INTRODUCTION
Global warming is one of the most significant obstacles faced by the swine industry during hot and semi-hot seasons [1,2]. Heat stress compromises the growth performance and meat quality of growing pigs [3]. The first responses of animals to diminish metabolic heat increment are decreased average daily feed intake (ADFI) and metabolic interactions [1,2]. Low feed intake caused by heat stress in swine leads to imbalanced energy, body composition, and growth performance [4,5]. In heat-stressed pigs, not only feed intake but also intestinal integrity can be disrupted [3]. Additionally, intestinal fermentation order and short-chain fatty acid (SCFA) production can be affected [6]. In normal conditions, performance, welfare, and intestinal integrity were all increased in pigs fed 3% dietary fiber [7]. However, during heat stress, it has long been a practice to decrease dietary fiber and protein to lessen heat increment and mitigate the negative effects of high temperatures [4,8].
There is a lack of insight into how supplementing sources or levels of fiber during thermal stress affects the growth performance and health of growing pigs. Based on physical and chemical properties, dietary fiber is classified as soluble fiber (SF) or insoluble fiber (ISF) [1]. Pectin, gum, β-glucan, and hemicellulose are present in the SF, while cellulose, lignin, and insoluble arabinoxylan are found in the ISF [9]. The interaction between SF with water in digesta increases the volume of stools, resulting in more typical bowel motions, which may contribute to the mitigation of constipation incidence [7]. Furthermore, SF reduces the occurrence of diarrhea and improves water-holding capacity, gut health, and SCFA production, leading to a stable environment in the intestine [1]. Understanding how dietary SF can provide interactive advantages during heat stress is crucial due to the benefits of fiber in promoting intestinal growth in growing pigs. To analyze the effects on growth performance, nutrient digestibility, stress status, and metabolic changes in the gut, the diets in the current study were designed based on SF supplementation and different dietary energy levels in heat-stressed pigs.
MATERIAL AND METHODS
This experiment was carried out at the Republic of Korea’s National Institute of Animal Science Farm.
The Institutional Animal Care and Use Committee at the National Institute of Animal Science acknowledged the experiment’s adequate observance of ethical norms and institutional protocols (NIAS-2020458). All experiments were conducted in conformity with the necessary legislation and guidelines. Growing pigs (Landrace × Yorkshire × Duroc) were placed in partially slatted and concrete floor cages (2.8 × 2.0 m). A nipple drinker and a self-feeder were provided in each pen to allow ad libitum access to feed and water. A total of 96 growing pigs (initial body weight [BW], 56.6 ± 2.23 kg) were selected to be distributed randomly to one of six treatments. Each treatment had eight replicate pens with two pigs per pen. Two treatments in thermoneutral (TN) temperature (21°C–24°C) with dietary energy of 3,300 and the inclusion of high or low fiber, two treatments in heat stress (30°C–34°C) with dietary energy of 3,300 and the inclusion of high or low fiber, and two treatments in heat stress with dietary energy of 3,450 and the inclusion of high or low fiber in two growing periods (0–28 d and 29–56 d). Beet pulp was used as the source of fiber including 72.5% total fiber, 23.4% SF, and 49.1% ISF. According to the National Research Council [10], all of the diets met or surpassed the nutritional requirements for grower pigs (Table 1). The pens were equipped with temperature and humidity monitoring devices (Campbell Scientific, Shepshed, UK) that were positioned 1 m above the floor and utilized to continually record temperatures and relative humidity every 10 minutes. The recorded humidity and temperature were used for calculating the temperature and humidity index (THI) as described by Ataallahi et al. [11]. The THI was calculated using the following formula: THI = [(1.8 × temperature (°C) + 32) – (0.55 − 0.0055 × humidity) × (1.8 × temperature (°C) – 26)]. The recorded temperature and THI are shown in Fig. 1.
