INTRODUCTION
The circadian rhythm is called the biological clock, and it is derived from the interactions among neuronal clusters of suprachiasmatic nuclei in the anterior hypothalamus situated right above the optic chiasm in the brain [1]. The pineal gland transfers circadian information among neurons in the suprachiasmatic nuclei, including not only the day and night cycle but also other factors affecting the synthesis of melatonin, which could regulate a seasonal circadian rhythm and response to ambient temperature changes [2].
Melatonin, which is mainly secreted from the pineal gland in the brain, is biosynthesized in pinealocytes in the pineal gland [3]. Tryptophan enters pinealocytes and is converted into 5-hydroxytryptamine (5-HT, serotonin) by aromatic amino acid decarboxylase [4]. The balance of 5-HT can regulate day/night homeostasis, and the day:night ratio of 5-HT is higher than 10:1 [5]. Consequently, N-acetyltransferase, which is the rate-limiting enzyme in the reaction of melatonin biosynthesis, is induced when darkness comes, the pineal gland finally starts to make melatonin, and it can join the cerebrospinal fluid to flow into the blood, affecting various physiological functions in the body [6]. Additionally, melatonin is reported to have a diverse biological ability to regulate the light or dark cycle or circadian rhythm, modulate gastrointestinal motility, act as an antioxidant agent, and enhance sedative and immune activators [7]. In the 1950s, the US Food and Drug Administration categorized melatonin as a dietary supplement; however, most other countries have not allowed it as a supplement to be consumed in the current market [8].
Weaning is one of the most stressful conditions in the life of animals, and piglets must undergo inevitable competition for their feed with each other and quarrel over the dominant status among pen mates [9]. This aggressiveness status is reported to have a negative effect on the growth rate throughout the whole life of pigs; however, there is a lack of studies on the correlation between growth performance and fight behavior [10]. Additionally, free-roaming behavior has attracted attention because this behavior is considered to be seeking freedom in terms of animal welfare [11]. However, roaming behavior is considered energy wasting that might be negatively related to the growth of pigs [12]. In 1999, Apeldoorn demonstrated that dietary 40 ppm melatonin supplementation could increase growth performance in broiler chickens by reducing energy expenditure for physical activity [13].
Collectively, we have focused on the sedative effect of melatonin on animal behavior in early weaned rat and pig models. In a rat experiment, we aimed to investigate the antioxidant effects of dietary melatonin on rat. Next, we also investigated the sedative effects of melatonin on early weaned piglets by behavior observation and health status using diverse blood profiles.
MATERIALS AND METHODS
All works related to animals were approved by the Institutional Animal Care and Use Committee (KU-2021-0015) of Korea University.
A total of twenty-four 21-day-old weaned Wistar rats were adopted for a week before the start of the experiment, and the 28-day-old rats were allotted into two experimental treatments and four replications (three rats per cage) according to their body weight ([BW] CON, basal diet; MEL, 50 ppm melatonin-supplemented diet). Rats were raised in a controlled experimental room, and the light cycle was a 12:12 light and dark cycle. The nutrient specifications of the rat feed are presented in Table 1. The BW and feed intake were measured every week, and the average daily gain (ADG), average daily feed intake (ADFI), and feed efficiency (FE) were calculated. All rats were sacrificed by CO2 gas to collect the blood and liver samples. The weight of the liver was measured, and the relative liver weight was calculated. Whole blood was collected by the cardiac puncture method, and serum samples were obtained by centrifugation at 3,000×g and 4°C and stored at −80°C until further experiments. Hepatic tissues were collected to analyze the concentration of malondialdehyde (MDA) and the gene expression level of superoxide dismutase 3 (SOD3) and stored at −80°C until further analysis.
1) Provided per kilogram of complete diet: vitamin A, 12,000 IU; vitamin D3, 2,500 IU; vitamin E, 30 IU; vitamin K3, 3 mg; pantothenic acid, 15 mg; nicotinic acid, 40 mg; choline, 400 mg; vitamin B12, 12 μg; iron, 90 mg; copper, 8.8 mg; zinc, 100 mg; manganese, 54 mg; iodine, 0.35 mg; selenium, 0.30 mg.
