INTRODUCTION
The sensory properties such as taste, flavor, and tenderness are among the most important determinants of meat purchase by consumers [1–3]. Several studies have shown that consumers are willing to pay more for better-quality meat [4–6]. Post-slaughter aging is an essential process to enhance the sensory properties of meat through the action of proteolytic systems inherent in meat. Industrially, several methods are used for the aging of meat to enhance its value. These methods range from traditional carcass hanging to storing vacuum-packed meat at refrigerated temperatures for a certain period. In general, two techniques are used for meat aging, i.e., dry-aging and wet-aging. Wet-aging has the advantage of convenience while dry-aging has the advantage of conferring excellent sensory properties [7–9].
Although aging generally improves the sensory properties of meat, the specific conditions for maximizing the sensory properties according to the aging method have not been fully established. Therefore, it is important to investigate the optimal aging conditions by exploring the rate and extent of the aging effect according to the aging method to improve meat quality and value. From that perspective, this review summarizes the underlying molecular mechanisms by which aging induces changes in meat quality and discusses the mechanisms and factors for improving the sensory properties of aged meat. During aging, the natural enzymes in the meat break down the proteins and connective tissue, increasing the tenderness of meat [10,11]. Moreover, during the dry-aging process, meat juice is further concentrated in meat and the chemical breakdown of protein and fat constituents creates a more intense nutty and meaty flavor [12]. However, the dry-aging process is more expensive and time-consuming than the wet-aging process due to high aging shrinkage, trim loss, contamination risk, and requirements for aging conditions and space [13,14].
The aging process improves both the tenderness the taste characteristics of meat. The improvement of the savory taste of meat is largely attributable to the increased content of amino acids related to the umami, such as glutamic acid and aspartic acid, caused by proteolysis [15]. With the recent advances in omics analysis techniques, several studies have investigated the mechanism of the breakdown of meat proteins and the increase of taste-related substances due to aging [16–19]; however, the underlying mechanisms are not well characterized. In addition, novel technologies or new aging techniques are being developed and applied to enhance the effect of meat aging [10,11,20,21]. However, there is a lack of review related to the increase in sensory properties. Therefore, this review summarizes the available evidence regarding the molecular mechanism of the degradation of proteins and the changes in meat quality and taste characteristics during postmortem aging.
MECHANISM OF POSTMORTEM AGING ON CHANGES IN MEAT QUALITY
Several reports have described significant biochemical and biophysical changes during muscle conversion to meat, and these changes have a direct effect on meat quality [22–25]. During the postmortem aging process, cytoskeletal myofibrillar protein degradation by endogenous proteases results in significant improvements in the sensory properties of meat [25]. Meat color, water-holding capacity (WHC), tenderness, and texture are the major quality attributes of meat [1]. Tenderness is the most important attribute influencing beef palatability [25,26] while WHC is the most important attribute for the sensory properties of pork [1,27]. Therefore, in this respect, improvements in tenderness and WHC have been extensively studied in beef and pork, respectively, in relation to the development of aging techniques [11,21].
Proteolysis is a major factor in improving meat quality traits such as tenderness and WHC [26,27]. Several factors influence the rate and extent of proteolysis such as species, breed, animal age, diet, individual muscle, marbling content, and aging method [17,28–31]. The effect of aging on the tenderness of beef has long been studied, and many theories have emerged, such as those related to calpain, calcium ion, and cathepsin [32]. Among these theories, the calpain system has received much attention and is considered a major cause of proteolysis during postmortem storage. Proteolysis of myofibrillar proteins has been reported to be the main cause of improvement in meat tenderness during postmortem storage [33]. Specifically, the weakening of Z-disks and degradation of desmin, titin, troponin-T, and nebulin increase the fragility of myofibrils [34–36]. As shown in Fig. 1, the mechanism by which the calpain system affects meat tenderness is summarized into four points. First, calpain weakens the interactions between myofilaments and the Z-disk with the breakdown of titin and nebulin and fractures the I-band and Z-disk in myofibrils, loosening the microstructure of myofibers [35]. Second, the calpain breaks down costamere and desmin, deranging the orderly structure of myofibrils or the integrity between myofibrils and peripheral muscles [37]. Third, calpain plays a decisive role in the degradation of tropomyosin, weakening the bond between thick and thin filaments [38]. Fourth, calpain degrades troponin-T, a tropomyosin-binding subunit, weakening the structure of thin actin filaments [39].
