INTRODUCTION
Deoxynivalenol (DON; also known as vomitoxin) is one of the most common mycotoxin contaminants of cereal-based food and animal feed. DON also occurs in grain-based products, such as fermented beer, bread, cereal snacks, noodles, and soy sauce [1,2]. This compound is produced by various Fusarium species, including F. graminearum, F. oxysporum, and F. culmorum. Chemically, DON belongs to the type-B trichothecene family and is termed 3α,7α,15-trihydroxy-12,13-epoxytrichothec-9-en-8-one (C15H2006); it is soluble in organic solvents and water [3]. The most important properties of DON are that it is stable under heat, pressure, and acidic conditions. Hence, it withstands food processing procedures and the risk of DON mycotoxin occurrence in food is higher than that of other toxins. The toxicity of DON is mainly due to its hydroxyl groups. The maximum advisory level of DON for human consumption is 1,000 µg/kg in raw grains, according to the USA Food and Drug Administration. However, for pigs, this advisory level ranges from 5,000 µg/kg to 10,000 µg/kg in grain-based feed [4].
DON exerts acute and chronic toxic effects on humans and livestock, and the severity of toxicity differs based on the species and in a dose-dependent manner. Transformation or deactivation of DON by gut microbiota is not more efficient in monogastric animals than in ruminants. In monogastric animals, most of the toxins are absorbed in the small intestine; therefore, less substrate remains for microbial fermentation in the large intestine [5]. Thus, pigs are more sensitive than other farm animals. The primary symptoms include vomiting, abdominal pain, anorexia, diarrhea, weight loss, and other immunological effects. Ingestion of low to moderate dosages causes gastroenteritis and body weight loss, whereas higher dosages lead to tissue and organ damage, resulting in shock and death [6]. Long-term dietary exposure to low dosages of DON reduces daily food consumption and alters nutritional efficiency. At the molecular level, transcription, translation, the tricarboxylic acid cycle, and oxidative phosphorylation are the major pathways affected by DON [7,8]. The main target of DON is the 60S ribosomal subunit, which is responsible for translational mechanisms involved in protein synthesis. DON induces a ribotoxic stress response that activates mitogen-activated protein kinases (MAPKs) and induces apoptosis [9].
Pigs are more sensitive to DON toxicity than mice, poultry, and ruminants. Contamination of feed with 1–2 ppm of DON causes toxicity in pigs, whereas ruminants can tolerate up to 20 ppm [6]. The gastrointestinal tract is the first site of DON exposure, where it potentially interferes with intestinal barrier functions [10,11]. Disruption of the intestinal barrier leads to increased epithelial permeability to toxins, pathogenic bacteria, and viruses. Moreover, it can adversely affect the immune system and gut health, leading to reduced growth performance of production animals [12]. Low dosages can also induce intestinal damage and affect animal health. Jia et al. [13] found that piglets treated with DON at low dosages (1,000.6 µg/kg) showed up regulation of pro-inflammatory cytokines in the jejunum and alteration of gut microbiota; however, no effect on growth performance was observed in that study.
Three strategies have been developed to prevent or reduce the harmful effects of mycotoxin contamination: 1) prevention of contamination in food chains from field to plate; 2) detoxification of mycotoxins in food and feed using physical, chemical, or biological methods; and 3) reducing bioaccessibility or inhibition of mycotoxin absorption in the intestine [14,15]. Various physical, chemical, and biological methods have been used to decontaminate food and feed containing mycotoxins [16]; however, physical and chemical methods are not effective in reducing DON toxicity because of its high stability. Furthermore, these processes are made more disadvantageous by the nutrient loss that the feed undergoes. Therefore, biological degradation of DON is considered a promising approach to reduce toxic effects without compromising the nutritional value of feedstuff. Biological detoxifiers may degrade toxins through chemical transformation of DON into less toxic compounds, by interacting with toxin molecules or by adsorption of toxic substances, thereby reducing the bioavailability of DON in the gastrointestinal tract [17,18]. Among biological detoxifiers, viable microbial cultures, or died microbial cells that can transform or adsorb toxins, represent promising approaches. Several microbial strains isolated from different sources (including the environment), such as Agrobacterium-Rhizobium from soil [19], Bacillus sp. LS100 from chicken intestines [20], bacterial consortia isolated from wheat leaves [21], and a Eubacterium strain from bovine rumen fluid [22], have successfully been used to decompose DON into non-toxic compounds in in-vitro studies. However, few studies have investigated the effects of DON-detoxifying bacterial cultures as swine feed additives.