One hundred twenty crossbred (Landrace × Yorkshire × Duroc) weaned pigs (weaned at 21 days of age; average BW of 6.00 ± 0.15 kg) were fed for 35 days in three phase feeding programs (Day 0–7, Day 8–21, and Day 22–35). Pigs were allotted into 2 treatments with 6 replications, and each pen had 10 pigs of mixed gender. Pigs had free access to feed and water during the experimental period. The feeding program of pigs consisted of 3 phases (phase I: Days 0–7; phase II: Days 8–21; phase III: Days 22–35). The diet ingredients and specifications was shown in Table 2. Melatonin was supplemented at a dose of 50 mg in a kg of diet. All pigs were weighed weekly, and feed consumption was measured to calculate ADG, ADFI, and FE. Blood samples were collected at Days 0, 21, and 35 from the jugular vein. The collected whole blood was centrifuged at 3,000×g and 4°C, and serum samples were obtained and stored at −80°C until further analysis. The behavior of pigs was recorded using closed-circuit television (CCTV) cameras (SONY HS-652DI 1/3“CCD, NTSC 3.6 mm). The CCTV cameras were installed above the pens, and the video data were collected by a digital video recorder (DS-2008DH stand-alone DVR). All video data were analyzed by using a DVR video viewer program (MDVR Video Manager). The behavior was separated into 4 states, including resting, roaming, feeding, and fighting states, and they were calculated as the percentage of pigs per the representative days of each week (Days 3, 10, 17, 24, and 31).
Serum triglyceride (TG), blood urea nitrogen (BUN), and creatinine were measured in the rat experiment, and serum TG, total cholesterol, BUN, glucose, and creatinine were measured in the pig experiment. Briefly, plasma samples and TG standards (300 mg/dL) were loaded on 96-well microplates, and lipoprotein lipase, glycerol kinase, alpha-glycerol-3-phosphate, peroxidase (POD) and 4-aminoantipyrine (4-AAP) mixed in N-ethyl-N-m-toluidine solution were then added to the well and incubated at room temperature for 5 minutes. Optical density was measured by a colorimetric microplate reader at 550 nm wavelengths. Next, plasma samples and urea standard (30 mg/dL) were loaded on 96-well microplates, and urease mixed in sodium salicylate and sodium nitroprusside buffer solution was added to each well and incubated for 15 minutes at room temperature. After incubation, sodium hypochlorite solution was added to the well and incubated for 10 minutes at room temperature. Optical density was measured by a colorimetric microplate reader at 580 nm wavelengths. Picric acid was added to the plasma sample for deproteinization, and the reactant was centrifuged at 3000 rpm for 10 minutes. The supernatant was removed, and the CRE standard (10 mg/dL) and its diluents were loaded on a 96-well microplate in duplicate using a blank (picric acid). Optical density was measured by a colorimetric microplate reader at 515 nm wavelengths. The total cholesterol concentration was measured according to the manufacturer’s protocol. In summary, plasma samples and T-CHO standard (300 mg/dL) were loaded on 96-well microplates in duplicate, and then cholesterol esterase, cholesterol oxidase, POD and 4-AAP mixed with phenol solution were added to the well and incubated at room temperature for 5 minutes. Optical density was measured by a colorimetric microplate reader at 500 nm wavelengths. Finally, plasma samples and glucose standard (200 mg/dL) were loaded on 96-well microplates in duplicate, and in the next step, mutarotase, glucose oxidase, 4-AAP and POD mixed with phenol solution were added to the well and incubated at room temperature for 5 minutes. The optical density of the end product quinoneimine was measured by a colorimetric microplate reader at 500 nm wavelengths.
The representative indicator of lipid peroxidation, MDA, was measured by TBARS assay. Briefly, 100 mg of each liver sample was weighed, and 500 μL of distilled water was added. The samples were homogenized and centrifuged at 10,000×g for 20 min at 4°C. Then, 125 μL of supernatant was placed into a 96-well microplate, and 125 μL of TBA solution was added. After 15 and 30 hours of incubation, the optical density was measured to calculate the MDA concentration in serum.
