INTRODUCTION
Deer antler velvet, sourced from the antlers of deer (Cervus canadensis Erxleben), has a fascinating historical backdrop dating back to 168 B.C., as evidenced by tomb scrolls from the Han Dynasty in Nanyang, China. Deer antler velvet’s roots run deep in traditional East Asian medicine, with the Shen Nong Ben Cao Jing documenting its use for diverse ailments since before 2000 B.C. [1,2]. Recognized by the United States Food and Drug Administration (FDA), deer antler velvet comes in various forms—powder, extracts, and spray products. The FDA approval has paved the way for numerous deer antler velvet spray products for external use and dietary supplements, addressing needs such as enhancing sports performance and aiding injury recovery [3,4]. Recent research has focused on understanding the chemical composition, physiologically active compounds, functional lipids, and pharmacological aspects of deer antler velvet, hinting at its potential applications in health and medical therapeutics [5,6].
Delving into the pharmacological attributes of antler velvet has been enlightening, uncovering a rich array of physiologically active elements, including growth factors, hormones, minerals, and proteins. This composition suggests a range of potential physiological impacts, with research primarily focusing on anti-inflammatory, antioxidant, promotion of skeletal development, immune enhancement, and energy-boosting effects [5,7–9]. Ongoing studies are now seeking to discern the potential positive effects of antler velvet on blood circulation, vasodilation, and blood pressure regulation. While these findings hold promise, additional research and clinical trials are crucial for more conclusive insights into the multifaceted effects of antler velvet [10, –11].
However, the effectiveness of deer antler velvet may fluctuate based on drying methods (heat-drying, air-drying, and freeze-drying) [12,13], extraction techniques (alcohol extraction, supercritical extraction, and aqueous extraction) [8,14,15], and anatomical regions of antlers (tip, upper [U], middle [M], and base [B]) [16,17]. This variability poses challenges for standardization, raising concerns about the potential degradation or molecular breakdown of functional substances.
Consequently, this study undertook the intricate task of employing a myriad of processing methods for deer velvet antler, ensuring the utmost standards in both food safety and the stable intake of extracts obtained. Simultaneously, this study assessed the toxicity and immune-boosting properties inherent in deer antler velvet through a comprehensive array of evaluations. This specific experimental endeavor encompassed a diverse array of deer antler velvet extracts. These included fresh antler velvet extracts (FAV), fermented freeze-dried antler velvet extracts (FFA), dried antler velvet extracts (DVA), and naturally shed antler extracts from an aged deer (CFA). Following this generous provision, each antler velvet sample underwent meticulous segmentation into U, M, and B components for further detailed analysis. We used a strategic combination of Caenorhabditis elegans, immunosuppressive mouse models, and immune cells for a thorough examination of toxicity aspects.
The prominence of C. elegans in toxicity studies have been underscored by its not only sharing significant genomic similarities with humans but also exhibiting unmistakable age-dependent metabolic changes and a comparatively abbreviated life span, rendering it a model of choice for expedited toxicity assessments [18–20].
In the evaluation of the immune-boosting properties of antler velvet, we navigate the complexities by incorporating a cyclophosphamide (CPA)-induced immunosuppressive mouse model [21, –22]. This model has been characterized by heightened tumor cell-centric inflammation and a proclivity for immunogenic cell death. Simultaneously, we engaged in co-cultures featuring tumor YAC-1 cells with antler velvet-treated natural killer (NK) cells, revealing a compelling enhancement in the sensitivity of NK cells toward tumor cells [23, 24]. This multifaceted approach not only ensured a comprehensive understanding but also opens avenues for nuanced insights into both the safety and the potential immune-enhancing effects encapsulated within deer antler velvet.
MATERIALS AND METHODS
To standardize the functionality and advance the development of applications for deer antler velvet products, diverse processing methods were applied. Prior to the processing stages, meticulous disease testing was conducted on samples sourced from the Korea RND Nonghyup (Seoul, Korea). Collaborative analyses, in partnership with Yonsei University (Seoul, Korea) and the Chungbuk Institute of Health and Environment (Chungcheongbuk-do, Korea), were conducted to assess deer tuberculosis via polymerase chain reaction (PCR), Brucella through PCR, and chronic wasting disease in deer. All samples exhibited negative results for these three diseases (data not shown).