The microbial community in the intestine can be disturbed by exposure to DON, which may lead to dysbiosis and affect gut health [23]. Several published reviews have focused only on the effects of DON on the gut health of farm animals and humans; however, the potential of biological DON detoxicants should be elucidated with respect to gut health in pigs. Therefore, this review aims to provide insights into biological DON degradation strategies and their effects on pigs.
Common adverse effects of DON exposure in production animals include reduced feed intake and reduced weight gain, which leads to suboptimal animal performance and economic losses in animal production. The effects of DON on growth performance and nutrient utilization differ among species and depend on the duration of exposure, dosage, and source of contamination (natural or artificial). Piglets fed low dosages of naturally DON-contaminated feed at 280, 540, 840, and 1,000.6 µg/kg were not affected in their feed consumption and average daily gain (ADG) during the four-week experimental period [13,24]. Body weight loss mainly depends on DON dosages. In a few studies, low dosages (1–2 ppm) of DON contamination showed significant effects on piglets compared to growing pigs [25,26]. Wang et al. [27] reported that pigs fed 1.28 and 2.89 mg/kg DON showed lower ADG and average daily feed intake (ADFI) than the controls during the 28-day experimental period; however, no difference was observed in the gain-to-feed ratio. Wellington et al. [28] observed the most pronounced weight reduction in pigs fed 5 ppm of DON, compared to pigs fed 3 and 1 ppm of DON in a 6-week trial. Using a linear regression model, a negative correlation of DON intake and ADG was observed. High dosages of DON significantly affect growth performance, and ADG and ADFI were significantly lower in pigs fed 12 mg/kg of DON than in those fed 3 and 6 mg/kg of DON; however, no effect on the gain-to-feed ratio was observed [29]. Reddy et al. [30] also reported that pigs fed a high dosage of DON (8 mg/kg) showed decreased body weight, food conversion rate, and AFDI, compared to the control. Young et al. [31] found that 12 ppm of dietary DON caused almost complete feed refusal, and 20 ppm caused vomiting in young pigs after consumption of a moldy corn diet. Low dosage, artificial inoculation, and short-term exposure to DON did not affect animal performance when compared to natural contamination and high dosages [32]. However, the reduction in body weight during the experimental period was inconsistent among previous studies. Li et al. [33] observed that ADG decreased by 19% in the first week of DON treatment (2.7 mg/kg feed) in growing-finishing pigs. Similarly, growth performance was significantly lower in the first week of the experimental period of a different study [34]. This suggests that pigs may adapt to DON-contaminated diets. In contrast, a different study showed that dietary supplementation with DON did not influence the initial body weight, whereas growth performance was affected after four weeks of the experiment [35].
Various DON detoxifiers have shown promising effects on the growth performance of pigs exposed to dietary DON. The effects of microbial supplements as DON detoxifiers on the growth performance of DON-exposed pigs are summarized in Table 1.
