INTRODUCTION
Volatile fatty acids (VFA), the main metabolic product of anaerobic fermentation in the rumen, especially acetic acids play a significant role in milk performance. However, the carbon dioxide (CO2) and hydrogen (H2), produced along with the acetate and butyrate, have a negative impact on the environment by contributing methane (CH4) production which has 20 to 50 times higher greenhouse effect than that of CO2 [1] and considered as dietary energy losses (2%–12%) from ruminants [2]. The ruminants produce VFA, H2, CO2, and ammonia (NH3) after fermentation of feeds by microorganisms such as bacteria, protozoa and fungi in their rumen [3]. The CH4 is produced by utilizing H2, accumulated inside the rumen, by methanogens. Therefore, it is needed to find out suitable microorganisms that are able to compete with methanogenic bacteria in the rumen for H2. Reductive acetogens, anaerobic microorganisms, produce acetic acid through the pathway of acetyl-coenzyme A (CoA), is a process which requires H2 atoms and thus competing with CH4 production by methanogens [4]. So, reductive acetogenesis is considered as an alternative pathway of hydrogen-utilization that reduce methanogenesis in the rumen and could be a probable approach to reduce greenhouse gas emissions from ruminants.
Milk performance is the prime target in dairy farmers along with the reduction of greenhouse gas from animal sources. The cows will produce larger quantities of milk with better quality only with the receiving of proper nutrition. Milk fat is synthesized by using acetate and butyrate from rumen whereas lactose is synthesized by propionate, lactate and amino acid [5]. Acetic acid accounts for 60%–70% of total VFA production which is mostly used for energy production and milk fat synthesis. In addition, various feed additives including probiotics have been used to improve the milk yield and quality of dairy cows along with blood profile [6]. Reductive acetogens, present in the rumen, are able to increase acetate production which leads to increase milk fat percentage as well as reduce enteric methane production which subsequently prevent energy loss from dairy cows. Though several reductive acetogens have been so far identified and implemented to improve in dairy production however, still needed to find more suitable strains for better performance. Therefore, reductive acetogens can be used as a good source of probiotics which will improve performance of lactating dairy cows.
Because acetogens can be the potential inhibitors to methanogenesis, this study was conducted to evaluate the effect of the ruminant’s origin reductive acetogens on in vitro rumen fermentation with emphasis on methane reduction. Consideration of lactating performance, this study targeted another objective to investigate the effects of reductive acetogens on the milking performance such as milk production, milk fat, milk protein and SCCs as well as the blood profile in dairy cattle supplemented as feed additives.
MATERIALS AND METHODS
The studies were conducted at the Sunchon National University (SCNU) animal farm and in the ruminant nutrition and anaerobe laboratory, Department of Animal Science and Technology, SCNU, Jeonnam, South Korea.
Three Korean native goats (KNG; Capra hircus coreanae collected from Hwasun, South Korea) and 3 ruminally cannulated Holstein cows were used as experimental animal. The KNGs, having body weight of 43.93 ± 1.22 kg, were offered timothy and commercial concentrate at a rate of 2% of body weight twice daily. And, 48-months old ruminally cannulated Holstein cows, having body weight of 600 ± 47 kg, were fed concentrate feed and Italian ryegrass at a ratio of 2:8 twice a day. The rumen fluids were collected from KNGs after slaughtering and from cannulated Holstein cows 2hrs after feeding, respectively. Immediately after collection, the rumen fluids were strained through the four layers of surgical gauze and subsequently placed in amber bottles with an oxygen free headspace. Then the rumen fluid was sealed and immediately transferred to the laboratory by maintaining 39°C temperature.
For initial enrichment, AC-B1 medium (9.5 mL), described by Le Van et al. [7], was dispensed into 30 mL Hungate tube under oxygen-free CO2 (20%) and N2 (80%) gases, sealed with butyl-rubber septum and aluminum crimp cap. AC-B1 medium contained (per 1,000 mL) the following: KH2PO4 (0.28 g), K2HPO4 (0.94 g), NaCl (0.14 g), KCl (0.16 g), MgSO4 · 7H2O (0.02 g), NH4Cl (0.5 g), CaCl2 · 2H2O (0.001 g), trace mineral solution (10 mL), vitamin solution (10 mL), yeast extract (0.5 g), NaHCO3 (6.0 g), reducing agent [2.5% {wt/vol} each cysteine hydrochloride · H2O and Na2S · 9H2O, pH 10.0] (10 mL), clarified bovine rumen fluid (100 mL), BES [sodium salt; filter sterilized] as indicated (20 mL), and resazurin (0.001 g). After autoclaving for 15 min at 121°C, strained rumen fluid (0.5 mL) were inoculated into the medium and incubated for 7 days with horizontal shaking at 120 rpm and maintained 39°C temperature. After an initial enrichment, the culture was diluted serially from 10−2 to 10−9, inoculated into AC-B1 agar medium and incubated for another 7 days. The visible colonies were subsequently isolated and inoculated into broth medium and finally incubated for more one week with horizontal shaking at 120 rpm and 39°C temperature. The isolation step was repeated to obtain pure colonies.
