in distilled water for 1 h, and serial dehydrated with ethanol in distilled water (50%, 60%, 70%, 80%, 90%, and 100%, v/v) for 1 h each. The dried sample was put onto holder, coated with gold, and visualized under SEM at magnification of ×100 [17].
Moisture, crude protein, crude fat, and ash content were determined using AOAC official methods [22]. Moisture content was determined by drying the samples in an oven at 105°C for 24 h. Crude fat content was determined by ether extraction using a Soxhlet system. Nitrogen content was determined using the Kjeltec system (2200 Kjeltec Auto Distillation Unit, Foss, Hillerød, Denmark). Crude protein was calculated as nitrogen content multiplied by 6.25. Ash content was determined by burning the samples in a muffle furnace at 550°C for 8 h.
This study employed a randomized block design and each replication being treated as a block. In first study, the effect of salt concentration (0.5%, 1.0%, 1.5%, and 2.0%, w/w) on the pH and salt-soluble protein concentration of beef extract was observed. One-way analysis of variance (ANOVA) was employed to determine the effect of salt concentration. In second study, two-way ANOVA was employed to determine the effect of salt concentration and the addition level of elephant foot yam flour (5%, 10%, and 15%, w/w). Significant differences (p < 0.05) of mean values among treatments were determined using Duncan’s multiple range test.
RESULTS AND DISCUSSION
Table 1 shows that pH and salt-soluble protein concentration of beef extract did not differ significantly among treatments. The pH of the beef extract ranged from 6.90 to 6.98 as the result of using phosphate buffer (pH 7.0) for extraction. Further, the pH of the beef used in this study was 5.96, which is relatively high for normal meat (pH 5.5–5.6) as the result of early collection (5 h post slaughter and being frozen 24 h). The observed concentration of salt-soluble proteins ranged from 2.96 to 3.84. The observed pH (6.90–6.98) supported the extraction of salt-soluble protein in present study.
Salt concentration (%) | pH | Protein concentration (μg/mL) |
---|---|---|
0.5 | 6.98 ± 0.02 | 2.96 ± 0.03 |
1.0 | 6.96 ± 0.11 | 3.01 ± 0.04 |
1.5 | 6.92 ± 0.10 | 3.55 ± 0.04 |
2.0 | 6.90 ± 0.05 | 3.84 ± 0.05 |
Meat proteins, that are soluble in a solution with ionic strength more than 0.3 M, are categorized into salt-soluble (myofibrillar) protein. These proteins comprise approximately 50% to 60% of meat protein, e.g. actin and myosin [4]. Çarkcioğlu et al. [23] mentioned that myofibrillar proteins play a critical role in texture development for both raw and processed meat. Further, postmortem conditions, such as pH and the use of salt in processing, influence the extractability of myofibrillar protein. Eady et al. [20] reported that the solubility of protein declines when the pH is around its isoelectric point (5.4–5.5). The former condition (pH 5.4–5.5) leads the myofibrillar proteins structure cannot be unfolded, thus limiting the extraction of actin and myosin. The addition of salt optimizes the extraction of myofibrillar proteins and eventually develop a good texture of protein gel [24]. These suggest that salt concentration ranged from 0.5% to 2.0% showed comparable results in the extraction of myofibrillar proteins of beef with pH of 5.96.
The salt-soluble proteins, that were extracted from beef round using salt solution at different concentrations, are shown in Fig. 1. The bands in lane 1 to 6 were identified as myosin group as the molecular weight ranged from 68 kDa to 240 kDa, while the bands in lane 7 were identified as actin group with molecular weight of 45 kDa. The SDS PAGE gel shows that the bands for myosin group were thicker than those of actin group. Price and Schweigert [25] mentioned that myosin group comprises approximately 55% to 60% of myofibrillar proteins. Choi et al. [6] added that myofibrillar proteins with molecular weight of 205 kDa are classified as heavy chain myosin, while those with molecular weight of 45 kDa are actin. In more details, the molecular weight of heavy meromyosin, light meromyosin fraction I, light meromyosin, tropomyosin, and G-actin are 232, 120, 96, 68, and 47 kDa, respectively [25]. Therefore, the use of salt with concentration ranged from 0.5% to 2.0% was effective to extract myosin, the major protein group of myofibrillar proteins, and the differences in salt concentration did not show any significant effect on fragmentation pattern.
