INTRODUCTION
For several decades, antibiotics (AB) have been used in pig diets to promote growth, improve feed efficiency, and prevent diseases [1]. However, the use of antibiotics is a concern because of the potential occurrence of antibiotic-resistant bacteria and residual antibiotics in pork [2,3]. Hence, the swine feed industry in many countries has prohibited the use of AB in swine diets, and identifying alternatives has become a research focus [4-7]. Bacteriophages (BP) have received attention as appropriate AB alternatives and many studies on BP have been conducted in pigs [8-10].
As the BP are non-hazardous and self-replicating agents that are parasitic on bacteria as host, they have been suggested to infect and grow on bacteria [11]. Therefore, supplemental BP in pig diets may mimic the antimicrobial effects of AB, promoting the digestion and absorption of nutrients by eliminating detrimental bacteria in the intestinal tract. Previous studies reported that supplemental BP in pig diets increases the apparent total tract digestibility (ATTD) of energy and nutrients [12] and decreases the concentration of Escherichia coli and Salmonella in feces [13]. In addition, a recent study reported that supplemental BP improved intestinal barrier function and intestinal microbiota in nursery pigs [10]. However, information on the influence of BP on changes of microbial concentrations in the small intestine and ileal nutrient digestibility is lacking. Thus, to bridge this gap, the present work aimed to determine the effects of BP on the relative proportions of microbiota in the ileal digesta and feces and on nutrient digestibility in pigs.
MATERIALS AND METHODS
The experimental protocols were reviewed and approved by the Institutional Animal Care and Use Committee of Konkuk University (KU15016).
Twelve crossbred castrated male pigs with an initial mean body weight of 29.5 kg (standard deviation = 2.3) were employed to assess the effect of BP on microbial concentrations in the small and large intestines, as well as energy and nutrient digestibility. Pigs were fitted with a T-cannula at the distal ileum using the surgical procedure described by Stein et al. [14]. The animals were individually housed in pens (2.0 × 2.2 m) equipped with a feeder and a nipper waterer. The 12 animals were randomly allotted to one of 3 dietary treatment groups in a quadruplicated 3 × 2 Latin square design, with 3 experimental diets and 2 periods per square. Potential residual effects were balanced using a spreadsheet program developed by Kim and Kim [15].
The 3 experimental diets were (Table 1): a control diet mainly based on corn and soybean meal, with 0.2% ground corn cob and wheat shorts as BP carriers; an AB diet, supplemented with 0.1% AB (avilamycin; Avilamix, CTCBIO, Seoul, Korea); and a BP diet supplemented with 0.2% BP at the expense of the BP carriers in the control diet. The energy and nutrient concentrations in all diets met or exceeded the requirement estimates suggested by the NRC [16]. All experimental diets contained chromic oxide at 0.5% as an indigestible index. The BP product was obtained from CTCBIO Inc. (Seoul, Korea). The product contained a mixture of BP consisted of Clostridium perfringens types A and C, Escherichia coli (f41, k88, and k99), Salmonella spp. (Salmonella choleraesuis, Salmonella derby, Salmonella enteritidis, and Salmonella typhimurium), and Staphylococcus aureus. The BP product contained 109 plaque-forming units per gram.
2) BacterPhage (CTCBIO) contained contained a mixture of BP consisted of Clostridium perfringens types A and C, Escherichia coli (f41, k88, and k99), Salmonella spp. (Salmonella choleraesuis, Salmonella derby, Salmonella enteritidis, and Salmonella typhimurium), and Staphylococcus aureus. The BP product contained 109 plaque-forming units per gram.
4) Provided the following quantities per kg of complete diet: vitamin A, 25,000 IU; vitamin D3, 4,000 IU; vitamin E, 50 IU; vitamin K, 5.0 mg; thiamin, 4.9 mg; riboflavin, 10.0 mg; pyridoxine, 4.9 mg; vitamin B12, 0.06 mg; pantothenic acid, 37.5 mg; folic acid, 1.10 mg; niacin, 62 mg; biotin, 0.06 mg; Cu, 25 mg as copper sulfate; Fe, 268 mg as iron sulfate; I, 5.0 mg as potassium iodate; Mn, 125 mg as manganese sulfate; Se, 0.38 mg as sodium selenite; Zn, 313 mg as zinc oxide; butylatedhydroxytoluene, 50 mg.