Microbial category | Supplement name | Origin | Experimental period | DON concentration (mg/kg feed) | Effects (compared to toxic group) | Reference |
---|---|---|---|---|---|---|
Bacteria | Bacillus sp. LS100 | - | 9 d | 5 | ADG and feed efficiency increased | [39] |
Bacillus subtilis ASAG 216 | Animal intestine | 6 wk | 3.6 | ADG and ADFI increased | [40] | |
Devosia sp. ANSB714 | Soil | - | 2.85 | ADG increased | [33] | |
Clostridium sp. WJ06 | Intestinal tract of adult chickens | 35 d | 1.9 | No impact | [41] | |
Yeast | Cell wall product | - | 6 wk | 4.8 | No impact | [36] |
Yeast fermentation product | - | 6 wk | 4.8 | ADG increased | [36] |
Supplementation with viable or dead yeast additives reduced the adverse effects of DON on pig growth performance. Cell walls of dead yeast act as mycotoxin adsorbents and prebiotics, which enhance the growth of beneficial gut microbiota, resulting in improved gut health and reduced toxic effects. Furthermore, supplementation with viable yeast cultures may increase fermentation in the intestine of the host and improve gut health. Weaver et al. [36] found that pigs fed yeast fermentation products showed higher daily weight gain and feed intake than pigs treated with mycotoxins (4.8 mg/kg DON and 0.3 mg/kg zearalenone). Similarly, cellular components and viable cultures of bacteria can adsorb and detoxify DON. Peptidoglycans and polysaccharides in the cell walls of Lactobacillus show toxin adhesion capacity, and heat inactivation of bacteria causes increased pore size in peptidoglycans and can enhance the adsorption of DON through cell wall components [37]. Various Lactobacillus strains have shown DON adsorption capability in in-vitro incubation studies [37,38].
Under DON exposure, the intestinal epithelium, particularly in the small intestine, shows typical structures. The monolayer of the epithelium is composed of enterocytes and goblet cells, which form projecting villi and crypts to increase the nutrient-absorbing surface area. Several studies reported that DON-contaminated diets affected the intestinal morphology in pigs, manifested as atrophy and fusion of villi; decreased crypt depth; reduced numbers of goblet cells; higher abundances of lymphocytes; diffuse apical necrosis; and reduced villi-to-crypt ratios in the duodenum, jejunum, and ileum [27,42]. Piglets fed diets contaminated with 1.28 mg/kg and 2.89 mg/kg of DON showed mild to moderate lesions in the intestinal mucosa [27]. Bracarense et al. [43] observed edema in the lamina propria and cytoplasmic vacuolation of enterocytes in the ileum and jejunum in DON-fed piglets. Kolf-Clauw et al. [44] demonstrated that histological lesions, including the flattening of villi, necrosis, and edema induced by DON were time- and concentration-dependent in ex-vivo jejunal explants. The viability of epithelial cells decreases with increasing DON concentration in cultured cells [45] and, morphologically, DON-treated intestinal Porcine Epithelial Cell line (IPEC)-J2 cells show irregular architecture and low densities. High concentrations of DON reduce ribosomes in the cytoplasm, and mitochondria and vacuolization of the endoplasmic reticulum [45].
Several probiotic supplements show protective effects in the intestine. Dietary supplementation of probiotic yeast Saccharomyces cerevisiae boulardii I1079 to DON exposed piglets significantly reduced the severity of villi atropy, and vacuolization of enterocytes [46]. The addition of Clostridium sp. WJ06 to DON-contaminated diets can alleviate intestinal barrier damage in pigs [41]. Ex-vivo incubation of pig jejunal explants with heat-inactivated Lactobacillus plantarum strains can improve histological and morphometric characteristics, compared to DON-exposed samples [47]. A mixture of microbes and other plant bioactive compounds showed positive effects on the gut morphology. Xiao et al. [48] reported that supplementation of composite antimicrobial peptides, which is a mix of bovine lactoferrin, plant defensins, and active yeast, resulted in increased villus height and villus height-to-crypt depth ratio, compared to the respective parameters in DON-fed piglets.