The colonies were isolated from AC-B1 agar medium and their DNA were extracted. The 27F (5´-AGAGTTTGATCCTGGCTCAG-3´) and 1492R (5´-GGTTACCTTGTTACGACTT-3´) primers [8] were used to amplify 16S ribosomal RNA gene (16S rDNA), whereas reductive acetogenesis-related genes were used for the effective separation and identification of the target bacteria (reductive acetogens). The separation was made through the detection of the specific formyltetrahydrofolate synthetase (FTHFS) gene which was described by Leaphart and Lovell [9] using the primers FTHFSf (5´-TTY ACW GGH GAY TTC CAT GC-3´) and FTHFSr (5´-GTA TTG DGT YTT RGC CAT ACA-3´).
For DNA sequencing, the purified PCR products of 16S rDNA were sent to the Macrogen (Macrogen Inc. Korea, 10F, 254 Beotkkot-ro. Geumcheon-gu, Seoul 08511, Korea). The SeqMan program (DNA Star, Lasergene software, Madison, WI, USA) were used to assemble the sequenced fragments. The obtained gene sequences were compared with 16S RNA gene sequences which were available in GenBank using the program BLAST (http://www.ncbi.nlm.nih.gov/BLAST/) and EzTaxon (http://147.47.212.35:8080/index.jsp). The approximate phylogenetic affiliations were determined through aligning the gene sequences with those sequences of closely related species by using CLUSTAL W version 1.6. The neighbor-joining (NJ) method and pairwise gap removal techniques were used to construct the phylogenetic tree whereas distance matrices were calculated according to Kimura [10]. Lastly, two-parameter NJ method with in PHYLogeny Inference Package (PHYLIP package) which was employed and the bootstrap analysis was done by resampling the data (1,000 times) in order to evaluate the stability of phylogenetic tree. Only the bootstrap values larger than 50% were expressed on the internal nodes.
The isolated acetogens strains from the rumen were compared to Eubacterium limosum ATCC8486 as standard acetate-producing bacteria. All acetogens, isolated from ruminant sources, were sub-cultured and inoculated in to RM02 medium in order to characterize their fermentation parameters [11]. RM02 medium contained (per 1,000 mL) the following: dH2O (950 mL), (NH4)2SO4 (0.6 g), K2HPO4 (1.4 g), L-cysteine-HCl (0.5 g), KCl (1.5 g), resazurin [0.1% solution] (4 drops), NaHCO3 (4.2 g), selenite-tungstate solution (1 mL), trace element solution (1 mL), and vitamin solution (1%). The media were dispensed under O2-free CO2 (20%) and N2 (80%) gases. The cultures were cultivated without (Con) or with the addition of mix gas (20% CO2 and 80% H2) and glucose (5 mM) and then incubated at 39°C temperature in a shaking incubator with 120 rpm for 4 days and subsequently the acetic acid production was determined.
Upon arrival of rumen fluid samples in the laboratory, rumen fluid was mixed with prepared buffer solution, recommended by Russell and Van Soest [12] at a ratio of 1:2 and adjusted pH 6.7. All serum bottles (120 mL in size), containing either soluble starch or casein powder substrates (1% DM), were dispensed with 50 mL of prepared medium anaerobically under O2-free N2 bubbling and sealed with rubber stoppers and aluminum caps immediately. The serum bottles were then incubated in a shaking incubator at 120 rpm and 39°C temperature for 24 h. The experimental groups included a negative control (without acetogens), a positive control (inoculated with 1% of the standard acetogenic bacteria, E. limosum ATCC8486), and the 4 experimental treatments (bottles inoculated with 1% of either of the isolated acetogens).