1) Supplied per kilogram of diet: 16,000 IU vitamin A, 3,000 IU vitamin D3, 5.0 mg vitamin B1, 20 mg vitamin B2, 4 mg vitamin B6, 0.08 mg vitamin B12, 40 IU vitamin E, 5.0 mg vitamin K3, 75 mg niacin, 40 mg pantothenic acid, 0.15 mg biotin, 0.65 mg folic acid.
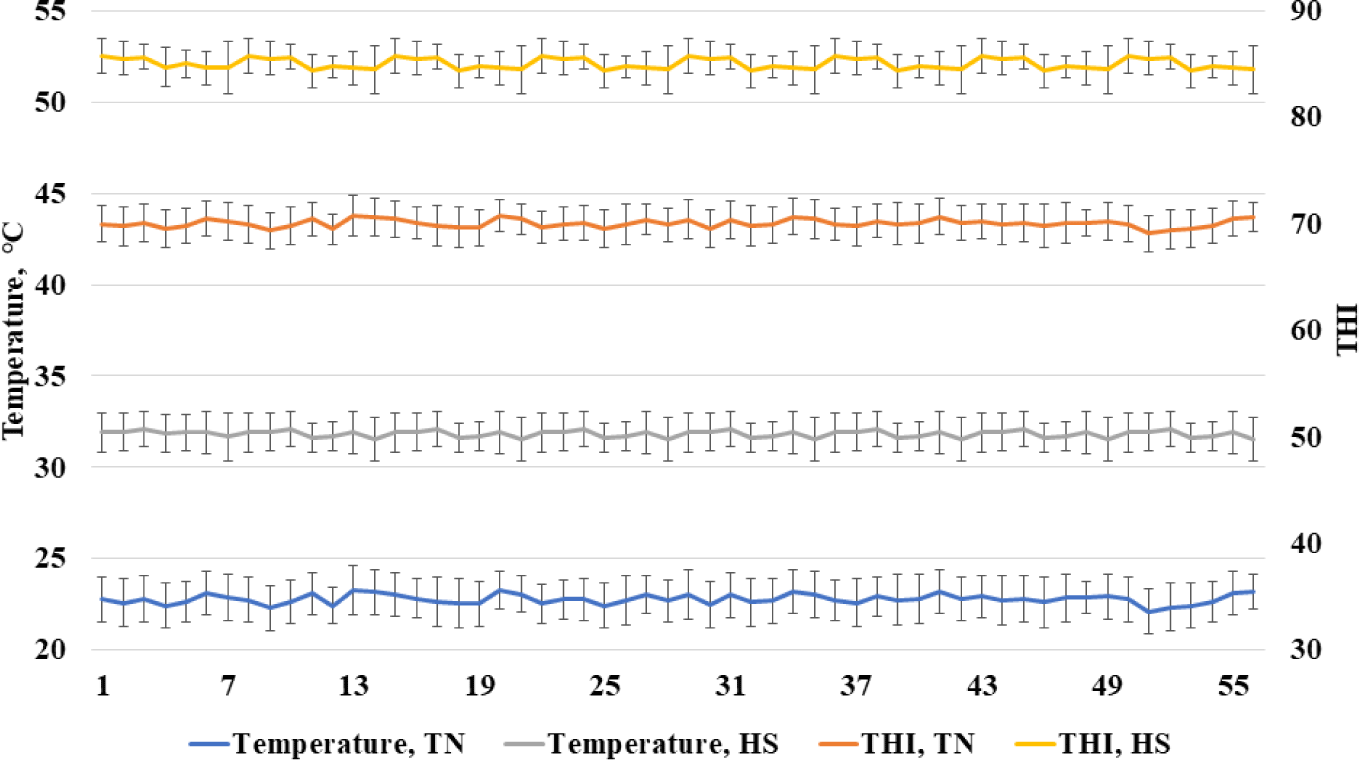
Throughout the trial, the rectal temperature was recorded every morning and evening at 8:00 to 9:00 and 19:00 to 20:00, respectively, using a digital thermometer that was inserted 2 cm into the rectum [2]. By counting the number of breaths per minute while watching the thoracic movement of the sow in a lying down position, the respiratory rate was simultaneously measured.