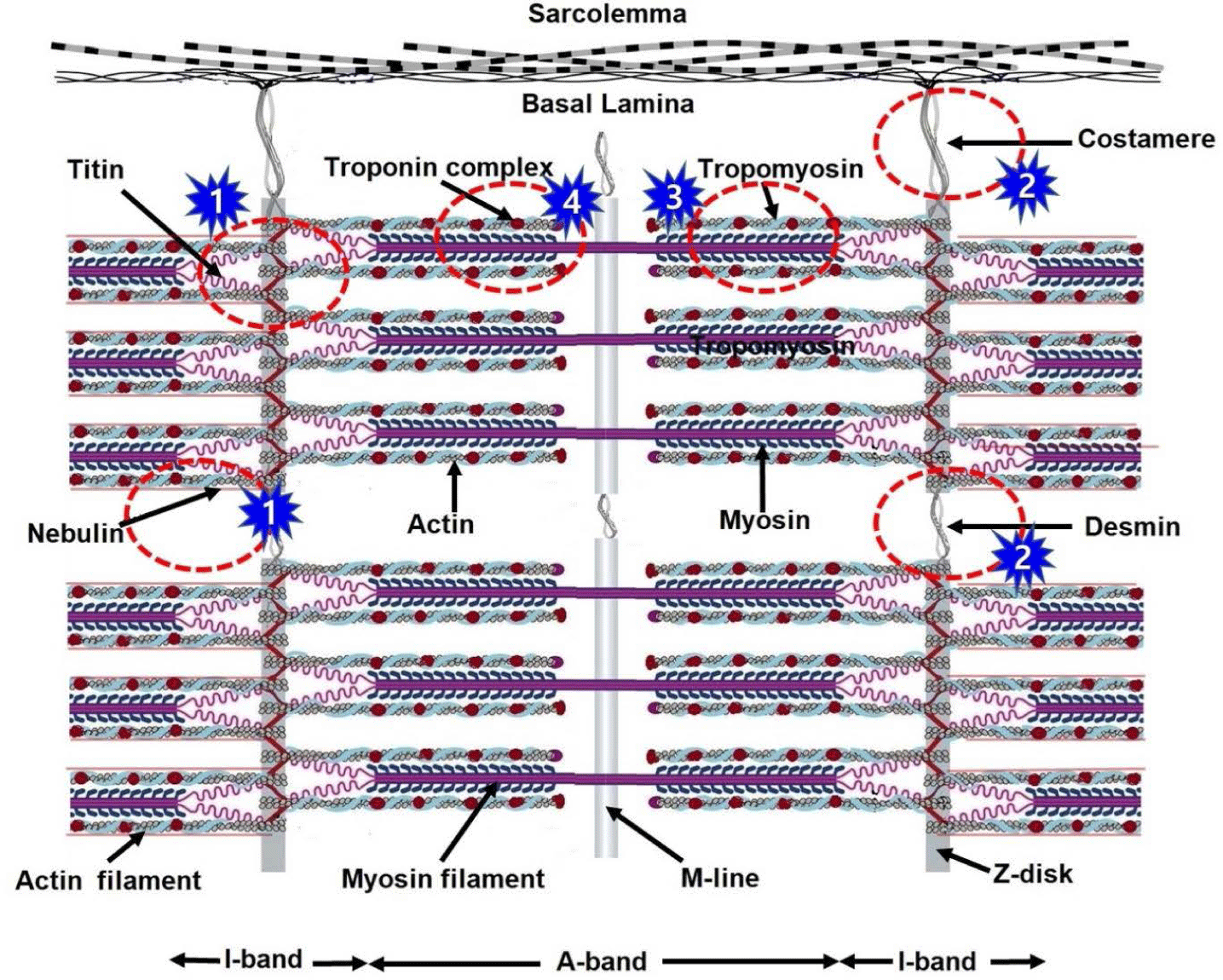
In general, there is a rapid change in tenderness between 3 and 7 days postmortem, after which the rate of change in tenderness slows significantly [36,40]. However, in the case of beef produced from innate tough muscles or old cattle muscles, some reports suggest that tenderness may gradually improve up to 28 days postmortem [8,41–43]. The aging-induced improvement in tenderness is attributable to the decrease in mechanical strength of the intramuscular connective tissue due to proteolysis caused by endogenous enzymes [10,44–46]. This decrease in mechanical strength is mainly caused by an increase in collagen solubility and dissociation of the structural integrity of muscle connective tissue [15,47,48]. The strength and structural integrity of collagen fibrils, usually stabilized by proteoglycan, degrade with the progression of postmortem aging. This leads to further exposure of the active sites of potential degradative enzymes, such as lysosomal glycosidase or β-glucuronidase, further weakening the structural integrity and making the meat tender [15].