The animals were fed the experimental diets at 3 times the daily maintenance requirement for energy (i.e., 197 kcal metabolizable energy/kg body weight (BW)0.60; [16]). The amount of feed allowance per pig was divided into 2 equal meals and provided to pigs at 0800 and 1700 h. Water was freely available. An experimental period was consisted of 4 days of adaptation to the experimental diet, 1 day of fecal collection, and 2 days of ileal digesta collection. Fecal samples were collected from 0900 to 1800 on day 5 by grab sampling, and rectal massage was carried out to acquire fresh fecal samples for microbial community analysis. Ileal digesta samples were collected from 0900 to 1630 on days 6 and 7 using a wired plastic bag fixed to a T-cannula. The plastic bags were replaced whenever filled with ileal digesta, or at least every 30 min. Collected feces and ileal digesta were immediately stored at −20°C for further analyses. After the termination of the first period, 4 days of buffering period was used to prevent potential carryover effects.
Bacterial genomic DNA were extracted from fresh ileal and fecal samples and the V4 region of the 16S rRNA gene was amplified. Amplicons were sequenced on an Illumina MiSeq, and microbial communities and alpha diversity were analyzed using Quantitative Insights into Microbial Ecology as described by Han et al. [17].
Fecal samples were dried in a forced-air drying oven and ileal digesta samples were lyophilized in a freeze dryer and finely ground (< 1 mm) before chemical analysis. Diets, feces, and ileal digesta samples were analyzed for dry matter (AOAC [18]; method 930.15), crude protein (AOAC [18]; method 990.03), and chromium (AOAC [18]; method 990.08) contents. The fibers were analyzed (D200; Ankom Technology, Macedon, NY, USA) for neutral detergent fiber and acid detergent fiber contents. All samples and diets were analyzed for gross energy using bomb calorimetry (Model C2000, IKA, Staufen, Germany).
The apparent ileal digestibility (AID) and ATTD of energy and nutrients were calculated using the index method [19]. Experimental data were analyzed using the MIXED procedure of SAS 9.4 (SAS Institute, Cary, NC, USA). The statistical model included dietary treatment as the fixed variable and replication, period within replication, and animal within replication as random variables. The proportion of microbial communities in each treatment was expressed as a percentage of the total 16S rRNA gene sequences. Least squares means for the response variables were calculated for each treatment. Orthogonal contrasts were used to compare the control versus the AB and BP groups and the AB group versus the BP group. An individual pig was the experimental unit. Statistical significance and trend were determined at p ≤ 0.05 and 0.05 < p ≤ 0.10, respectively.
RESULTS
One pig that was provided with the control diet declined to consume the diet during the collection period and was subsequently excluded from the data for statistical analysis.
A total of 528,719 (mean = 11,494 ± 575) 16S rRNA reads were generated, with an average of 12,361 (±1,465) and 9,970 (±1,627) reads in ileal digesta and feces from the pigs fed the control diet, 12,787 (±699) and 10,633 (±1,627) reads in ileal digesta and feces from the pigs fed the AB diet and 12,932 (±1,761) and 10,198 (±1,059) reads in ileal digesta and feces from the pigs fed the BP diet, respectively.
The alpha diversity of the microbial communities in the ileum and feces was not affected by AB or BP supplementation (Figs. 1 and 2). At the phylum level, Firmicutes accounted for the largest proportion of the microbiota in the ileal and fecal samples of all groups, followed by Bacteroidetes (Table 2). The proportion of Bacteroidetes in the ileal digesta tended to be less (p = 0.099) in the pigs fed the AB- or BP-supplemented diets compared with the control group, but there was no difference between AB and BP groups. The proportion of Firmicutes in the ileal digesta tended to be greater (p = 0.059) in the pigs fed the AB or BP diets than in the control group but there was no difference between the AB and BP groups. In the feces, there were no differences in the relative proportions of Bacteroidetes and Firmicutes among all treatments.