The digestibility of nutrients was evaluated as previously mentioned [12]. The nutrient digestibility evaluation was conducted to determine the apparent digestibility of dry matter (DM), gross energy (GE), and crude protein (CP) at the end of phases 1 and 2. On day 28 of phase 1 and day 56 of phase 2, the two chosen pigs were put in separate cages (one pig per cage) in order to collect fecal samples. Chromium oxide, an indigestible marker, was given to the feed of the chosen pigs at a rate of 2.5 g kg −1. For phase 1 and phase 2, feces samples were taken on days 26 through 28 and 54 through 56 respectively. Following sample collection, the drying (60°C, 72 h) step was carried out in a forced air-drying oven, and the material was thereafter ground with a mill (Thomas Model 4 Wiley Mill, Thomas Scientific, Swedesboro, NJ, USA) using a 1 mm screen. DM and CP diet and feces analyses were carried out according to the AOAC [13] technique. The concentration of Cr2O3 was measured using a calorimeter bomb (Model 1261, Parr Instrument, Moline, IL, USA) and a spectrophotometer (Jasco V-650, Jasco, Tokyo, Japan). Every day at 7:00 a.m. and 15:30 a.m., feces were collected, combined, sealed in plastic bags to be frozen at −20°C. The fecal samples from each pig were collected, frozen, and pooled, dried for 72 hours in a forced-draft oven (65°C), crushed through a 1-mm screen, and well mixed, and then a subsample was obtained for chemical analysis.
Blood samples (10 mL) were collected by puncture of the ear veins and transferred in vacuum tubes (5 mL) and heparinized tubes (5 mL) for zonulin, lipopolysaccharide (LPS), and lipopolysaccharide-binding protein (LBP) analysis. In order to obtain serum, the samples were centrifuged at 12,000×g for 15 minutes at 4°C in vacuum tubes. The serum samples were then kept at −80°C for further analysis. Using an enzyme-linked immunosorbent assay technique, pigs serum and feces samples were examined for LPS, zonulin (Cat # MBS2607498, MyBioSource, San Diego, CA, USA), and binding protein LBP (Nanjing Jiancheng Bioengineering Institute, Nanjing, China). Fecal samples were used for lipocalin-2 determination.
The concentrations of cortisol in the hair was determined as previously mentioned [14]. At days 90 and 112 of gestation, hair samples from the sows’ foreheads were taken. Aluminum foil was used to collect and store the hair samples before they were dried in polypropylene tubes (HM Hyundai Micro, Seoul, Korea). Samples were cleaned of contaminants by being washed three times in 5 mL of isopropyl alcohol and then allowed to dry for seven days at room temperature (24°C). According to the manufacturer’s instructions, cortisol was extracted using a methanol dilution technique after drying (Cayman Chemical, Ann Arbor, MI, USA).
As stated by Jeong et al. [15], gas chromatography (GC) was used to measure the SCFA levels in fecal samples. The samples were divided using a TRACE 1310 GC equipped with a flame ionization detector, and they were examined on an HP-88 column (100-m length, 0.25-mm diameter, and 0.2-m film thickness from the producer). The temperature schedule was as follows: 70°C for 1 minute, 180°C maintained at 25°C for 1 minute, 200°C maintained at 10°C for 1 minute, 220°C maintained at 2°C for 10 minutes, and finally 240°C maintained at 20°C for 6 minutes. The sample was run at a split ratio of 20:1 with a column flow rate of 1.3 mL/min. The use of hydrogen as a carrier gas is made. The use of hydrogen as a carrier gas was considered and the injector and detector were both set at 270°C and 290°C, respectively.