Recent studies have further clarified the tenderizing mechanism of postmortem aging. A schematic illustration of the newly proposed muscle aging mechanisms is presented in Fig. 2. Tenderizing of postmortem muscle is driven by the calpain system, which depends on the concentration of Ca2+ in the sarcoplasm [49], and the increase in Ca2+ concentration in postmortem muscle is due to apoptosis [50]. Postmortem aging generates reactive oxygen species (ROS) which induce oxidative stress and apoptosis [51]. Some of the apoptotic proteins released from mitochondria in response to ROS participate in regulating apoptosis [52]. These apoptotic enzymes participate in the early stages of muscle aging, leading to the degradation of titin and nebulin, as well as regulation of the Ca2+-activated enzyme system [53–55]. Activation of apoptotic enzymes such as caspase-3 by denitrification induces apoptosis for myofibril fragmentation, as well as direct proteolytic activity against calpastatin [56–58]. Moreover, chaperone proteins such as small heat shock proteins (sHSPs) have an anti-apoptotic effect [59]. sHSPs delay the postmortem tenderizing process by inhibiting the onset of apoptosis by directly binding to key proteins in the apoptotic cascade such as cytochrome c and caspase-3 [60]. On the other hand, calpain is a cysteine protease, and the cysteine residue at the active site can be modified by protein S-nitrosylation, which consequently affects its autolysis and proteolytic activity [61,62]. Protein S-nitrosylation modifies the release channels of Ca2+, affecting the rate of Ca2+ release and resulting in muscle contraction and altered moisture distribution in myofibrils [63]. In addition, S-nitrosylation inhibits the activity of enzymes such as phosphofructokinase involved in postmortem glycolysis, affecting the rate of decline in pH, ultimate pH, and meat quality traits including tenderness [64].
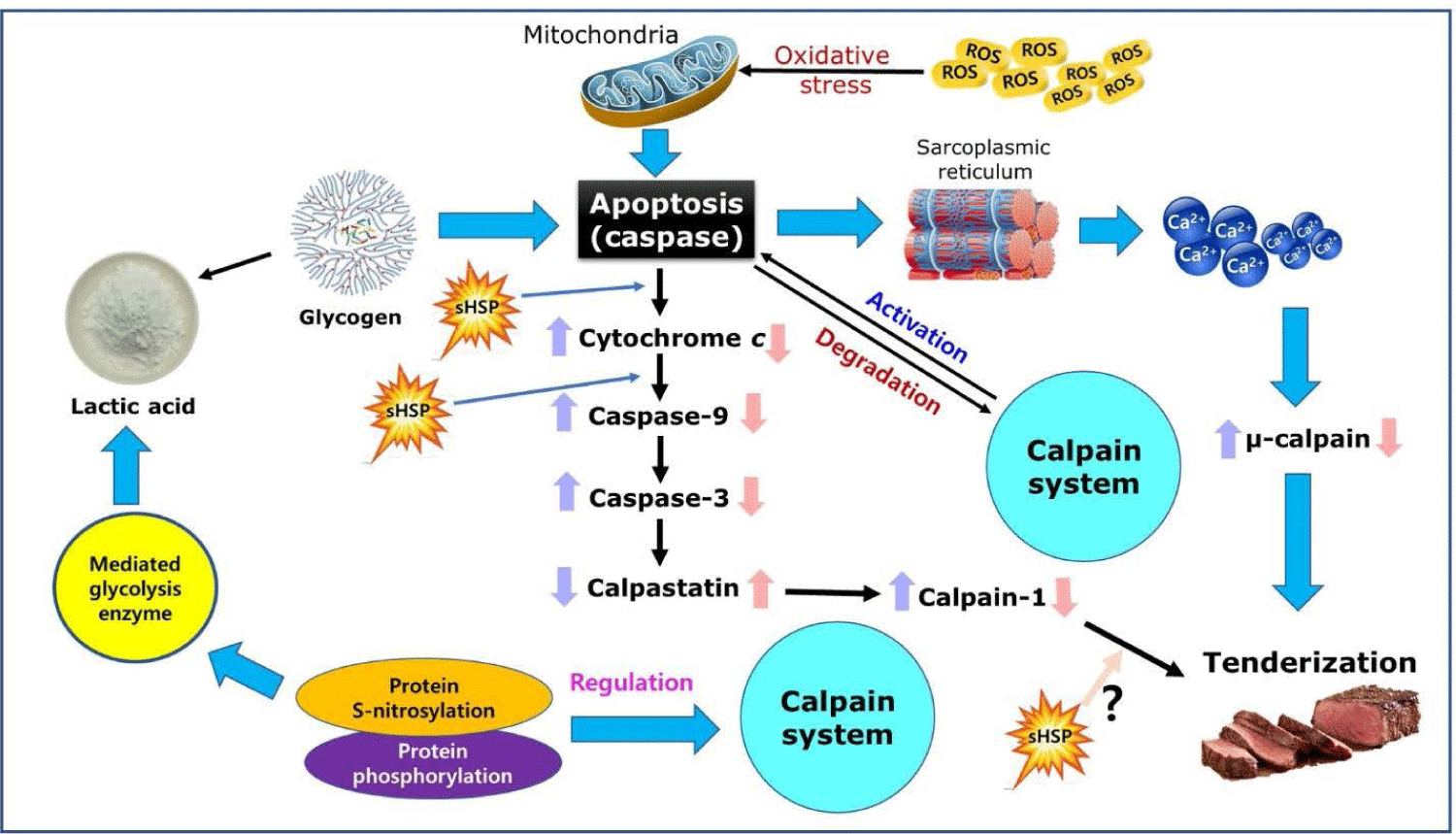
WHC is one of the most important quality traits of fresh meat because it is closely related to meat color, texture, and tenderness [1,11]. An increase in water loss is unavoidable due to the occurrence of rigor mortis in the process of conversion from muscle to meat. The formation of crosslinks between thick and thin filaments within the myofibrils stiffens the muscle fibers and leads to the extrusion of intracellular water from the myofibers [65]. Subsequently, with the resolution of rigor and initiation of postmortem aging, intracellular water continues to move to the surface of the meat and is observed in the form of a purge or drip. However, long-term aged meat often shows improved WHC due to the degradation of proteins. Postmortem proteolysis of structural/cytoskeleton