For this particular experiment, Korea RND Nonghyup generously provided FAV, FFA, DAV, and CFA. Subsequently, each antler velvet sample was segmented into U, M, and B components. Prior to their utilization in the experiment, the samples underwent dissolution in distilled water and filtration.
The C. elegans strain CF512 (fer-15(b26)II; fem-1(hc17)IV) and strain CL4176 (dvIs27 [myo-3p::A-Beta (1-42)::let-851 3′UTR) + rol-6(su1006)] X.) were acquired from the Caenorhabditis Genetic Center (St. Paul, MN, USA) and sustained on nematode growth medium (NGM) plates at a controlled temperature of 15°C. The conventional nutritional substrate for C. elegans, Escherichia coli strain OP50 (OP50), underwent cultivation in Luria–Bertani medium (Miller; BD Difco, Franklin Lakes, NJ, USA) at 37°C for a duration of 24 h with continuous agitation at 225 rpm. As a component of the positive control diet for C. elegans, Lacticaseibacillus rhamnosus GG (LGG) was employed, and LGG was cultured on BD Difco’s de Man, Rogosa & Sharpe (Franklin Lakes, NJ, USA) at 37°C for a period of 24 h. To ensure extended preservation, bacterial cultures were stored at −80°C, supplemented with 15% glycerol as a cryoprotectant. Prior to the start of experimental analyses, OP50 underwent two sequential subculturing procedures.
For the lifespan assay, eggs were extracted from egg-bearing worms using a sodium hypochlorite–sodium hydroxide solution (Sigma-Aldrich, St. Louis, MO, USA) and synchronized to L1 stage worms on NGM plates at 25°C. The young adult L4 staged worms were then transferred to 35-mm-diameter NGM plates seeded with OP50 and the antler velvet extracts (100 μg/mL). Throughout the experiment, all worms were daily transferred to fresh OP50 lawns until the termination of the entire cohort.
To evaluate the motor ability of C. elegans, the number of thrashes in the M9 buffer was recorded. After exposing worms to antler velvet extracts (100 μg/mL) and OP50 for 10 days (n = 10 per group, three replicates), they were transferred to a sterile 35-mm-diameter NGM agar plate without bacteria. The worms were allowed to crawl for a minute to remove aggregated bacteria. Subsequently, another sterile 35-mm-diameter NGM agar plate filled with 1 mL of M9 buffer was prepared. A single worm was introduced into the buffer and allowed to acclimate to the environment. The number of thrashes was then counted for 30 s, with a valid movement defined as the worm bending its head and tail to the same side.
To evaluate the neuromuscular ability of C. elegans, the number of pharyngeal pumps was recorded. After exposing worms to antler velvet extracts (100 μg/mL) and OP50 for 10 days (n = 10 per group, three replicates), they were transferred to a sterile 35-mm-diameter NGM agar plate without bacteria. The worms were allowed to crawl for a minute to remove aggregated bacteria. Subsequently, another sterile 35-mm-diameter NGM agar plate filled with 1 mL of M9 buffer was prepared. A single worm was introduced into the buffer and allowed to acclimate to the environment. The number of pharyngeal pumps was then counted for 30 s under a microscope, with a valid movement defined as rhythmic contractions of the pharynx.
The chemotactic ability of C. elegans serves as a proxy for olfactory behavior. To assess olfactory plasticity, the responsiveness of worms to attractant and repellent chemicals was measured. After exposing worms to antler velvet extracts (100 μg/mL) and OP50 for 10 days (n = 10 per group, three replicates), they were transferred to a sterile 35-mm-diameter NGM plate without bacteria, allowing them to crawl for 1 min to remove aggregated bacteria. Subsequently, another sterile 35-mm-diameter NGM agar plate was prepared with a drop of 100 mM isopropyl alcohol diluted in 100% EtOH (Merk, Kenilworth, NJ, USA) as an attractant, dried on the edge of the plate. Worms were then placed in the middle of the plate and allowed to freely crawl for 30 min, and the number and direction of turns toward the attractant were recorded. The same procedure was repeated using the repellent, 30% octanol (v/v) in 100% EtOH.