The MIXED procedure was applied to perform the statistical analysis (SAS Institute Cary, NC, USA), based on two 2 × 2 factorial arrangement of treatments as room temperature×dietary fiber level and dietary energy level vs dietary fiber level in randomised blocks. Initial BW was included as a covariate factor. Using Turkey’s Honestly Significant Difference technique, statistical differences between the treatments were defined as those with significant differences (p < 0.05). For all parameters, a single pig was regarded as the experimental unit.
RESULTS
Among standard energy level treatments (Table 2), heat-stressed pigs showed lower final BW, average daily gain (ADG), and ADFI in phase 1, phase 2, and overall. Moreover, the growth-to-feed ratio (G:F) was decreased in heat-stressed pigs in phase 1 and phase 2, however, the overall G:F was unaffected. Dietary fiber level showed no effects on the final BW, ADG, ADFI, and G:F during the experiment. There was a positive interaction for ADG between room temperature and dietary fiber.
Among heat stress treatments, dietary energy level did not affect final BW, ADG, ADFI, and G:F during the whole experiment. Pigs fed high dietary fiber showed no change in final BW, ADG, ADFI, and G:F in phase 1 and phase 2, however, the overall ADG was increased in pigs fed a high fiber. There were no interactive effects between room temperature and dietary fiber.
Among standard energy level treatments (Table 3), heat-stressed pigs showed lower digestibility of DM, GE, CP, crude fiber (CF), neutral detergent fiber (NDF), and acid detergent fiber (ADF) in phase 1 and phase 2. Dietary fiber level showed no effects on the digestibility of DM, GE, CP, CF, NDF, and ADG in phase 1 and phase 2. There was no interaction for nutrient digestibility parameters between room temperature and dietary fiber.
Among heat stress treatments, dietary energy level did not affect the digestibility of DM, GE, CP, CF, NDF, and ADF during the whole experiment. Pigs fed high dietary fiber showed no change in digestibility of DM, GE, CP, CF, NDF, and ADF in phase 1 and phase 2. There were no interactive effects on nutrient digestibility between room temperature and dietary fiber.
Among standard energy level treatments (Table 4), heat-stressed pigs showed lower concentrations of acetate, butyrate, propionate, valerate, and total SCFA in feces in phase 1. Moreover, the concentration of acetate, propionate, butyrate, valerate, isovalerate, and total SCFA in feces was reduced in heat stressed-pigs in phase 2. Heat-stressed pigs showed a lower concentration of isobutyrate in feces in phase 2. The concentration of acetate, propionate, butyrate, and total SCFA in feces was increased in pigs fed high dietary fiber in phases 1 and 2. There was a negative interaction between dietary fiber and energy for the fecal concentration of isobutyrate in phase 1 and valerate in phase 2.
Among heat stress treatments, dietary energy level did not affect the concentration of acetate, propionate, butyrate, valerate, isovalerate, and total SCFA in feces during phases 1 and 2. Pigs fed high dietary fiber showed a greater concentration of acetate, propionate, butyrate, and total SCFA in phase 1. There were no interactive effects on nutrient digestibility between room temperature and dietary fiber. Moreover, the concentration of acetate, propionate, butyrate, valerate, isovalerate, and total SCFA were increased in feces of pigs fed high dietary fiber in phase 2. However, a lower concentration of isobutyrate was shown in pigs fed high dietary fiber in phase 2.