Frozen liver samples were homogenized in TRIzol reagent (Invitrogen, Waltham, MA, USA), and the total RNA from each sample was obtained according to the manufacturer’s protocol. Subsequently, cDNA was synthesized by reverse transcription using a high-capacity cDNA synthesis kit (Applied Biosystems, Waltham, MA, USA). The relative mRNA levels of each gene were determined using StepOnePlus (Applied Biosystems) and normalized to β-actin using the 2−ΔΔCt method. The forward primer for the SOD3 gene (5’-TCTCAGGCCTCTAGCTGGGT-3’) and reverse primer for the SOD3 gene (5’-AGGTTCCACACCTGACAAGCTC-3’) were used.
The serum concentration of melatonin was measured by GC‒MS according to Paik’s protocol [14]. Briefly, 800 μL of serum samples was mixed with 800 μL of chloroform and sonicated at 1,500×g for 10 min. After centrifugation, 600 μL of the bottom layer chloroform phase was collected, and sonication was repeated once again for bottom layer collection. The extracted sample was dried, derivatized and injected into the GC‒MS machine. The GC condition was as follows: 140°C to 315°C (15°C/min) of the oven temperature with holding 2 minutes, 300°C of detector, 250°C of injector, 1/10 of split ratio, 13 psi of pressure, 40 mL/min of purge flow, 0.5 mL/min of purge time, 1 mL/min of flow rate, helium as a carrier gas, 35 kPa of inlet pressure, 40–450 a.m.u for 1 scan/s and 70 eV for the MS detection. A capillary column was used in the specification as follows: HP-5MS, 30 m × 0.25 mm internal diameter, 0.25 µm.
RESULTS
The BW of the rats were significantly higher in the melatonin-supplemented group at Days 21 and 28 than in the control group (p < 0.05); however, the ADG, ADFI, and FE during the overall experimental period were not changed by dietary melatonin supplementation (Table 3). The relative liver weight of rats fed the melatonin-supplemented diet was significantly heavier than that of rats fed the basal diet (Fig. 1A, p < 0.05). In addition, the hepatic concentration of MDA was significantly decreased by dietary melatonin supplementation (Fig. 1B, p < 0.05); however, melatonin ingestion did