The total gas (TG) production was measured by using a press and sensor machine (Laurel Electronics, Inc., Costa Mesa, CA) and the pH was measured by using a Pinnacle series M530p meter (Schott instruments, Mainz, Germany) just after uncapping the bottles. The method described by Chaney and Marbach [13] was followed to measure ammonia nitrogen (NH3-N) concentration in the rumen fluids. The CH4 and CO2 emitted during the incubation period were measured by using Gas chromatography (Agilent Technologies HP 5890). The CH4 production was estimated using the formula described by Orskov and McDonald [14]. VFAs production were analyzed by using high-performance liquid chromatography (HPLC; Agilent Technologies 1200 series) following the method described by Tabaru et al. [15].
Holstein cows (n = 14) having initial body weight of 450 ± 50 kg, milk yield 28.63 ± 3.65 kg/d and DIM (Day in Milk) 115 ± 60 d, were blocked into 2 (two) groups by parity, DIM, and milk yield. The average parity was 2.5 and lactation stage was 2 to 6 months. Cows in each block were then randomly allocated to one of the two treatment diets (a randomized complete block design). The experiment was conducted in summer season through a period of 60 days consisting 20 days of adaptation and 40 days of sample collection. Due to higher acetate as well as lower methane producing ability of Proteiniphilum acetatigenes GA03 strain isolated through in vitro trial, which was stored in the Korea culture center of microorganisms, Seoul, Korea as SROD1 (KCCM11219F), was used as probiotics for in vivo experiment. Cows were separated into 2 groups such as control and treatment GA03. All cows were fed the same diet as a total mixed ration (TMR; Table 1) ad libitum, twice a day at 0800 h and 1900 h, allowing for 5% refusals throughout the study, and had free access to water and mineral. After 20 days feed adaptation, only the treatment group received probiotics GA03 as diet supplement daily with TMR (Table 1) and the feed intake was recorded from day 21.
1) Vitamin premix contained the following amount which was diluted in cellulose (g/kg premix): L-ascorbic acid, 121.2; DL-α-tocopherol acetate, 18.8; thiamin hydrochloride, 2.7; riboflavin, 9.1; pyridoxine hydrochloride, 1.8; niacin, 36.4; Ca-D-pantothenate, 12.7; myo-inositol, 181.8; D-biotin, 0.27; folic acid, 0.68; p-aminobenzoic acid, 18.2; menadione, 1.8; retinal acetate, 0.73; cholecalciferol, 0.003; cyanocobalamin, 0.003.
The dry matter (DM), crude protein (CP) and ether extract (EE) contents of the experimental diet were analyzed according to the guidelines of the Association of Official Analytical Chemists [16]. The neutral detergent fiber (NDF) and acid detergent fiber (ADF) were measured by using an Ankom fiber analyzer (Ankom Tech. Corp, Fairport, NY, USA) following the protocol described by Van Soest et al. [17].
The isolated acetogen bacterial strain GA03 (Table 2), was anaerobically cultivated at 39°C with 100 rpm for 48 h. Mixed wheat bran and corn gluten feed (6:4) supplemented with 1:1 ratio of cultivated GA03 served as treated (TRT). For the purpose of treatments with acetogens, 10% GA03 acetogen inoculum was inoculated with the basal feed and incubation for three days with after fermentation cell unit of 4.18 Log CFU/g. The GA03 acetogen treated group received the diet supplemented with GA03 as a feed rate of 1% supplementation however, control group received only 1% supplementation of wheat bran and corn gluten feed (6:4) without GA03 inoculum. The prepared probiotic mixture was supplied on the top of the basal feed for immediately feeding as early as possible.
Cows were individually milked twice daily in their stalls at 0600 h and 1700 h and recorded daily milk production. The milk samples were collected on day 0 and day 60 and the milk fat, protein and somatic cells were measured by using a Milko-Scan (Foss Electric, Denmark).
Blood samples were collected before 30 minutes of feeding from the jugular vein on day 60. Blood samples were immediately transferred to the tube containing a solution of disodium EDTA and placed on ice. After centrifugation for 20 minutes, 1,800×g at 4°C (Labogene 1248, Korea), the plasma was transferred to a storage tube and labelled with the date and the animal identification number and subsequently analyzed the fresh sample or stored the sample at −20°C until analysis. An automatic blood analyzer (Express Plus, Ciba-Corning, CA, USA) was used to determine total protein, albumin, blood urea nitrogen (BUN) and glucose concentrations. The non-esterified fatty acid (NEFA) concentration was measured by HPLC.