Among standard energy level treatments, pigs in heat stress treatments showed a higher (p < 0.05) rectal temperature on wk 1 to 8 compared with pigs in TN groups (Fig. 2). Among heat stress treatments, high fiber treatments showed a lower respiratory rate at wk 7 and 8. Among standard energy level treatments, pigs in heat stress treatments showed a higher (p < 0.05) respiratory rate on wk 1 to 8 compared with pigs in the TN groups (Fig. 3). Among heat stress treatments, there was no change in respiratory rate between the pigs fed diets with different energy levels. Moreover, high-fiber treatments showed a lower rectal temperature rate at wk 7 and 8. There was no change in rectal temperature between the pigs fed diets with different energy levels. Among standard energy level treatments, pigs in heat stress treatments showed a higher (p < 0.05) hair cortisol in phases 1 and 2 compared with pigs in the TN groups (Table 5), however, dietary fiber did not show any changes in hair cortisol. Among heat stress treatments, dietary fiber levels and energy levels showed no change in hair cortisol.
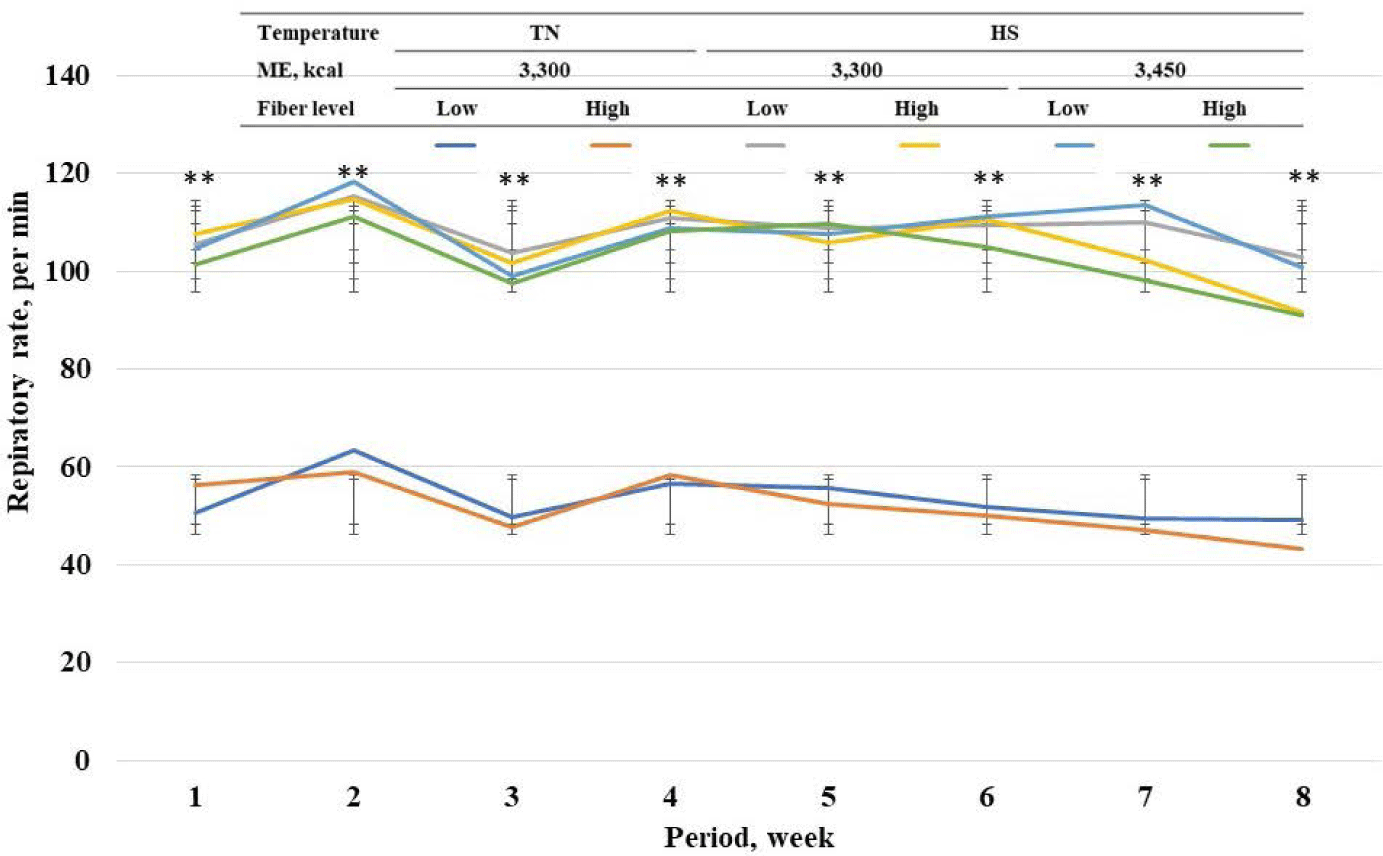
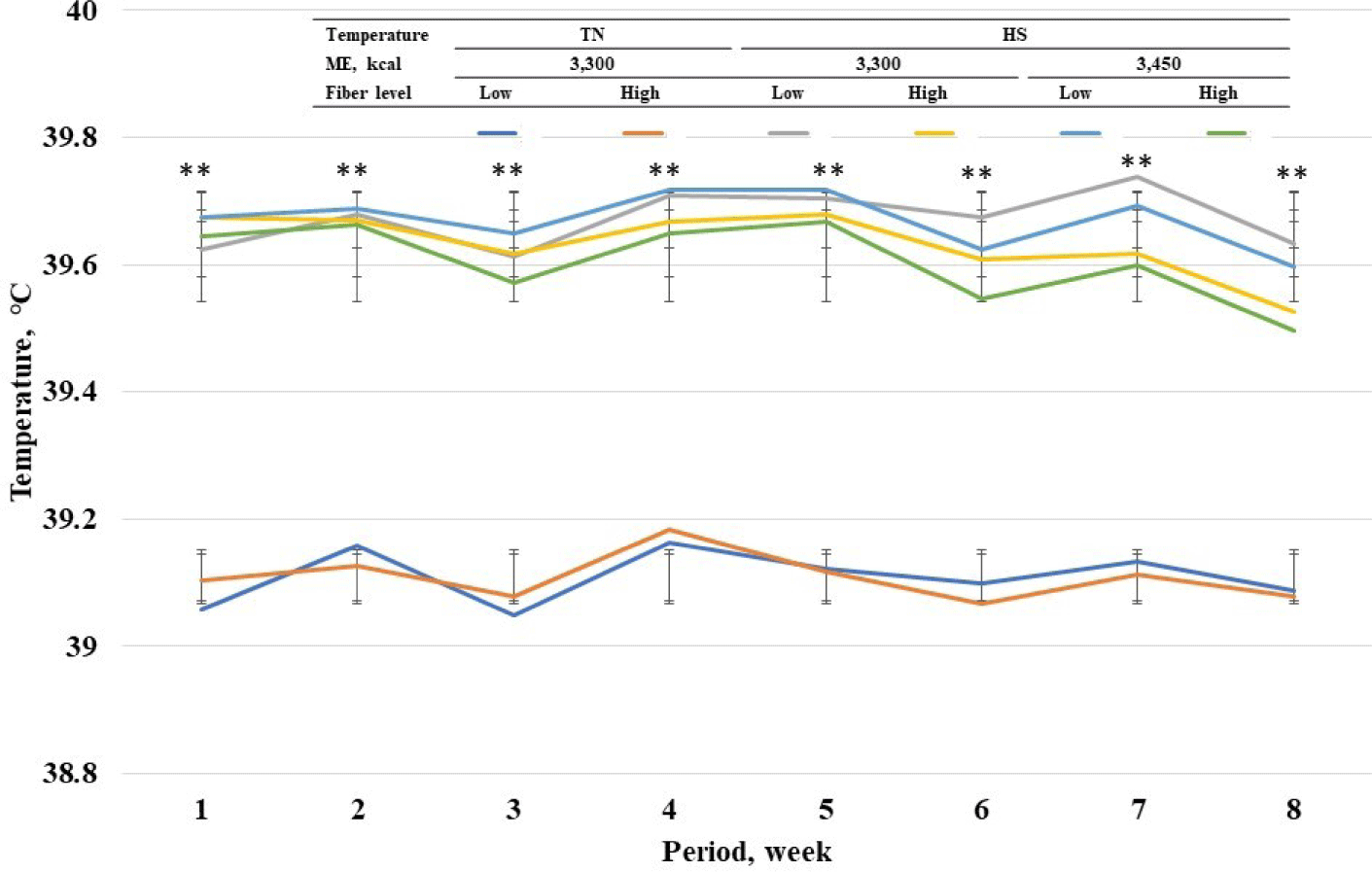
Among standard energy level treatments, pigs in heat stress treatments showed a higher (p < 0.05) plasma zonulin in phase 1, and a higher plasma zonulin and fecal lipocalin-2 in phase 2 compared with pigs in TN groups (Table 6). Moreover, dietary high fiber reduced plasma zonulin in phase 1. Among heat stress treatments, dietary energy level did not affect plasma