proteins, including desmin, titin, nebulin, and integrin, is associated with the improvement of WHC [66–68]. Changes in the microstructure of muscle fibers during postmortem aging are believed to improve WHC. First, during postmortem aging, degradation of costamere linkages reduces myofibril shrinkage, resulting in more space within muscle fibers to retain water [65,68,69]. In addition, the so-called ‘sponge effect’ occurs wherein the myofibrillar proteins break down and disturb the drip channels, resulting in water trapping within the myofiber [70]. This is the likely underlying mechanism by which aging beef improves the juiciness of steak [8,71,72].
However, the relationship of juiciness of aged meat with tenderness and WHC has not yet been clearly identified. Several studies have shown a positive correlation between sensory tenderness and juiciness [73,74]. Therefore, the improved juiciness of aged meat is likely attributable to the synergistic effect due to the increase in sensory tenderness [10]. Many sensory studies and consumer surveys have reported a positive correlation between tenderness and juiciness of meat; however, the coefficient of determination (R2) was not high enough and varied depending on the species or muscles [75]. Thus, although there is less correlation between objective shear force measurements and sensory tenderness of cooked meat, a positive correlation between sensory tenderness and juiciness can be inferred. In this respect, some studies have proposed the so-called ‘halo effect’ whereby improved tenderness increases the perception of juiciness, and vice versa [76,77]. Indeed, there is an increase in WHC associated with the swelling of myofibers during postmortem aging, but this does not lead to lower cooking loss [78]. This is because aged meat not only causes pronounced shrinkage of myofibers during cooking but also exhibits a significant decrease in myofibrillar water after cooking. The water lost during cooking is higher in meat aged for at least 3–6 days than unaged meat, but this depends on the aging period [79–81]. Compared to un-aged meat, the increase in cooking loss in aged meat varies depending on the pre-rigor temperature conditions of muscles and sarcomere length [82]. In aged meat, weakened protein structure appears to be unable to retain or trap water during cooking because the swelling of muscle fibers is limited due to the degradation of myofibrillar and cytoskeletal proteins [83]. However, even if the cooking loss of aged meat is high, a recent study showed that juiciness is improved at the same time as the early activation of calpain-2, suggesting that postmortem proteolysis may play a role in improving the juiciness of aged meat [84].