Overall, 20 male C57BL/6 mice, aged 10 weeks, were purchased from SamTako Bio (Osan, Korea). Subsequently, the mice were grouped into five individuals per cage and housed under controlled conditions at 23 ± 1°C with 55% ± 5% humidity, following a 12-hour light/dark cycle. Mice were provided with ad libitum access to sterile water and a standard chow diet. A 1-week acclimatization period with the normal diet preceded the commencement of the experimental procedures. Following initial weighing, the mice were randomly allocated into five groups (n = 4 for each group): CONT (vehicle control group), administered orally with phosphate-buffered saline (PBS; 200 μL/day); CPA (negative control group), administered orally with PBS (200 μL/day) and intraperitoneal injection of CPA solution (100 mg/kg/day); CPA + β-glucan (positive control group), administered orally with β-glucan (250 mg/kg/day) and intraperitoneal injection of CPA solution (100 mg/kg/day); CPA + F100 (low concentration treatment group), administered orally with FAV upper extract (100 mg/kg/day) and intraperitoneal injection of CPA solution (100 mg/kg/day); and CPA + F300 (high concentration treatment group), administered orally with FAV upper extract (300 mg/kg/day) and intraperitoneal injection of CPA solution (100 mg/kg/day).
Throughout the experiment, all groups received oral administration for a duration of 28 days, with the CPA, CPA + F100, and CPA + F300 groups additionally receiving intraperitoneal injections of CPA from days 22 to 28. Over the course of the 28-day period, food intake, water intake, and body weight were recorded weekly. The entire set of animal experimental procedures adhered to the protocols and received approval from the Institutional Animal Care and Use Committee of Seoul National University, as indicated by certificate SNU-221025-1.
Blood samples were obtained from the postcaval vein of mice and centrifuged at 1,500×g for 15 min to obtain serum, which was subsequently stored at −80°C for further analysis. The levels of aspartate transaminase (AST/GOT), alanine transaminase (ALT/GPT), gamma-glutamyl transpeptidase (GGT), total cholesterol, and glucose were determined using Embiel GOT, GPT, GGT, Total Cholesterol, and Glucose test kits (Embiel, Gunpo, Korea) to assess the extent of inflammation in the mice.
Fecal samples were systematically collected from each experimental cohort on the day of sacrifice. Genomic DNA (gDNA) was meticulously extracted utilizing the DNeasy PowerSoil Pro Kit (Qiagen, Hilden, Germany). Subsequently, the V4 region of the 16S rRNA genes was selectively amplified with the V4 amplicon primer set (forward: 515F, 5′-TCGT CGGCAGCGTCAGATGTGTATAAGAGACAGGTGCCAGCMGCCGCGGTAA-3′; reverse: 806R, 5′-GTCTCGTGGGCTCGG AGATGTGTATAAGAGACAGGGAC TACHVGGGTWTCTAAT-3′). Illumina® MiSeq sequencing was conducted to acquire high-throughput data. The subsequent bioinformatic analysis of the MiSeq paired-end sequencing data was conducted utilizing Mothur software (v. 1.44.3). Evaluation of bacterial alpha diversity, quantified by Shannon and Chao indices, involved a nonparametric one-way analysis of difference (p < 0.05), the Kruskal–Wallis test, and Tukey’s post hoc analysis. Welch’s t-test was employed to discern differences in the relative abundance of bacterial taxa. The weighted and unweighted UniFrac metrics, calculated based on the operational taxonomic unit table and the phylogenetic tree, facilitated an assessment of bacterial beta diversity. Visualization of the results occurred through principal coordinate analysis (PCoA) plots, portraying distinctive clustering patterns within the fecal microbiome of each experimental group.
The YAC-1 lymphoma cell line obtained from mice, the Raw264.7 macrophage cell line from mice, and the NK92MI NK cell line employed in this study were sourced from the American Type Culture Collection (Manassas, VA, USA). All cellular entities were cultured in Roswell Park Memorial Institute 1640 medium (Gibco, Grand Island, NY, USA), supplemented with 10% heat-inactivated fetal bovine serum (Gibco), 100 U/mL penicillin, and 100 μg/mL streptomycin (Gibco), within a controlled environment at 37°C with 5% CO2 in a humidified incubator.
For assays involving NK cells, YAC-1 and Raw264.7 target cells were individually cultured and seeded in 96-well cell culture plates, with YAC-1 cells at a density of 3.0 × 106 cells/mL and Raw264.7 cells at 1.5 × 105 cells/mL. Once reaching full confluency, NK cells were introduced to the target cells for cocultivation at a ratio of 50:1 (target:NK cell). Prior to the coculture, NK cells were treated with FAV upper extract (100 µg/mL) 24 h prior. Following a 4-hour incubation in a controlled environment at 37°C with 5% CO2, the medium was harvested and subjected to the lactate dehydrogenase (LDH) cytotoxicity assay (Abcam, Cambridge, UK) according to the manufacturer’s protocol.