zonulin and fecal lipocalin-2, however, high fiber treatments decreased plasma zonulin in phases 1 and 2. Among standard energy level treatments, pigs in heat stress treatments showed a higher (p < 0.05) plasma LPS and LBP in phases 1 and 2 compared with pigs in TN groups (Table 7). Moreover, dietary high fiber reduced plasma LBP in phases 1 and 2. Among heat stress treatments, dietary energy level did not affect plasma LPS and LBP, however, high fiber treatments decreased plasma LPS and LBP in phases 1.
DISCUSSION
Chronic heat stress has been linked to decreased growth performance in growing pigs [3] via compromising gut health and feed consumption. According to the current study, prolonged heat stress markedly reduced ADFI, ADG, and G:F. Although the fiber level groups consumed the same amount of feed, the ADG was higher in pigs fed high-fiber diet than those of the low-fiber group, suggesting that the high temperature and reduced feed intake together are the reasons for the decline in growth performance. Decreased feed consumption has negative effects on pigs growth performance and meat quality. Addressing low feed intake and poor nutrient absorption in heat-stressed pigs, the aim of the current feeding strategy was to offer a high-energy concentrated meal dense in nutrients while limiting the quantity of undigestible fibre. Along with financial losses imposed by performance declines, the sow’s health is also harmed. Increased medicine expenditures to treat the rise in inflammation and an increase in mortality further reduce the