The meat color, color stability, and WHC of meat undergo significant changes during postmortem aging. The surface redness of aged meat is initially improved compared to non-aged meat or relatively short-term aged meat [27,85]. The temporary improvement in the redness of the aged meat surface is due to a decrease in oxygen consumption of respiratory enzymes within mitochondria. However, with the prolongation of the aging period, the oxidative stability of the myoglobin or lipid eventually deteriorates. Extended aging period under lighting conditions of meat retailers accelerates surface discoloration and promotes off-flavor generation [86,87,88]. Even if aging improves the eating quality of meat, discoloration due to metmyoglobin and darkening due to surface dehydration as a result of extended aging will inevitably cause economic losses [89,90]. The negative effect of extended aging on meat color and oxidative stability is due to the accumulation of pro-oxidants (heme and non-heme iron) and the depletion of endogenous reducing compounds (NAD+, α-Tocopherol, and β-Carotene) or antioxidants (acylcarnitines, nucleotides, nucleosides, and glucuronides) [91,92,93].
CHANGES IN TASTE CHARACTERISTICS OF MEAT DUE TO AGING
Postmortem aging causes a significant increase in meat flavor. This phenomenon is related to the reducing sugars, the release of free amino acids and peptides, and the increase in the content of inosine monophosphate (IMP), guanosine monophosphate (GMP), inosine, and hypoxanthine due to the breakdown of ribonucleotides [94–96]. In addition, flavor enhancement in aged beef is associated with the production of other flavor-related volatile compounds such as n-aldehydes (e.g., pentanal and hexanal) and ketones, which also contain lipid oxidation-related products [10,12,97]. These flavor precursors interact with each other throughout the cooking process, generating new flavor components [12]. Therefore, the development of meat flavor can be considered as a dynamically evolving process, as illustrated in Fig. 3.
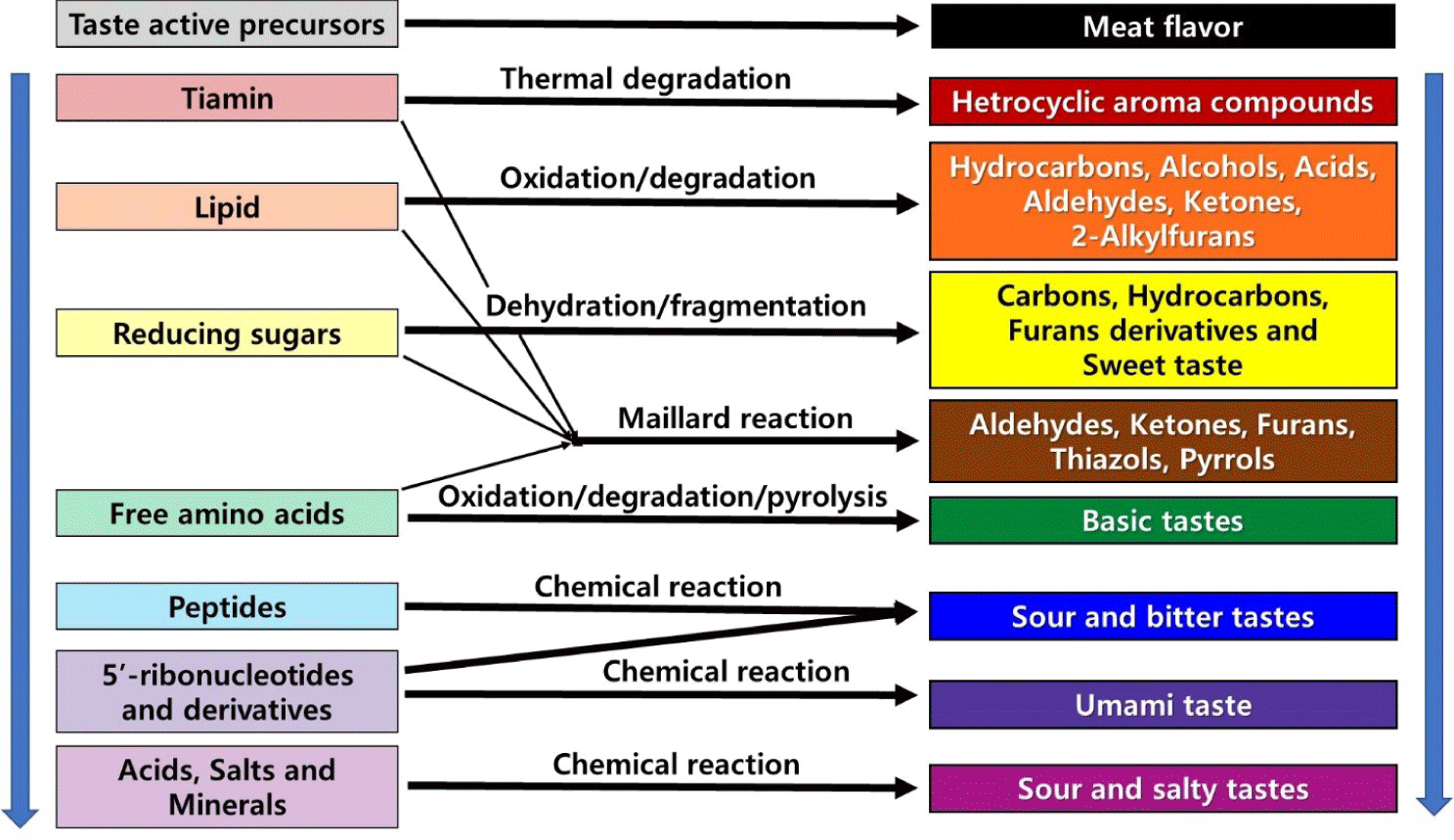
The improvement of meat taste characteristics during aging is mainly due to hydrolysis activity. In addition, the activity of various hydrolases such as calpain, which fragments the muscle structure, and cathepsin, which is involved in the production of taste peptides, also plays an important role in improving taste characteristics [95]. During the longer aging period, more taste-related peptides and free amino acids are broken down due to the enzymatic