not change the gene expression level of SOD3 compared to that in the CON group (Fig. 1C). The concentrations of TG and creatinine were not different between the CON and MEL groups; however, the concentration of BUN was significantly lower in rats fed the melatonin-supplemented diet compared to the CON group (Figs. 2A, 2B, and 2C; p < 0.05).
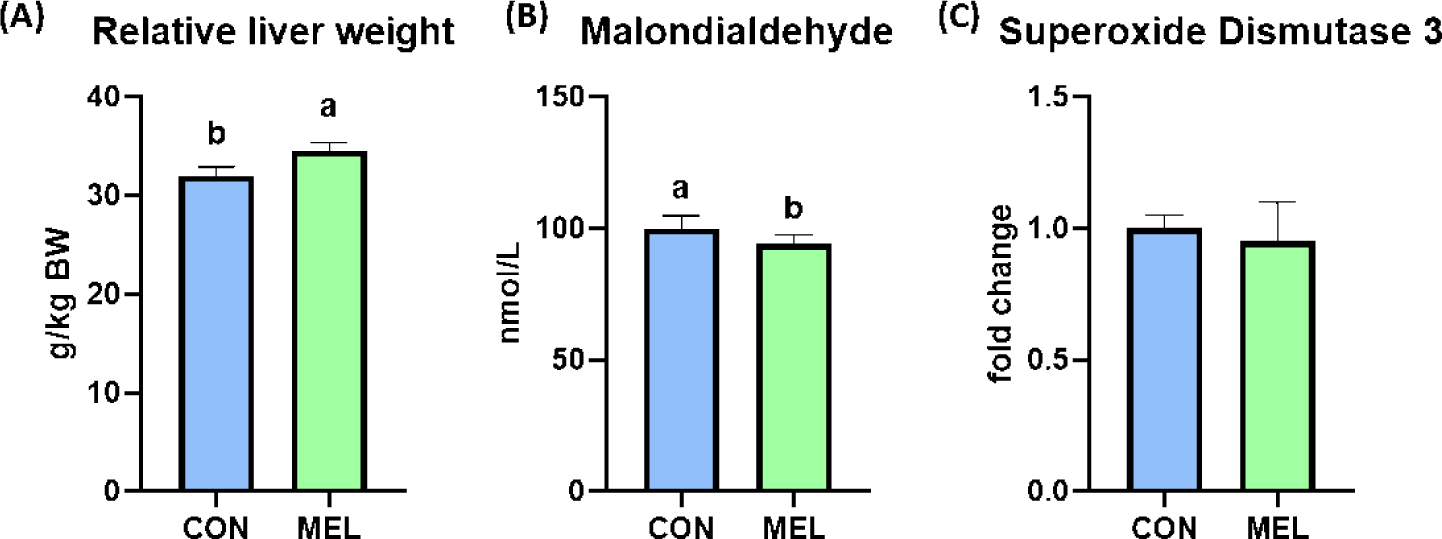
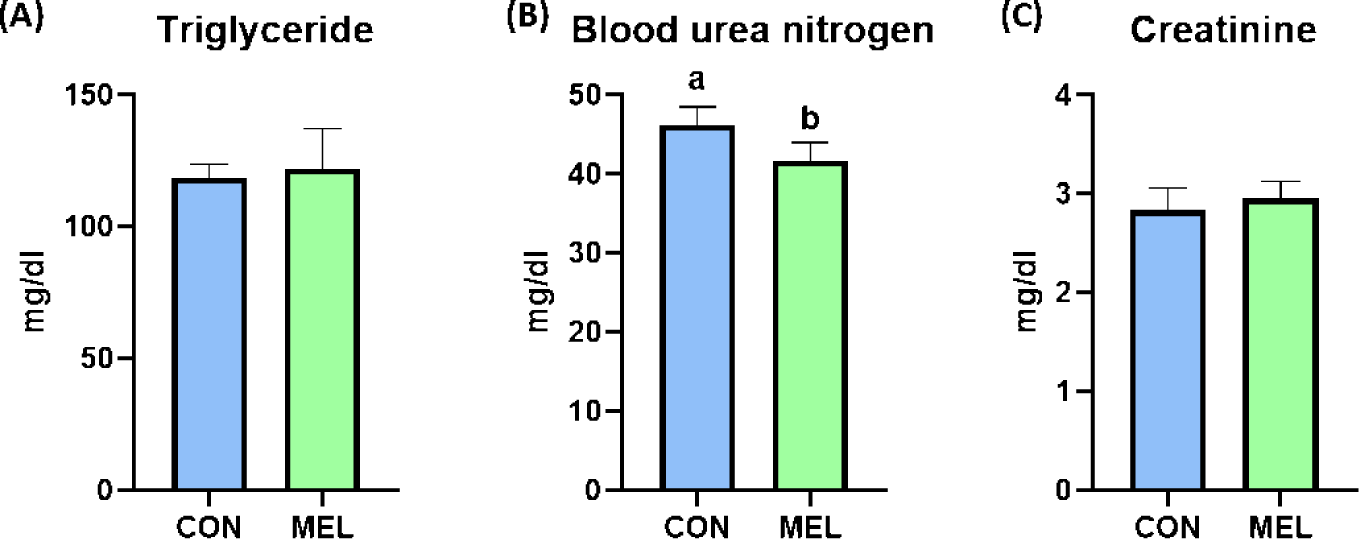
After weaning, the BW of piglets was significantly improved at Day 28 by dietary melatonin supplementation (p < 0.05), and the final BW of pigs fed the melatonin-supplemented diet were numerically increased compared to the control group. During the whole experimental period, the ADG and ADFI were not changed by dietary melatonin supplementation, and FE was also not changed by dietary melatonin supplementation (Table 4). At Days 21 and 35, the concentration of melatonin in serum was not different as a result of dietary melatonin supplementation (Fig. 3A). Additionally, dietary melatonin supplementation did not change the concentrations of TG, total cholesterol, glucose, BUN, and creatinine between pigs fed the basal diet and those fed the melatonin-supplemented diet. However, the concentrations of TG and total cholesterol gradually decreased at Days 21 and 35 compared to the concentrations at Day 0 (Figs. 3B, 3C, 3D, 3E, and 3F). During Days 8–21, roaming behavior was significantly decreased by melatonin supplementation, and fight behavior was also significantly reduced in pigs fed the melatonin-supplemented diet compared to the basal diet group (Fig. 4A, p < 0.05). Consequently, this change led to a significant increase in resting behavior and feeding behavior in pigs fed the melatonin-supplemented diet compared to the control group during Days 8–21 (Figs. 4B and 4C, p < 0.05). Additionally, resting behavior was also significantly increased during Days 22–35 in the MEL group compared to the CON group (Fig. 4B, p < 0.05). The fight state was significantly decreased during Days 8–21 in the melatonin-supplemented group compared to the CON group (Fig. 4D, p < 0.05).