performance of farms. The pigs benefit greatly from the alternative strategy of providing significant amounts of fiber since the conventional method only focuses on nutrient absorption impairment, but the fibre strategy optimizes intestinal integrity as well. In contrast, it has been reported that the decrease of SF that leads to excessive fermentation is taken into account when formulating recommendations for fiber supplementation under heat-stress circumstances [7]. High levels of fiber-bound protein are also present in wheat bran and sugar beet pulp, which favors unfavorable protein fermentation in the large intestine[6,16,17]. However, the result of our study showed the positive role of fermentation on intestinal health, digestibility of nutrients, and growth performance.
As a result, poor nutrient absorption can lower available nutrients to maintain body metabolism, severely impacting growth performance. Energy levels and fiber inclusion did not affect the digestibility of DM, CP, GE, CF, NDF, and ADF, however, the HS decreased the digestibility of DM, CP, GE, CF, NDF, and ADF compared with non-stressed treatments. Hemicellulose and pectin form the soluble portion of fibers, which can be fermented by gut bacteria [1,7]. As a result, the SF enhances gut health, which facilitates nutrition absorption. The ISF component of fiber, on the other hand, is not affected by fermentation and is excreted undigested, as evidenced by the fact that the digestibility of ADF was not enhanced in heat-stressed pigs in the current experiment.