Intestinal epithelial cells, which form a monolayer in the gastrointestinal tract, act as a first barrier for pathogenic and genotoxic substances between the luminal and systemic regions of the gut. The epithelial barrier is composed of epithelial cells and tight junction proteins that maintain barrier functions with respect to the permeation of ions, toxins, and microbes. Intestinal permeability can be measured by recording the paracellular and transcellular passage of ions [49], and transepithelial electrical resistance (TEER) is a good indicator of epithelial integrity. Changes in TEER and fluorescein isothiocyanate-dextran (FITC-dextran) passage are thus indications of abnormal transcellular and paracellular permeability.
Exposure to DON causes reduced gastrointestinal barrier integrity and induces intestinal injury. The cell lines of porcine epithelial cells, such as IPEC-1 (from the small intestine of newborn swine) and IPEC-J2 (from swine jejunum), are promising cell culture models for investigating the effects of mycotoxins and feed additives on pathological or protective mechanisms in vitro. The TEER value is reduced in epithelial cells after DON treatment [50], and Diesing et al. [51] reported that low dosages of DON (200 ng/mL) did not significantly influence TEER, whereas high concentrations of DON (2000 ng/mL) significantly lowered TEER after 72 h of incubation. TEER values decrease in a time- and dose-dependent manner in IPEC-1 and Caco-2 cells [52], and Springler et al. [12] observed a significant reduction in TEER values in differentiated IPEC-J2 cells at DON concentrations above 5 µM. Similarly, Pomothy et al. [53] reported that 8 μmol/L of DON significantly reduced TEER values in IPEC-J2 cells, and Pinton et al. [52] observed increased basal Paracellular FITC-dextran passage in the mid-jejunal segments of DON-treated pigs.
The intestinal barrier is formed by a single layer of enterocytes and multiple protein complexes termed “tight junctions” [54]. Tight junctions are composed of transmembrane proteins such as claudin; occuldin; tricellulin; and intracellular plaque proteins including zonula, occluden, and cingulin [54,55]. These tight junctions restrict the entry of pathogens, toxins, and other undesirable chemical compounds through the intestinal epithelium. DON exposure disrupts epithelial tight junctions by decreasing tight junction protein levels, leading to increased permeability, and decreased intestinal barrier integrity (Fig. 1). Recently, Li et al. [39] reported that DON increased endocytosis and decreased tight junction proteins by activating the p38 MAPK and c-Jun-N-terminal kinase signaling pathways. Influence of DON on expression of tight junction proteins in epithelial cells and pigs intestine tissue are shown in Table 2.
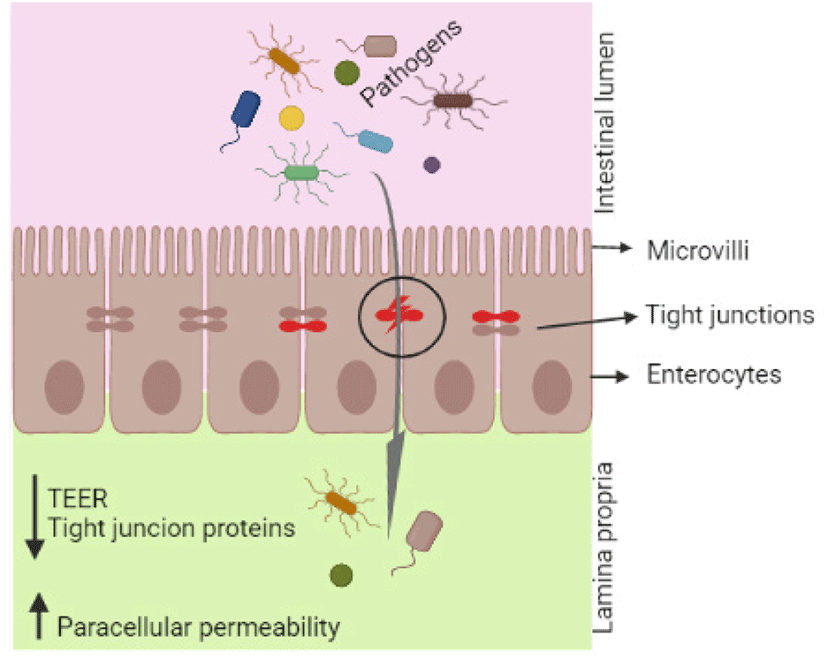
Study | Samples | DON concentration | Effects | Reference |
---|---|---|---|---|
In vitro | IPEC-1 | 0.2 to 2 µM | Reduction of ZO-1 protein in higher concentration | [7] |