In the present study, all data points underwent analysis and are presented as the mean ± standard deviation. To discern significant differences, statistical tests, including the Student’s t-test, one-way ANOVA, and two-way ANOVA, were conducted utilizing GraphPad Prism 9.5.0 (GraphPad Software). The significance level for the statistical analyses was predetermined at p values of < 0.05 (*), < 0.01 (**), < 0.001 (***), and < 0.0001 (****).
RESULTS AND DISCUSSION
C. elegans, an extensively utilized genetic model organism capable of mimicking numerous human diseases, holds considerable value for in vivo studies at both metabolic and genetic levels [25,26]. This study employed the C. elegans lifespan assay to scrutinize the toxicity of distinct antler velvet extracts, including FAV, FFA, DAV, and CFA. Initially, we explored the differential effects of various regions within FAV, specifically the upper (FAVU), middle (FAVM), and base (FAVB). Intriguingly, FAVU exhibited the most significant increase in C. elegans lifespan, reaching 21.4% compared with OP50 treatment, followed by FAVM with a 14.3% increase and FAVB with a 7.1% increase (Figs. 1A, 1B, and 1C). Consequently, we opted to proceed with further experiments focusing on the upper regions of antler velvet.
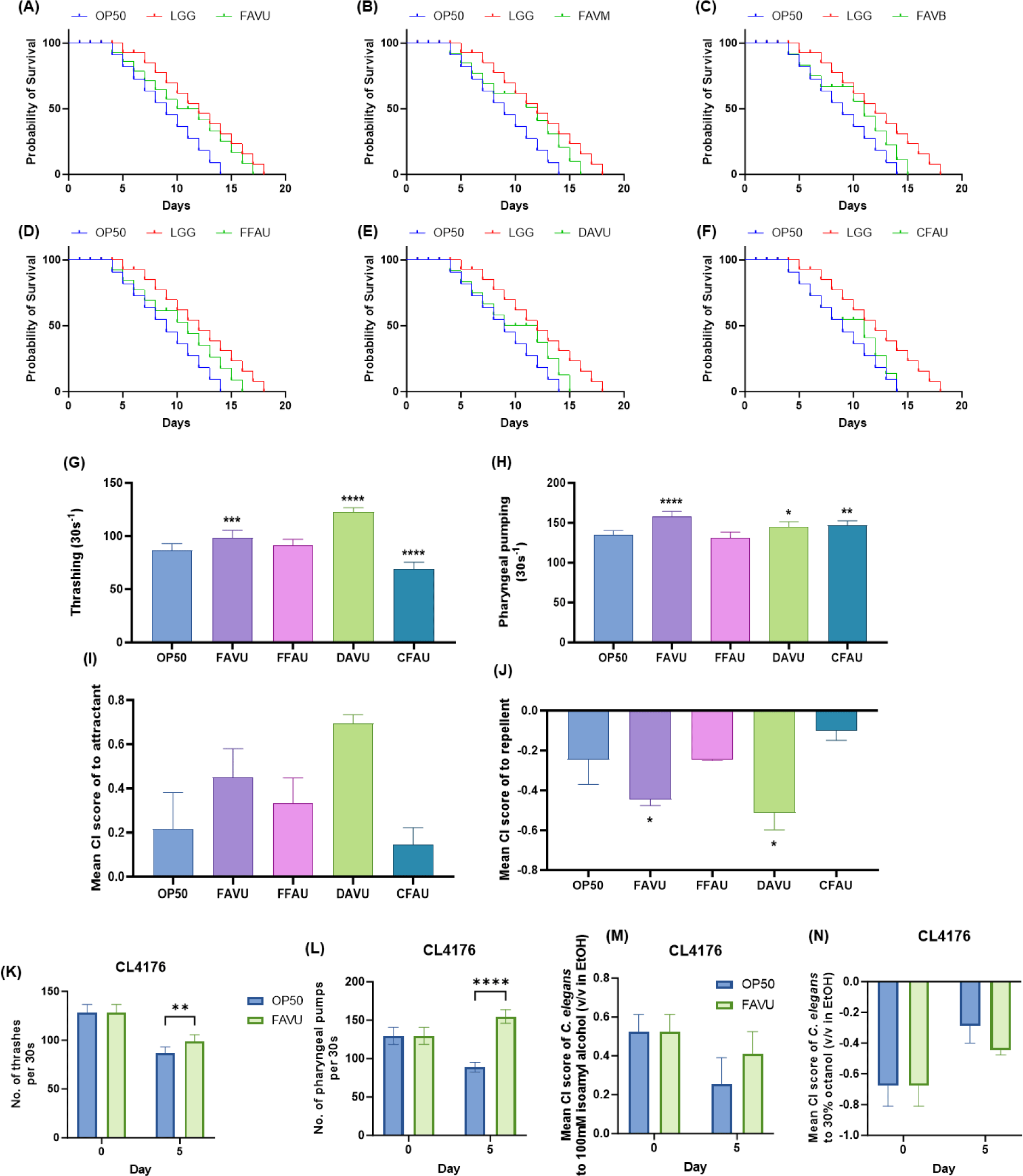
Subsequently, the study investigated distinct effects associated with various processing methods of antler velvet by exposing C. elegansto extracts from the upper region of FAV, FFA, DAV, and CFA. The results indicated that FAVU showed the most significant enhancement in lifespan relative to other methods (Fig. 1A), FFAU displaying the next highest increment at 14.3%, succeeded by dried antler velvet extracts upper (DAVU) with a 7.1% increase, while naturally shed antler extract from an aged deer upper (CFAU) did not present a notable difference in comparison to OP50 (Figs. 1A, 1D, 1E, and 1F). While variations were observed based on the region and processing methods, the overarching outcome highlighted the host-beneficial nature of all antler velvet extracts, with no detectable toxicity.
Furthermore, considering that antler velvet extraction may encompass preventative measures against cognitive impairment and exhibit antiaging effects, this study delves into assessing the neuromuscular and cognitive functions of C. elegans, which are closely associated with metabolic deterioration due to aging [27–29]. The thrashing assay, a valuable tool for identifying motor neuron defects, measured