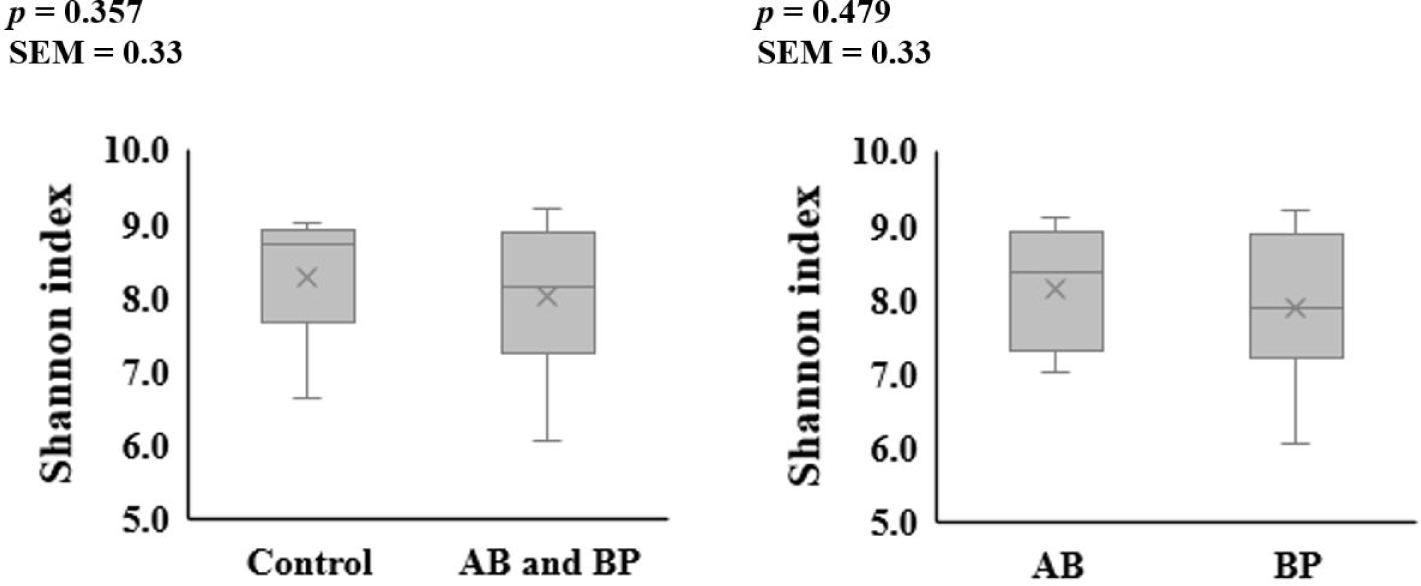
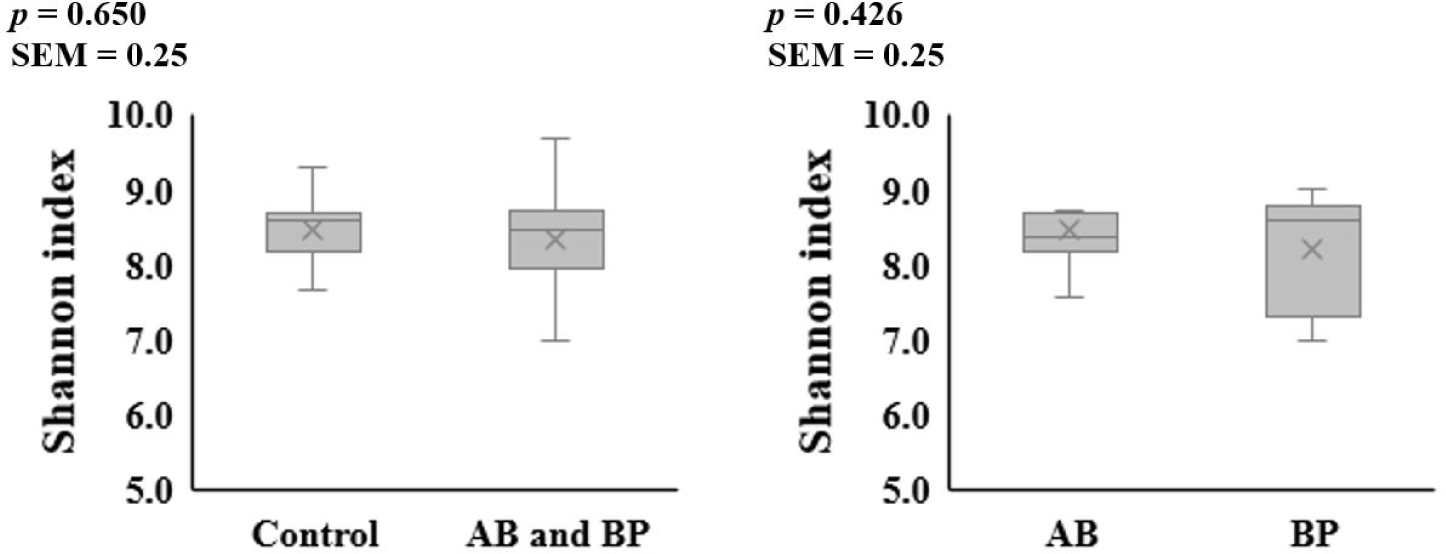
8) BacterPhage (CTCBIO) was supplemented at 0.2%. The product contained a mixture of BP consisted of Clostridium perfringens types A and C, Escherichia coli (f41, k88, and k99), Salmonella spp. (Salmonella choleraesuis, Salmonella derby, Salmonella enteritidis, and Salmonella typhimurium), and Staphylococcus aureus. The BP product contained 109 plaque-forming units per gram.
At the genus level, the proportion of Lactobacillus in the ileal digesta was the most dominant and tended to be greater (p = 0.062) in the pigs fed the AB- or BP-supplemented diets than in the control group, with no difference between the AB and BP groups (Table 3). The proportions of Bacteroides (p = 0.074) and Streptococcus (p = 0.088) in the ileal digesta tended to be less in the AB and BP groups compared to the control group with no difference between the AB and BP groups. In the fecal samples, the relative proportion of Lactobacillus was the most dominant. The relative proportion of Bifidobacterium was less (p = 0.029) in the AB and BP groups compared with the control group with no difference between the AB and BP groups. In contrast, the relative proportions of Parabacteroides and Succinivibrio were less (p < 0.05) in the AB group compared with the BP group.
4) BacterPhage (CTCBIO) was supplemented at 0.2%. The product contained a mixture of BP consisted of Clostridium perfringens types A and C, Escherichia coli (f41, k88, and k99), Salmonella spp. (Salmonella choleraesuis, Salmonella derby, Salmonella enteritidis, and Salmonella typhimurium), and Staphylococcus aureus. The BP product contained 109 plaque-forming units per gram.
The AID and ATTD of energy and nutrients were not affected by the supplemental AB or BP (Table 4).