IPEC-J2 | 0.2 to 2 µM | Reduction of ZO-1 protein in higher concentration | [7] | |
IPEC-1 | 10, 30 µM | Claudin-3,4 reduced at high concentration of DON | [52,56] | |
IPEC-J2 | 2 µM | Decreased ZO-1, occludin and claudin-3 expressions | [57] | |
In vivo | Pigs jejunum | 2.85 mg/kg feed | 40% decrease of claudin-4 expression | [52] |
Pigs ileum | 3.5 mg/kg feed | mRNA expression of claudin-3 and 4 and occluding were decreased | [58] | |
Pigs jejunum | 1.006 mg/kg feed | Decreased claudin-4 expression | [13] | |
Pigs ileum | 3 mg/kg feed | Decreased expression of E-cadherin and occluding junction proteins | [43] |
DON detoxifying microbial cultures and individual strains have been isolated and investigated in in-vitro studies [20,59]. However, few microorganisms have been tested as feed additives for pigs. Probiotics are promising candidates to alleviate the toxic effects of mycotoxins by modulating gut microbial composition and immunity [60]. Supplementation with yeast cultures as feed additives can exert beneficial effects on DON detoxification and animal health [36,61]. Cell wall components of yeast, including mannans, glucans, and chitin, act as prebiotics, while adsorbents can modify gut microbial communities, resulting in improved barrier function and gut health [62].
The intestinal lamina propria contains innate and adaptive immune cells, such as B cells, T cells, and macrophages, which play an important role in maintaining intestinal homeostasis [49,54]. Consumption of DON by pigs results in various adverse (immunotoxic) effects, which may lead to immunostimulatory or immunosuppressive processes [9,63]. Some studies showed that DON can up regulate inflammatory genes, cytokines, and chemokines, leading to abnormal immune functions and adverse effects on health status [64,65]. According to a recent study, DON-treated IPEC-J2 cells showed 480 differentially expressed genes (320 upregulated and 160 downregulated genes) [66], and most of these genes were associated with immune-related pathways such as the NF-kappa B signaling pathway, the tumor necrosis factor (TNF) signaling pathway, and the MAPK signaling pathway. Moreover, DON impairs the expression of host defense peptides in IPEC-J2 cells and weaned piglets [27].
DON exposure can potentially affect the structure and function of epithelial cells in the intestine, and it can induce inflammation and apoptosis in intestinal epithelial cells [67]. Different studies showed that DON treatment of epithelial cells influences the intestinal barrier integrity and induces abnormal expression of inflammation- and apoptosis-related genes such as those encoding interleukin-6 (IL-6), IL-1β, IL-17, TNF-α, COX-2, BAK, caspase-3, and caspase-8 in IPEC-J2 cells [45,67]. DON also upregulates the levels of chemokines including CCL2, CXCL2, CCL4, and CCL20 [66,68]. Tables 3 and 4 shows the effects of DON exposer on cytokines in DON-treated intestinal cells and tissue samples.