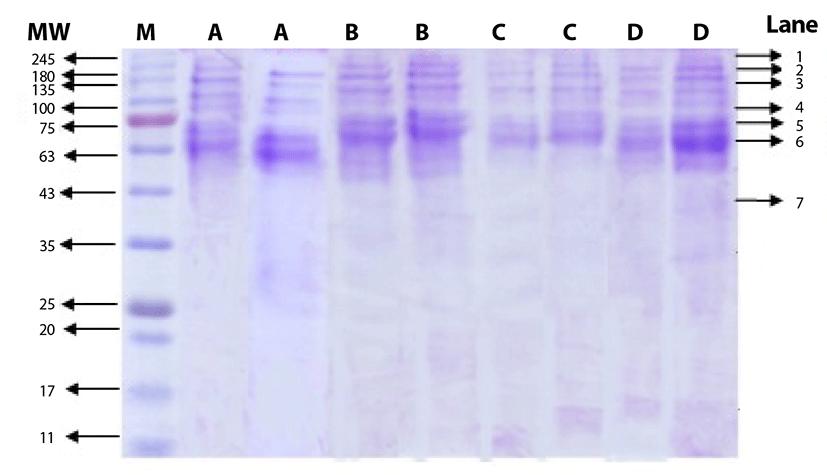
Table 2 shows that salt concentration did not affect the pH and instrumental color of the gel made from beef salt-soluble protein and elephant foot yam flour. However, gel strength was significantly influenced by both salt concentration (p < 0.01) and the addition of elephant foot yam flour (p < 0.01). On the other hand, the addition of elephant foot yam flour influenced the pH (p < 0.05) and instrumental color of the gels (p < 0.05). The interaction effect was observed only for gel strength (p < 0.01). These indicate that elephant foot yam flour showed greater impact on the characteristic of the gel than salt used for extraction.
The addition of elephant foot yam flour increased the pH of the gel resulting an increase of gel strength. Although there was no significant difference on pH found between the gels that were added with 5% and 15% elephant foot yam flour, the texture of the gel that made from 15% elephant foot yam flour was significantly firmer than that made from 5% elephant foot yam flour. These suggest that the more the elephant foot yam flour was added, the higher the pH and the firmer the texture of the gel were resulted. Eady et al. [20] reported that elevated pH in meat emulsion system affects the unfolding of meat protein, resulting an increase of water absorption. The addition of elephant foot yam flour would result in more water absorption and retention in the emulsion system. Furthermore, the gelatinization of starch and protein complex will form a swelling 3-dimensional gel with firm texture.
The interaction effect between salt concentration and the addition level of elephant foot yam flour is shown in Table 2. High salt concentration used in this study (1.5% and 2.0%) enhanced the strength of the gels made from 15% elephant foot yam flour. Although the amount of protein extracted in salt solutions at different concentrations was not different statistically, the concentration of protein in solutions containing salt at 1.5% and 2.0% was numerically higher than that in lower salt concentration. These suggest that more myofibrillar proteins interacted with starch from elephant foot yam flour and formed stronger gel matrix in higher salt groups. Hasemi and Jafarpour [17] mentioned that an increase of protein level in frankfurter resulted in firmer texture. The role of elephant foot yam flour in this case is critical too as this ingredient contains starch with amylopectin level of 74.66%, dietary fiber of 26.87%, and glucomannan of 1.02%, which has functionality as a filler and supports the development of gel matrix in meat emulsion [26]. Chen et al. [27] mentioned that polysaccharide can well interact with protein in biological system including in food. That interaction will eventually determine the characteristic of the food. In this study, the mixture of elephant foot yam flour and beef salt-soluble protein would result in the good interactions between myofibrillar protein, starch, glucomannan and water that form stable gel matrix.
The micrographs revealing the microstructure of the gels made from a mixture of salt-soluble protein and elephant foot yam flour at different addition levels are shown in Fig. 2. The micrographs No. 1, 2, 3, and III show comparable and uniform microstructures in terms of pore size and gel matrix as these treatment groups used similar amount of elephant foot yam flour (15%), regardless the differences in salt concentration used for beef protein extraction. These indicate that salt concentration used in this study did not contribute significantly to the microstructure of the gel made from salt-soluble protein and elephant foot yam flour. Meanwhile, the micrographs No. I, II, and III show different microstructure of the gels as these gels were made from different addition levels of elephant foot yam flour with similar amount of salt (2.0%) used for beef protein extraction. Chen et al. [27] mentioned that the differences in microstructure would be used as evidence to describe the differences in functionality of the gel matrix. The smallest pores and more uniform gel matrix were observed in micrograph III or gel made from 15% elephant foot yam flour in comparison with micrograph I and II.