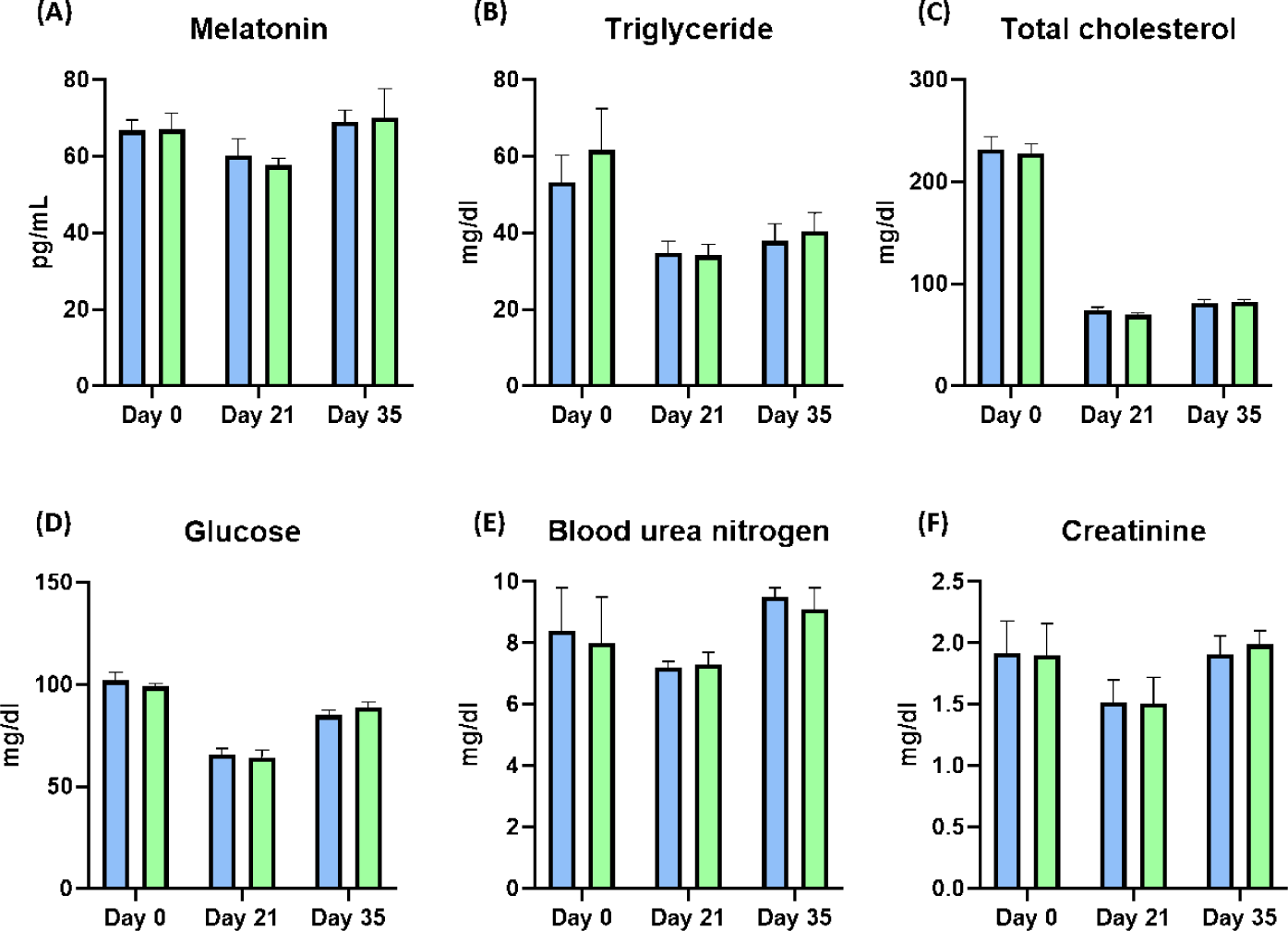
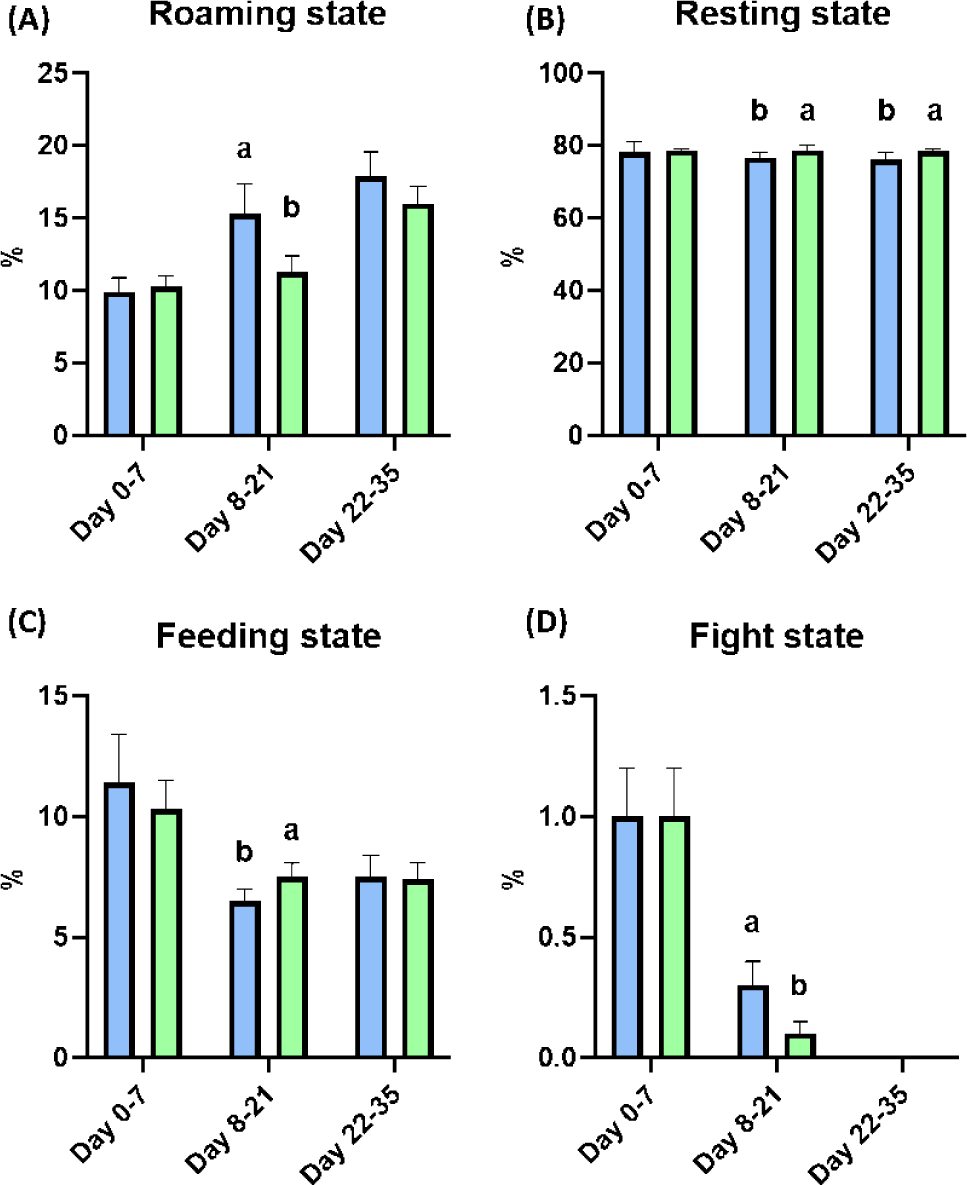
DISCUSSION
Melatonin, an indole-based compound, is involved in the entrainment of circadian rhythms and various behavioral and physiological events in the animal’s body [15]. After synthesis of melatonin in the pineal gland, the blood concentration of melatonin is spiked quickly, and it spreads in bile, cerebrospinal fluid, and amniotic fluid [16]. Consequently, melatonin could act as an immunomodulator in neuroendocrine and physiological processes, and it could modulate angiogenesis and wound healing capacity [17,18].