Animal type | DON concentration (mg/kg) | Sample | Effects | Reference |
---|---|---|---|---|
Piglets | 3.2 | Mid-jejunal mucosa | Increased TNF-α, IL-8, IgA and decreased IgG | [26] |
Piglets | 3.5 | Ileum and Jejunum mucosa | IFNG was increased in the ileum samples while IL-6 gene expression decreased. mRNA expression of IL4 was increased in jejunum samples. |
[58] |
Piglets | 1.006 | Jejunum | Increased TNF-α, IFN-γ, IL-1β, and IL-6 | [13] |
Piglets | 3 | Jejunum and ileum | Increased IL-1β, IL-2, IL-6, IL-12 and MIP-1β expressions in the jejunum Increased TNF-α, IL-1β and IL-6 in the ileum |
[43] |
Pigs (barrows) | 1.28, or 2.89 | Cecum and colon | mRNA abundance of IL-12 in the ceca and colons were down-regulated in 2.89 mg/kg DON fed pigs mRNA expression of IL-6 was lower in cecum samples of 1.28 mg/kg DON fed pigs |
[69] |
Cell type | DON (μg/mL) | Effects | Reference |
---|---|---|---|
IPEC-J2 | 0, 0.5, 1, 2 | Increased inflammation and apoptosis gene expressions in a dose dependent manner (IL-1β, IL-6, TNF-α, COX-2, caspase-3, caspase-8, and caspase-9) | [67] |
IPEC-J2 | 0.125, 0.25, 0.5, 1, 2 | Increased mRNA expressions of IL-1β, IL-6, TNF-α, NF-κB p65, IKKα, and IKKβ with increasing concentration of DON Downregulated the relative expression of IκB-α mRNA |
[45] |
IPEC-J2 | 1, 2 | Gene expression levels of IL-6, IL-1α and TNF-α enhanced | [66] |
IPEC-J2 | 0.5 | Upregulated the relative mRNA abundances of Bax, IL-6, TNF-α, COX-2, IL-8, caspase 3, and NF-κB | [70] |
Probiotic supplementation can ameliorate DON-induced intestinal inflammation by modulating gut microbiota (Fig. 2). Bacillus subtilis has been shown protective effects on DON-exposed intestinal epithelial cells [57].
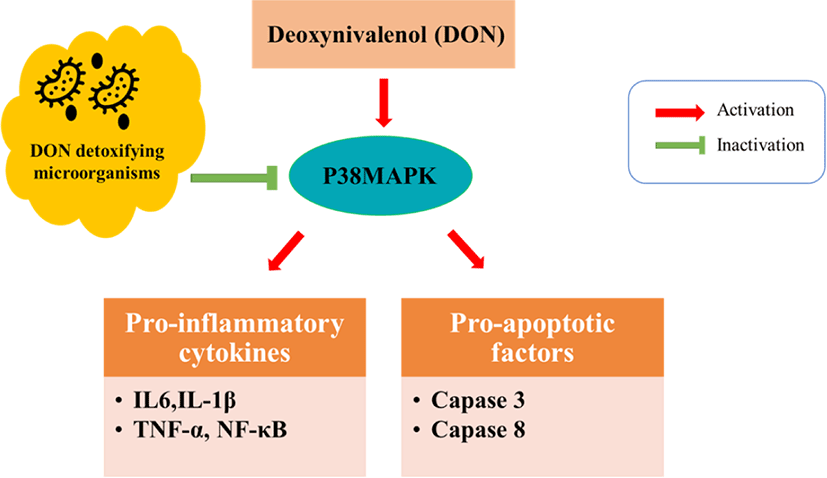
The gastrointestinal tract, which is colonized by a complex bacterial community, is the first site of DON toxicity after consumption of contaminated feed. The intestinal microbiota play a vital role regarding protective, nutritional, and immunological functions by preventing pathogen colonization and the production of fermentation products [71–73]. Therefore, gut health is associated with microbial diversity of the intestinal flora. The mean bioavailability of DON in pigs is 54% under acute exposure and 89% in pigs chronically exposed to DON [74]. Non-absorbed DON is transported to the large intestine and is a potential risk for gut microbial composition at the phylum, genus, and species levels [69,75]. Few studies have investigated the impact of DON on pig gut microbiota composition and diversity using next-generation sequencing [30,35,76]. Bacteroidetes and Firmicutes are the most abundant phyla of intestinal microbiota, and DON-exposed pigs show significant differences between the two dominant bacterial phyla [41]. In contrast, Reddy et al. [77] were not found any difference between Firmicutes and Bacteroidetes abundance in DON fed pigs and control diet fed pigs. DON disrupts the gut microbial balance and increases the pathogenic bacterial count. The abundances of Lactobacillaceae and Bacteroidaceae was higher at the family level in DON-fed pigs than in controls [78], and at the genus level, the relative abundances of unclassified_p_Firmicutes, norank_f_Muribaculaceae, Ruminococcaceae_UCG-01, and Lactobacillus were increased in the colon of DON-fed pigs, compared to the controls [76–78]. Similarly, other studies