3) BacterPhage (CTCBIO) was supplemented at 0.2%. The product contained a mixture of BP consisted of Clostridium perfringens types A and C, Escherichia coli (f41, k88, and k99), Salmonella spp. (Salmonella choleraesuis, Salmonella derby, Salmonella enteritidis, and Salmonella typhimurium), and Staphylococcus aureus. The BP product contained 109 plaque-forming units per gram.
DISCUSSION
The BP bind to specific receptors on the surface of bacteria prior to introducing their genetic materials [20,21]. The relationship between BP and bacteria is either lytic or lysogenic. During lytic infection, the phages adhere to the surface of bacteria and they inject their chromosomes into the bacterial cells. After that, the phages reproduce and release virulent phages. In the lysogenic cycle, the genetic materials of phages incorporate into bacterial chromosomes. This incorporation permits bacteria to reproduce generally along with phage genetic material known as prophages. Consequently, temperate phages are released, and these have the potential to convert into virulent phages at any time [22]. Thus, the antimicrobial potential of virulent phages is greater than that of temperate phages. Each type of BP can efficiently infect specific bacteria more efficiently than others [23]. As the BP product used in this study contained a cocktail of BP for Clostridium perfringens types A and C, Escherichia coliSalmonella spp., and Staphylococcus aureus, the phage product may be specifically effective against these bacteria. Kim et al. [24] reported that supplemental BP at 0.1% or 0.15% decreased ileal Escherichia coli and Clostridium proportion in pigs. Furthermore, a previous study found that supplemental BP against Salmonellatyphimurium increased the body weight gain and feed efficiency in Salmonella-challenged growing pigs [25].