All statistical analyses were carried out by using Statistical Analysis Systems (SAS version 9.1) [18]. The data were analyzed by analysis of variance (ANOVA) using the general linear model (GLM) under a randomized completely block design. Duncan’s Multiple Range Test (DMRT) was used to categorize differences among specific treatments in in vitro while t-test was used for in vivo data analysis. The significant differences were considered only when p < 0.05.
RESULTS
Forty-nine colonies were isolated and identified from the rumen samples. Among them, four identified species had formyltetrahydrofolate synthetase (FTHFS) gene and considered to be acetogenic bacteria (Table 2). All these phylotype sequences showed 95%–100% similarity to the phylogenetic tree including namely DA02 (Proteiniphilum acetatigenes DA02), GA01 (P. acetatigenes GA01), GA02 (Alkaliphilus crotonatoxidans GA02), and GA03 (P. acetatigenes GA03) (Fig. 1). These four reductive acetogenic bacteria isolated from the rumen and positive control E. limosum ATCC8486 were compared for acetic acid production. The DA02, GA01 and GA03 acetogeic bacteria, except GA02 from the rumen, showed higher acetic acid production than positive strains E. limosum ATCC8486 (Table 3). With addition of glucose on the media of GA03, it had the highest (p < 0.05) acetate production (11.31 mM).
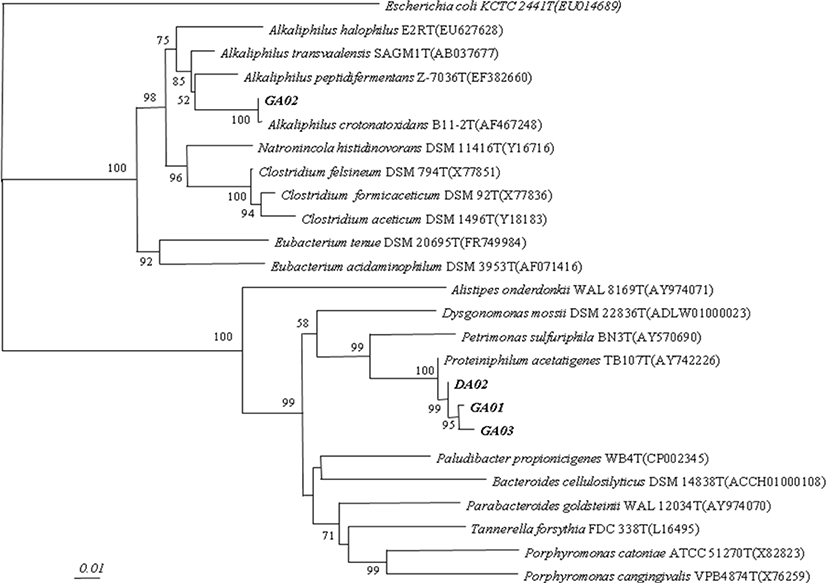
The pH, total gas, and VFA concentration of in vitro experiment are presented in Table 4. The total VFA was significantly (p < 0.05) higher in E. limosum ATCC8486 and other treatment groups than the control in both substrates, however, the pH was higher in the control than the other groups. The acetic acid production was higher (p < 0.05) in all 4 isolated acetogens in the soluble starch and casein than in the control and positive treatments. The isolated acetogens produced almost similar amount of propionate compare to positive group however higher (p < 0.05) than control (18.95 mM) while using soluble starch as substrate. In case of casien substrate, the GA03 produced lower acetic acid (17.57 mM) than other probiotic treated groups however, similar to positive group (17.59 mM) and significantly (p < 0.05) higher than the control (13.99 mM). Butyric acid was measured only in soluble starch however not detected in case of casein. The in vitro methane emission results are presented in Fig. 2. The amount of methane was lower (p < 0.05) in E. limosum ATCC8486 and GA03 groups than other probiotic groups viz. DA02, GA01, and GA02 with casein substrate. In case of starch substrate, methane production was lower (p < 0.05) in acetogens treated groups compare to control and E. limosum ATCC8486. In all experimental groups, the methane production was higher in case of casein substrate compared to starch. E. limosum ATCC8486 produced higher and lower CH4 with starch and casein, respectively. In contrast, GA01 produced higher and lower CH4 with casein and starch, respectively. However, GA03 produced lower CH4 with both starch and casein substrates.