also found higher Lactobacillus abundance in the ileum and cecum of DON-fed pigs [41]. One of the possible reasons for this is that Lactobacillus can transform toxic DON into less toxic metabolites by enzymatic chemical modification, and species of this particular genus grow faster than other species. In contrast, even low dosages of DON (1.006 mg/kg) decreased Lactobacillus and Bifidobacterium abundance while increasing the abundance of Staphylococcus and Escherichia coli [13,35]. Abnormal intestinal barrier functions lead to an increase in transepithelial passage of E. coli and other pathogenic bacteria [79]. Waché et al. [75] reported that aerobic mesophilic bacteria were significantly increased in fecal samples of pigs fed DON-contaminated diets (2.8 mg/kg). Increased aerobic bacteria can negatively affect intestinal physiology and growth performance. Wang et al. [69] reported that the abundance of Phascolarctobacterium and Ruminococcaceae_UCG-014 decreased, while Prevotella_9 and norank_f_Prevotellaceae abundance increased in the cecal microbiota composition of DON-fed pigs. The populations of Escherichia–Shigella and norank_f_Prevotellaceae were higher in the colonic microbiota of DON-fed pigs [69].
Commensal microorganisms are important for protecting the gut flora and counteracting pathogen colonization caused by DON-induced damage. Probiotic supplementation is a promising strategy for combating mycotoxin-associated toxic effects. Studies demonstrated the positive effects of probiotic supplementation on DON-fed mice and broilers [80,81]; however, few studies have been performed on pigs. Li et al. [41] reported that the abundance of Lactobacillus in the ileum, cecum, and colon was significantly decreased in pigs fed DON-contaminated diets, whereas abundances of Lactobacillus and Clostridium were increased in the pigs’ cecum and colon after consuming Clostridium sp. WJ06-supplemented diets.
Intestinal SCFAs are the metabolites of colonic bacteria. SCFAs such as acetic, propionic, and butyric acids are produced by anaerobic fermentation of non-digestible fibers in the intestine [82]. SCFAs are major substrates for colonocytes in intestinal lumen, these influence the intestinal morphology and immune functions, particularly, butyrate is the important energy substrate for colonocytes, and it promotes the intestinal barrier function through induction of tight junction proteins encoding genes [82,83]. Exposer of DON affects the production of SCFAs in intestine and decreased the butyrate concentrations in piglets colon [84]. Wang et al. [69] reported that DON-contaminated diets (4 mg/kg) induced microbial metabolic disorder in piglets and the levels of acetate, butyrate, and propionate were decreased while the concentrations of isovalerate and other branched chain fatty acids (BCFAs) were increased.
CONCLUSION
In conclusion, ingestion of DON-contaminated feed results in marked toxicity effects on the gastrointestinal tract, with or without impairment of animal growth performance. Moreover, it modulates immune and intestinal barrier functions, leading to reduced animal health and major losses in livestock production. Nevertheless, the use of microbial strains for DON mitigation requires detailed investigation before implementing their practical use as DON-detoxifying feed additives without altering the nutritional value and palatability of feeds.