activity in meat. Among them, aliphatic amino acids are related to the sweetness of meat while Cys and Met, containing a sulfur atom, and Glu and Asp are associated with the umami taste [98]. Furthermore, during aging, carbohydrates are broken down into sugars, enhancing the sweetness of meat, and fats and fat-like membrane molecules are broken down into aromatic fatty acids. All these end-products produced during postmortem aging contribute to the intensity of meat aroma, nut-like flavor, and umami taste of cooked aged meat [98,99].
The taste characteristics of aged meat, such as umami intensity or flavor, are not determined by any single factor, but rather by the complex interaction between sulfur-containing amino acids, aspartic acid, glutamic acid, nucleotide compounds, and β-histidyl dipeptides [98,100]. Moreover, postmortem energy metabolism also affects the taste of meat by causing an increase in sugar fragments through the degradation of glycogen content, resulting in an increase in the substrate for the Maillard reaction [101]. In addition, prolonging the aging period to > 28 days was found to considerably increase the aromatic volatile compounds [59,102]. While it is generally agreed that aging improves meat flavor, prolonged aging may adversely affect the flavor. Aging of beef for 4 days at 4°C desirably improves the sweetness and beefy flavor; however, further prolongation of the aging time may increase undesirable taste characteristics such as bitterness and sourness [95]. In addition, on prolonged aging, free fatty acids (FFAs) that are easy to oxidize are released, which react with proteins and other flavor precursors to negatively affect the aroma and/or flavor of aged meat [103]. Therefore, controlling the appropriate aging method is necessary to maximize the desirable taste and flavor of aged meat and minimize the off-flavor and off-odor.
Several peptides that are released during proteolysis in aging meat affect the taste characteristics. These peptides show different taste characteristics depending on the specific size (i.e., fraction). The small peptides (< 5 kDa) that are most noticeable and reproducible during postmortem aging are fragments of troponin T, nebulin, pro-collagen, and cipher proteins [104–106]. In particular, 1- to 5-kDa peptides, so-called Maillard peptides, and 3- to 10-kDa peptides were found to improve the flavor and taste intensity of grilled beef [107,108]. In addition, 1- to 10-kDa and 0.5- to 1-kDa fractions significantly inhibit the sourness of beef and pork [109,110].