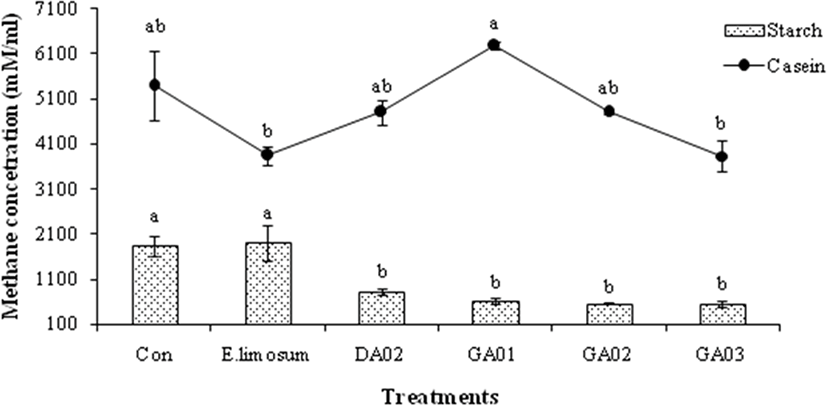
The results of effects of reductive acetogenic bacterial strain GA03, supplemented with diet, on feed intake and lactating performance such as milk yield and milk compositions of dairy cows are presented in Table 5. The feed intake of control and treated group was 19.18 kg/d and 20.27 kg/d respectively. Initial milk production amounted to 30.90 kg in control whereas 26.37 kg in GA03 treated group (p < 0.5) which was decreased to 25.83 kg and 23.f97 kg, respectively after 60 days. The milk fat was decreased during the 60 days’ trial. The control decreased from 3.83% to 3.59% and GA03 decreased from 3.69% to 3.58%. However, the decrease (p < 0.05) was less in GA03 than control (0.11% vs 0.24%). The milk protein in control was decreased from 3.41% to 3.14% however, increased from 3.28% to 3.32% in GA03. Blood parameters of dairy cows fed with reductive acetogenic bacteria was shown in Table 6. Glucose concentration was higher (p < 0.05) in GA03 group compare to Control group (70.11 vs 67.47 mg/dL). Likewise, TP (g/d) production was higher (p < 0.05) in GA03 group, however BUN (mg/dL), and NEFA (μEq/L) production were higher (p < 0.05) in Control group.
DISCUSSION
Reductive acetogens can produce acetate from hydrogen and carbon dioxides using the acetyl-CoA pathway which can compete with methangenesis in ruminants. In the present study, 4 reductive acetogens were isolated and identified from ruminants which were then used for in vitro experiments. In this study, positive control (E. limosum ATCC8486) and acetogens treated groups had significantly (p < 0.05) lower pH and higher total gas productions compared to control group with both substrates. However, the total gas production was higher with starch than casein, which may be due to the presence of higher content of easily fermentable starches viz. sugars, or hemicelluloses as substrates which was available for rumen microbes to produce more gases. This prediction is closely related to the digestibility of organic matter, CP, and ash contents of feeds [19]. In the present study, supplementation of isolated acetogens had comparable acetate production and they are significantly (p < 0.05) higher than E. limosum ATCC8486 and control while using casein as substrate and higher (p < 0.05) than control with starch substrate. Moreover, the propionate and total VFAs production were increased (p < 0.05) in acetogens treated groups compared to control either using starch or casein as substrate. A previous study showed that E. limosum ATCC8486 was able to utilize amino acid and produce acetate and butyrate, which stimulated its growth [20]. So, isolated acetogens are able to compete for amino acids which are used as a substrate for methanogen growth and methanogenesis [21]. The highest A:P ratio was observed (p < 0.05) in the control with soluble starch and GA03 with casein. Several pieces of literature from in vitro incubations of rumen contents [7] indicated that acetogens can function as hydrogenotrophs to compete with methanogenesis. Thus, reductive acetogenesis which occurs in the rumen might be an effective way for methane mitigation [22]. Lopez et al. [23] reported that E. limosum strain ATCC8486 and Ser 5 reductive acetogens were decreased 5% CH4 production after a 24 h incubation period in vitro without affecting VFA production. Likewise, this study had similar total VFAs productions among E. limosum ATCC8486 and acetogens groups using starch as substrate, however, significantly (p < 0.05) higher in acetogens groups using casein as substrate.