Avilamix containing 20 g/kg avilamycin was used in the present study. Avilamycin is an AB that inhibits protein synthesis by selectively targeting bacterial ribosomes. This action disrupts protein production, effectively suppressing bacterial growth and demonstrating antibacterial activity [26]. A previous study observed a significant reduction in Salmonella count in chicks treated with 100 ppm avilamycin compared to the control group [27].
The overall microbial diversity comprised prevalent intestinal microbial groups, with Firmicutes (35%), Bacteroidetes (21%), Proteobacteria (3%), and Spirochaetes (2%) dominating the total 16S rRNA gene sequences, as previously observed in pigs [28,29]. However, according to a meta-analysis to define the core microbiota in the gastrointestinal tract of pigs, the ileal digesta primarily consisted of Firmicutes and Proteobacteria, whereas the phylum composition in the cecum and colon exhibited a high level of consistency [30]. In the ileal digesta in the present study, Bacteroidetes were 10 percentage unit greater than that reported in the literature. This inconsistency might be explained by different ileal digesta sampling methods. Most studies in the meta-analysis obtained ileal samples by necropsy at the end of the experiment [31-33]. In contrast, the collection of ileal digesta in this study used a T-cannula inserted into the terminal ileum, approximately 15 cm from the ileocecal valve [14]. Because the flow of ileal digesta in the small intestine is not consistent in one direction, the ileal digesta collected using a T-cannula may contain some cecal digesta. The analytical procedure, such as the targeted region of the 16S rRNA gene and DNA purification methods, also affects the composition of the microbial community [34].
Intestinal microbiota plays a critical role in maintaining immune function, nutritional status, and physiology in pigs. [35,36]. Accordingly, major or frequent changes in intestinal microbiota are often associated with ill health [37-39]. Firmicutes and Bacteroidetes are the 2 dominant phyla in the intestine of pigs [40]. The proportions of Firmicutes and Bacteroidetes in the intestine are associated with the maintenance of homeostasis and changes in their proportions can lead to various pathologies. It has been reported that a decrease in the proportion of Bacteroidetes together with an increase in the proportion of Firmicutes can create an intestinal environment conducive to energy production and absorption in the intestine [41,42]. To our knowledge, we first report the effects of BP on microbiota at the phylum level in the ileal digesta of pigs. The effects of BP on the alteration of microbiota at the phylum level in the ileal digesta were similar to those of AB. However, the effects of AB or BP on fecal microbiota at the phylum level have shown inconsistent results compared to the ileal digesta, which may be explained by the experimental period. Fecal samples were collected after 4 days of the adaptation period, which could be insufficient to detect noticeable changes. However, ileal digesta samples were collected after 5 days of adaptation and the fecal collection period, which might be sufficient to detect the effects of supplemental AB or BP.
Bifidobacterium and Lactobacillus exert beneficial effects on the intestinal environment of animals [43-45]. The growth of these beneficial bacteria was enhanced by the addition of AB. Because the antimicrobial action of AB reduces the colonization of harmful bacteria, it is likely to result in an increase in probiotics in a less competitive intestinal environment [46]. The avilamycin used in this study is recognized for its primary targeting of Staphylococcus aureus, Streptococcus pneumoniae, and Streptococcus pyogenes [26]. However, our study did not detect these specific harmful bacteria and previous research indicated no significant impact on the normal gut flora [47]. This aligns with our findings, suggesting that microbial diversity remains unaffected by avilamycin administration. In this study, we hypothesized that microbes affected by avilamycin could be found among the low-abundance microbes.
The increased proportion of Lactobacillus in the ileum with supplemental AB or BP was likely due to the inhibitory action of AB or BP on pathogenic bacteria. To our knowledge, only one study determined the effect of BP on the microbiota of this genus in the ileal digesta of pigs. Kim et al. [24] reported that supplemental BP at 0.10% or 0.15% increased the ileal Lactobacillus proportion but had no effect on the fecal Lactobacillus proportion in pigs, which agrees with the present results. However, previous studies have reported that supplemental BP increased the fecal Lactobacillus proportion in pigs [8,12,13]. The reason for the inconsistency between the present and previous studies is unclear.