In the past few decades, many peptides related to the taste characteristics of meat have been reported. The content of oligopeptides increases during the refrigerated aging of meat. Among the oligopeptides, glutamic acid especially improves the savory taste of beef [111]. Octapeptide (Lys-Gly-Asp-Glu-Glu-Ser-Leu-Ala), called “beefy meaty peptide”, also occurs naturally during postmortem aging and is responsible for the delicious taste of beef [112]. In addition, the peptides (Glu-Glu, Glu-Val, Ala-Asp-Glu, Ala-Glu-Asp, Asp-Glu-Glu, and Ser-Pro-Glu) found in chicken are related to umami intensity, and the peptides (Glu-Asp-Glu, Asp-Glu-Ser, and Ser-Glu-Glu) found in fish hydrolysates are related to savory taste [113]. The peptide (Ala-Pro-Pro-Pro-Pro-Ala-Glu-Val-His-Glu-Val) found in pork suppresses sourness [110].
On the other hand, there is no clear consensus on the effect of naturally occurring dipeptides produced during aging on the taste characteristics. These dipeptides include carnosine, β-alanyl-L-histidine; anserine, β-alanyl-L-1-methylhistidine; balenine, β-alanyl-L-3-methylhistidine. Some studies have found a positive effect of these dipeptides on the taste characteristics of meat [114]. However, other reports suggest that anserine and carnosine produce bitterness if the presence of glutamic acid oligomers such as Glu-Leu, Pro-Glu, and Val-Glu is not effective in masking the bitter taste [115]. In addition, some dipeptides may indirectly affect the taste characteristics of meat. For example, carnosine and histidine, including dipeptide anserine, destroy unsaturated aldehydic products, reducing the lipid oxidation products and minimizing the rancidity in meat [116].
Studies have investigated the interrelationship between peptides and taste characteristics using various model systems. One such study evaluated the taste of synthesized oligopeptides containing Phe, Tyr, and Leu and found that hydrophobic residues in the peptides function as a bitter taste determinant site. Moreover, the intensity of its bitterness increased when the hydrophobic amino acid with the L-configuration was located at the C terminus and the number of hydrophobic amino acids at the C-terminal increased [117]. In addition, as a result of identifying amino acid compositions and amino acid sequences by separating two peptide fractions from a commercial beef extract as a macromolecular meaty flavor enhancer, it was confirmed that two peptides were composed of collagen and tropomyosin [118]. These results suggested that collagen and tropomyosin are precursors of the macromolecular meaty flavor enhancer. Studies involving other types of meat have identified different strips of amino acids responsible for the unique taste of individual meats. This means that the function of small peptides that affect the taste characteristics of meat depends on the type of meat (i.e., species or muscles).
Free amino acids (FAAs), which are related to improving the taste of meat, show dramatic changes during postmortem aging. Many studies have reported concentrated taste-activated compounds produced during the aging of meat; of these, FAAs in particular, are cited as a major contributor to the taste of aged meat [98]. Dry aging offers a great advantage in this regard as it can promote an increase in the FAA content. This increased FAA content directly increases the flavor of the meat. In addition, as a Maillard reaction and a Strecker degradation substrate, FAAs react to form aroma-active components and affect various taste characteristics [119]. For example, glutamine, alanine, glycine, methionine, and serine are related to sweetness, while leucine, isoleucine, phenylalanine, tyrosine, and valine are related to bitterness. Furthermore, cysteine, methionine, and glutamic acid are associated with umami, while aspartic acid and histidine are associated with sourness [120]. Some amino acids have more than one taste characteristic. Valine has a combination of bitterness and slight sweetness, threonine and lysine have sweetness, slight bitterness, and sourness, and aspartic acid has both sourness and sweetness [94, 120]. As shown in Fig. 3, all these water-soluble metabolites affect the flavor of cooked meat to some extent as precursors to the Maillard reaction or by themselves.
In general, dry-aging of beef increases the content of FAAs such as leucine, phenylalanine, valine, tyrosine, glutamate, and tryptophan compared to wet-aging [119]. In addition, the FAA content increases with the decrease in the moisture content of dry-aged beef; however, FAAs such as glycine, arginine, and alanine decrease with the decrease in moisture content. Therefore, the increase in FAA content in dry-aged beef cannot be entirely explained by the changes in moisture content. Rather, the greater content of taste-active compounds in dry-aged beef compared to wet-aged beef is likely attributable to the concentration effect of moisture evaporation. Studies have shown that the difference in the concentration of metabolites and the rate of protein degradation due to the evaporation of moisture can increase the FAA content [120,121].