In this study, we investigated the antioxidant capacity and sedative effects of melatonin in rat and pig weaning models. The results of this study indicated that dietary melatonin supplementation showed advanced hepatic antioxidant activity and could lead to improvement of protein bioavailability by increasing serum BUN levels. Additionally, dietary melatonin supplementation could significantly improve the behavior of piglets after weaning by increasing the resting and feeding state in phase II with reduced roaming and fight states of piglets. Collectively, dietary melatonin supplementation could improve animal growth performance by restoring the leaked energy expenditure from antioxidant activity and useless movement, including roaming and fighting, in the piglet stage.
The antioxidant activity of melatonin is one of the main characteristics of its application approach for improvement in animal health [19]. Ingested melatonin is able to reach the hepatic portal vein and can aid various physiological and metabolic activities depending on the metabolic requirements of the animal [20]. In particular, melatonin can act in the liver as a strong radical scavenging agent via transformation to other compounds, including N-acetyl-5-methoxykynuramine, cyclic 3-hydroxymelatonin, and N-acetyl-N-formyl-5-methoxy-kynuramine [21]. Consistent with this study, dietary melatonin supplementation in weaning rats could decrease hepatic MDA concentrations, which might indicate that dietary melatonin could improve antioxidant capacity in the liver. MDA is a major secondary oxidation product of peroxidized polyunsaturated fatty acids [22]. It has been widely used as a biomarker related to lipoperoxidation in biological and medical science fields and applied as an index of lipid oxidation and rancidity in the food and feed industry [23,24]. In 2020, Moryaridzadeh also demonstrated that melatonin intake could relieve oxidative stress in randomly selected unhealthy people with significantly lowered MDA levels without affecting nitric oxide levels and catalase activity [25]. Therefore, our results also suggested that dietary supplementation with melatonin could relieve the stress from weaning by improving the hepatic antioxidant status.
Fight behavior in early weaned piglets is the dominant problem in pig raising systems because fighting can reduce ADG and FE by upregulating skin damage and stress in ricks [26,27]. Indeed, fight behavior also significantly elevates heart rate and could lead to increased pig mortality and respiratory disease incidence with decreased backfat thickness [28,29]. However, various studies have demonstrated that fight behavior is necessary in pig society because the establishment of a new hierarchy is the instinct of pigs [30]. In 2002, Bubenik and colleagues demonstrated that feed intake increased the level of melatonin in the gastrointestinal tract and might lead to sleep or resting behavior [31]. In coincidence with this study, our results also suggested that dietary melatonin supplementation could reduce energy wasting by roaming and fight states with significant increases in resting and feeding behavior during Days 8–21 after the social hierarchy was established.
CONCLUSION
Collectively, our study demonstrated that dietary melatonin addition could accelerate the growth of rats and pigs after 4 weeks by improving antioxidant capacity. Additionally, dietary melatonin could reduce fight behavior after pig hierarchy establishment and could increase feed intake and sleep or resting behavior to minimize energy-wasting behaviors.