The fiber is used as substrates for SCFA production at the distal section of the colon other than dietary starch or protein [2,18]. The major byproducts of fermentation in the large intestine include acetate, propionate, and butyrate [7,15]. The quantity of SCFA synthesis depends on a number of variables, including the environment, nutrition, and intestinal microbiota [3]. Dietary fiber in soluble form is reportedly a more significant substrate for SCFA synthesis [1]. Although SCFA can be synthesized in all parts of the gastrointestinal tract, it is mostly produced in the colon and cecum [18]. Dietary fiber may enclose carbohydrates, facilitate their transit to the large intestine, and finally enhance fermentation [1]. The immune system, lipid metabolism, digestive organs health, and carbohydrate balance are predicted to be SCFA’s primary targets [7]. SCFA prevent histone deacetylation and have a beneficial function in body metabolism by binding to particular receptors in the gut, such as G-protein-coupled receptors 41 and 43 [2]. In our study productions of acetate, propionate, and butyrate were increased in heat-stressed pigs fed SF, however, the acetate production was relatively more regulated. In agreement, SF produces more acetate than butyrate [1]. Effect of fermentation and acetate synthesis in the colon plays a significant role in promoting intestinal development and health by lowering the vulnerability to LPS [1]. Additionally, SCFA production enhances barrier function and decreases intestinal permeability in epithelial cells, which is a crucial factor in modifying intestinal health, inflammation, and nutritional digestibility [3,9]. SF fermentation can help to improve gut health by reducing constipation due to excellent water-holding capacity [19]. In addition, starch-based carbohydrates can escape enzymatic digestion by being encapsulated in fiber structures, which indeed supply additional substrates for fermentation in distal regions of the intestine [12]. Our results confirm the necessity of dietary SF as fermentation substrates for SCFA production.
During a stressful condition, the release of cortisol is a common physiological response [20]. According to the findings of our study, an increase in hair cortisol is related to an elevation of room temperature. Fast-growing animals have a substantial reaction to cortisol release under heat stress, due to considerable tissue formation, weight gain, and immunological change [21,22]. Because of the long-term accumulation of cortisol in the hair, earlier studies demonstrated that hair cortisol is a better biomarker of chronic heat stress than blood cortisol [23]. The relationship between chronic stress and hair cortisol has been demonstrated in cattle [24] and sheep [25,26]. The mechanism of action behind the role of SF level on cortisol secretion in growing pigs has not been validated, however, satiety may be associated with decreased stress.
Heat stress impacts the physiological status of animals on several levels: in vitro data for cell cultures showed a heat stress-related lowering of the trans-epithelial electric resistance, which evaluates the integrity of the intestinal barrier in growing pigs. High-fiber treatments significantly reduced zonulin secretion. An increased zonulin concentration indicates a leaky gut, and any decrease in zonulin release is related to intestinal health at the mucosa level [1]. The intestinal tight junction injury was observed following the exposure of pigs to heat stress, which was demonstrated by the rise in zonulin and LPS in the serum. This finding is noteworthy since various authors have shown that pathogenic bacterial translocation associated with the onset of systemic inflammation may be mediated by a loss of intestinal epithelial barrier integrity [9,27,28]. The observed villus integrity may be explained in part by changes in the fermentation order, butyric acid synthesis, LPS penetration, plasma LBP, and zonulin concentration. The poorer the intestinal barrier, the quicker microorganisms can enter the body, causing infections and inflammatory processes as an immunological response. The more energy a pig must spend on immunological reactions, the less energy is available for growth.
CONCLUSION
In conclusion, based on the growth performance results, our study showed that the supplementation of SF in the pig diet is necessary during heat stress. The improvement in the production of SCFA and the reduction of zonulin, LPS, and LBP release into the blood emphasize antimicrobial activity and intestinal health. These beneficial alterations in gut health appeared in lower stress and cortisol deposition in hair. This finding may offer further insight into the importance of reducing the adverse effects of heat stress during growing stages and the function of SF as a regulator of intestinal integrity.