Two main mechanisms promote the production of FAAs during postmortem aging: proteolytic enzyme activity and microbial activity. The proteolytic enzymes that cause hydrolysis of proteins include endonucleases (such as calpain and cathepsin) and exonucleases (such as peptidase and aminopeptidase) that release amino peptidase C and H from muscles [122–124]. However, the endogenous enzymes in dry-aged beef can be inactivated with an extension of the aging time. Therefore, further hydrolysis of protein in dry-aged beef may be related to the action of microorganisms in the dry-aged process [119]. In a study, dry-aging of beef for 28 days led to a significant increase in mold distribution from 1.22% to 11.67%, which improved the flavor and tenderness [125]. This is because the growth of mold and yeast during the dry-aging process can induce additional proteolysis of dry-aged beef by activating muscle aminopeptidase and/or proteolytic enzymes [126,127]. The growth of beneficial molds or fungi during dry-aging of beef releases protease and collagenase, and breaks down myofibrillar proteins and connective tissue to improve the taste and flavor of meat.
One of the most notable chemicals in relation to changes in taste of aged meat is nucleotides. In particular, disodium 5-inosinate (5’-IMP) and disodium 5-guanosinate (5’-GMP), the so-called taste nucleotides, have a positive effect on meat taste and umami intensity [128,129]. IMP is widely known to improve the flavor and palatability of meat. The IMP content changes during postmortem aging. Therefore, changes in meat taste during aging are related to changes in IMP content, especially glutamic acid and aspartic acid, which have a synergistic effect on amplifying the umami intensity [15,99,130, 131]. In a study, the change in flavor intensity of high-marbling beef was consistent with the change in umami intensity [122].
The aging method also affects the extent of change in the IMP content. Therefore, the taste of meat varies considerably depending on the aging method. The IMP content in beef decreases rapidly during dry-aging compared to wet-aging [119,120,128]. Dry-aging increases the activity of enzymes related to IMP degradation, reducing IMP content which can negatively affect the taste of meat. Furthermore, in dry-aged beef, hypoxanthine produced by further degradation of IMP increases the bitterness of meat [119]. On the other hand, low-temperature aging not only greatly increases the IMP content but also induces the formation of GMP, resulting in a significant increase in the saltness and umami intensity of chicken and pork. However, the changes in IMP and GMP in cooked beef were found to be minimal or even undetectable [98].
The content of reducing sugars, which provides a desirable sweetness for meat, is lower in wet-aged beef than in dry-aged beef [124]. Beef contains reducing sugars, such as glucose, fructose, and ribose, which are formed by glycolysis and adenosine triphosphate (ATP) degradation [98]. These reducing sugars not only confer sweetness but also react with amino acids to produce volatile flavor components. For example, ribose and cysteine form many sulfur compounds by the Maillard reaction [98]. Maillard reaction refers to the reaction between a carbonyl compound (such as reducing sugars) and an amino compound (such as amino acids or proteins). This reaction produces sulfur and nitrogen compounds, such as pyrazine, resulting in the formation of brown or even black macromolecular substance melanoid or pseudomelanins [110]. The final product of the Maillard reaction varies depending on the substrate and affects the taste of meat. For example, cysteine and glucose mainly produce sulfide, while cysteine and glucose produce more pyrazines and furans under oxidative conditions [132]. Glutathione and glucose have a meat-like taste if they cause a thermal reaction, with or without chicken fat/oxidized chicken fat [133]. With the prolongation of the aging period of beef, the content of two sulfur compounds (methyl mercaptan and dimethyl disulfide) and one pyrazine (2-methyl pyrazine) showed a significant increase [134]. These sulfur compounds and pyrazine have a low odor detection threshold and play an important role in the flavor and taste of cooked beef.
CONCLUSION
Many studies have shown that the aging of meat improves both the tenderness of meat and the taste characteristics by producing taste-related substances. The fundamental mechanism by which aging improves the tenderness of meat involves the operation of the calpain system due to apoptosis, resulting in proteolytic enzyme-induced degradation of cytoskeletal myofibrillar proteins. The improvement of taste characteristics by aging is mainly explained by an increase in the content of taste-related peptides, free amino acids, and nucleotides produced by increased hydrolysis activity. However, the method or conditions of aging greatly influence the improvement of the tenderness and/or taste characteristics of meat. More robust studies on meat aging are required to obtain optimal tenderness and taste of different types of meat.