In the present study, higher methane production was observed in casein than soluble starch. Higher methane production in case of casein substrate in this study may be attributed to effective degradability of dry matter (EDDM). Qin et al. [24] stated that wheat had relatively higher EDDM, which was fermented more rapidly by ruminal microbes. In case of soluble starch substrate, all 4 isolated acetogens had lower (p < 0.05) CH4 production in comparison to E. limosum and the control. Among them, only the GA03 had the lower CH4 production with both substrates. This Proteiniphilum acetatigenes GA03 strain was then selected as a suitable probiotic for in vivo experiment which may contribute to lowering enteric methane emission from cows that leads to less dietary energy loss and increase hydrogen energy and ultimately improve lactation performance.
An earlier report revealed that probiotics increase milk yield at 3%–16% in dairy cows supplemented with diet [6]. In contrast, a decreasing pattern of milk yield and a tendency to decrease of feed intake in all treatments were observed in this study. This is may be due to the increase in temperature recorded during the experimental period, from day 0°C at 31°C to day 60°C at 35.5°C. This is in agreement with Mitchell and Russo [25] who reported that high temperatures in summer reduced milk yield. However, the reduction of milk production was lower in the GA03 treated group than the control (2.4 kg vs 5.07 kg) which indicated that the GA03 probiotic potentially prevents the amount of milk reduction over time. The decreased feed intake reduced overall milk production and milk fat and caused low-milk-fat syndrome which probably involves both alterations in rumen fermentation and availability of endogenous fatty acid sources [26]. However, the GA03 probiotic potentially protects the amount of milk fat reduction by producing precursor of milk fat synthesis in lactating dairy cows. This result is also supported by the in vitro result where higher acetate was produced by the acetogens treated group than the control. In terms of milk protein, our result showed that control was decreased from 3.41% to 3.14% while the GA03 treatment was increased from 3.28% to 3.32%. Usually, milk quality was valued on the basis of its fat content but at present, milk payments are based on the content of fat as well as protein [27].
The use of milk somatic cell count (SCC) in payment systems as a public health criterion for international trade is increasing. Our results revealed that the SCC (×103/mL) was decreased in GA03 treatment from 128.43 to 107.00, whereas opposite in control treatment which was increased from 138.14 to 395.71 (Table 5). The SCCs are used as a guideline for the state of health and management of the breast [28]. Dohoo et al. [29] reported that cow affects SCC when stressed, and that cow with heat stress increases SCC [30]. It is expected that at high temperature and humidity, which is a summer feature of the south of Korea, GA03 has prevented bacterial infection due to high-temperature stress and humidity. Glucose in the blood was used as an important index to determine the nutritional status of individuals [31]. In this study, the average glucose level was 70.11 mg/dL in GA03 whereas 67.47 mg/dL in control. Availability of glucose is the prerequisite for high milk production in dairy cows. NEFA resulted in 240.52 uEq/L in control with 151.43 uEq/L in GA03. The supplements of GA03 may have an impact on lactating cows. The main goal of the dairy industry is to increase milk production. So, it is important to maintain animal health. Energy requirements for milk yield in early lactation of dairy cows go beyond the available energy from feed intake resulting in a more or less severe negative energy balance, which the lactation of dairy cows tries to compensate by fat-mobilization from adipose tissue. Excessive mobilization of fatty acids can exceed the liver’s capacity for degradation and results in the elevated formation of ketone bodies and accumulation NEFA in the liver where they are converted to triglycerides and stored [32]. In this experiment, BUN tended to be as low as 16.37 mg/dL in the treatment group compared to 18.09 mg/dL in the control group. BUN has been used as a useful indicator of protein metabolism. The higher BUN was observed on control trial compared with the GA03 group, which may be due to the protein degradation at higher rates or deamination because of higher protein intake and probably a more functional rumen [33]. Overall results indicated that GA03 supplementation can be a good probiotic in lactating dairy cows.
CONCLUSION
Addition of isolated acetogens increased acetate, propionate and total VFA productions. Proteiniphilum acetatigenes GA03 strain had the lowest CH4 production than the other isolated acetogenic bacteria. The in vitro results indicated that P. acetatigenes GA03 strain can be used as a direct fed microbials in order to hinder methanogenesis. This in vivo study indicates that the P. acetatigenes GA03 strain is able to prevent the reduction of milk yield and milk fat during summer seasons by maintaining rumen fermentation in lactating dairy cows. This would be possible to reduce gross energy loss by methane.