The less proportion of Bifidobacterium in the feces observed in the AB or BP group compared with the control group was inconsistent with previous studies [8,12,24], the reason for which remains unclear. However, another study reported that a supplemental AB mixture consisting of ampicillin, gentamycin, and metronidazole resulted in decreased Bifidobacterium abundance in the feces of pigs [48], which agrees with the present study. They observed that the fecal Bifidobacterium proportion was positively correlated with branched-chain fatty acid concentration in the feces of pigs fed AB. Branched-chain fatty acids are formed from branched-chain amino acids that originate exclusively from the breakdown of proteins and thus serve as indicators of microbial branched-chain amino acid deamination. Thus, they suggested that the reduction in branched-chain fatty acids due to the effect of AB on microbial nitrogen results in a decreased Bifidobacterium proportion in the feces [48].
At the genus level in the gut, the target bacteria of BP containing Clostridium perfringens, Escherichia coli, Salmonella spp., and Staphylococcus aureus were not detected in this study. However, the proportion of Streptococcus in the ileal digesta decreased with the addition of AB or BP. Streptococcus is a genus of bacteria and certain species within this genus are known to cause various diseases. However, the results of this observation are unclear because of the known host specificity of BP.
The ATTD of energy and nutrients in the control diet was within the range of previous data [49,50]. The index method used to calculate digestibility in this study relies greatly on the accurate chemical analysis of index compounds in the feed and digesta, which requires sufficient adaptation to achieve a constant fecal index concentration. Choi and Kim. [51] suggested that a minimum adaptation time of 3.5 days is needed to achieve a constant fecal index concentration if a high-fiber diet containing 30% neutral detergent fiber is fed, whereas 5.5 days are needed for a low-fiber diet containing 5% neutral detergent fiber. Because the neutral detergent fiber concentration of the experimental diets in this study was greater than 5%, an adaptation period of 4 days was sufficient.
The intestinal microbiota helps the host utilize nutrients and defend against pathogens [52]. However, in the present study, BP supplementation did not affect nutrient digestibility despite having positive effects on the intestinal microbial community. There are two potential reasons for these results. First, the species of microbes are critical for significant changes in nutrient digestibility. Niu et al. [53] suggested that only specific microbial species improve nutrient digestibility. In their study, the proportions of 3 phyla of Proteobacteria, Tenericutes, and TM7, and 11 genera including Anaeroplasma, Campylobacter, and Clostridium were positively correlated with apparent crude fiber digestibility. However, in the present study, there were no differences in the abundance of these phyla or genera among the treatments. Second, the experimental conditions, such as the use of a variety of AB, experimental diet composition, and experimental period, differed among the studies. There are discrepancies among studies on the relationships between microbial communities and nutrient digestibility in pigs. A strong relationship between the intestinal microbiota and nutrient digestibility in pigs has been reported in multiple studies [12,13,48] whereas no relationship was observed in other studies [54,55]. These discrepancies were attributed to the fact that the magnitude of microbial changes was not large and perhaps would not improve nutrient digestibility because of the short experimental period compared to previous studies. Previous studies fed diets containing 0.05% [13] or 0.1% [12] BP for more than 30 days, whereas this experiment was conducted for only 7 days. In addition, Kim et al. [24] reported that supplementation with BP at 0.15% resulted in increased apparent total tract dry matter digestibility for 35 days, but had no effect when fed for 7 days.
In conclusion, although BP supplementation had no effect on energy and nutrient digestibility and seemed to require a relatively long adaptation time to improve digestibility, the alteration of the intestinal microbiota in the ileal digesta and feces in the BP group was similar to that in the AB group. This might be the basis for supplemental BP to show a function similar to that of AB. However, the effects of supplemental BP on the intestinal microbiota were inconsistent with those reported in previous studies. In addition, studies on the effect of BP on ileal microbiota at the phylum level and ileal digestibility in pigs are scarce. Therefore, further studies to determine the effects of BP on the ileal microbiota should be conducted to bridge this gap.