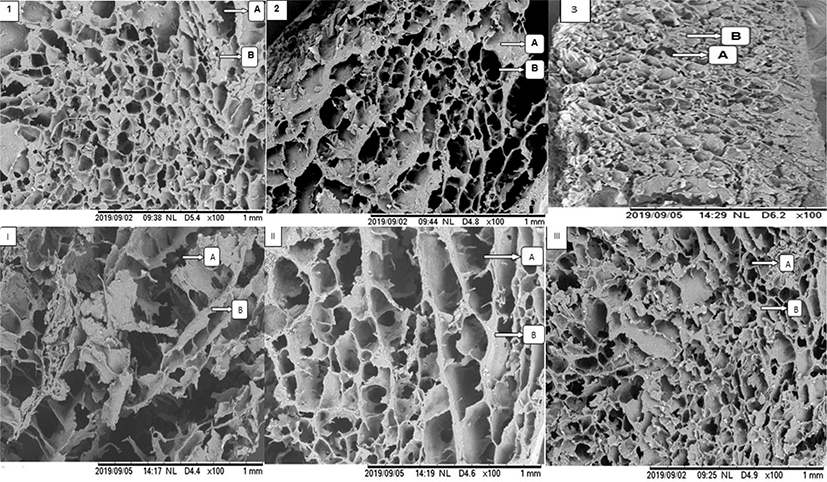
The variety in size of the pores in micrograph I explains that there was a syneresis occurred during gelatinization. The syneresis was a result of weak interactions between salt-soluble protein, elephant foot yam flour and water as the addition of elephant foot yam flour was lower in this group than in other groups shown in micrograph II and III. These also suggest that the higher the addition level of elephant foot yam flour was added, the more uniform gel matrix and the smaller pores were formed in the gel. The micrograph 1, 2, 3, and III also explain that beef salt-soluble proteins well interact with elephant foot yam flour during gelatinization at 90°C, when the addition level was optimized at 15%. The starch from tuberous roots flour is hydrophilic and thus can absorb and retain water in the mixture during gelatinization besides binding the hydrogen bond of myofibrillar proteins [28]. Therefore, syneresis was inhibited when the addition of elephant foot yam was 15% w/w.
The differences in salt concentration did not affect the instrumental color of the gels. However, the higher the addition level of elephant foot yam flour was, the darker the color (higher L*, a*, and b*) of the gel was observed (p < 0.05). These results are in line with previous studies using konjac glucomannan, carrageenan, and alginate [13–15]. The elephant foot yam flour used in this study was originally light brown. Thus, elephant foot yam flour decreased L*, a*, and b* values of the gels.
Salt concentration did not affect the moisture, crude protein, crude fat, and ash content of the gels made from beef salt-soluble proteins and elephant foot yam flour (Table 3). In contrast, the addition level of elephant foot yam contributed significantly on moisture, crude protein, and ash content. Crude fat content of the gels, however, was not affected by either salt concentration used for the extraction of myofibrillar proteins or addition level of elephant foot yam flour. The addition of elephant foot yam flour increased moisture, crude protein, and ash content of the gels (p < 0.01). The low proportion of moisture in 5% group were caused by syneresis during gelatinization. Elephant foot yam flour is the source of carbohydrates such as starch and glucomannan, which are able to bound with water molecules during gel formation [29]. The crude protein, ash and carbohydrate content of the flour used in this study were 9.49%, 10.86%, and 71.98%, respectively (data are not shown). Starch and dietary fiber can replace and mimic fat in the development of meat emulsion gel matrix [30–32]. Therefore, elephant foot yam flour added the functionality of the gel as it can retain moisture and develop compact gel matrix.
CONCLUSION
Elephant foot yam flour well interacted with beef salt-soluble proteins during gelatinization to form gel and the effect was level-dependent. The use of NaCl for beef myofibrillar protein extraction was not level-dependent, regardless the differences in salt concentration used in current study (0.5%–2.0%). The addition of elephant foot yam flour at 15% (w/w) to beef protein extracted using NaCl at 2.0% (w/w) could develop uniform meat emulsion gel with small pores. Therefore, elephant foot yam flour shows potency to be used in meat emulsion products as binder.