the thrashing frequency of C. elegansin a liquid state. Notably, among treatments with upper antler velvet extracts, FAVU and DAVU significantly mitigated the age-related decline in thrashing, showing improvements of 13.7 ± 8.0% and 41.5 ± 4.6%, respectively (Fig. 1G). Subsequently, the rhythmic pharyngeal pumping of C. elegans, integral to various physiological and behavioral aspects such as circulation and locomotion, was examined [30,31]. These rhythmic activities, categorized as myogenic or neurogenic, showed a general increase in pumping rates among the aged C. eleganstreated with upper antler velvet extracts, notably with FAVU exhibiting a 17.0 ± 4.4% increase, DAVU with 6.9 ± 5.1%, and CFAU with 8.9 ± 4.0% (Fig. 1H). The study further explored the potential direct benefits of upper antler velvet extracts on host cognitive function through a chemotactic assessment of C. elegans. The chemotaxis assay, examining responses to attractants and repellents, proved instrumental in analyzing the functionality of the chemical sensory system or neurons. Enhanced cognitive function and improved brain health assessments were evident in C. eleganstreated with FAVU and DAVU, surpassing the OP50 control groups (Figs. 1I and 1J). In the course of this study, our focus has been on systematically evaluating the holistic toxicity of antler velvet extracts subjected to diverse processing methods on living organisms. Upon a comprehensive analysis of the collective findings in the model organism C. elegans, a diverse range of favorable outcomes associated with antler velvet extracts became apparent. Notably, these extracts manifested a positive impact on the host by augmenting lifespan and ameliorating motor and cognitive functions affected by the aging process. Consequently, our attention shifted toward elucidating the intricate mechanisms underlying the immunoregulatory effects of antler velvet extracts, utilizing a transgenic C. elegansmodel for in-depth exploration.
Expanding on the upper results, we sought to delve deeper into the anti-inflammatory effects of antler velvet extracts, particularly focusing on FAVU, which demonstrated favorable impacts across various parameters. This study aimed to scrutinize the activation status of key gene groups critically involved in C. elegans, especially those associated with neurodegenerative brain diseases. The transgenic worm CL4176, specifically engineered to accumulate β-amyloid peptide, an essential marker of Alzheimer’s disease pathology, was instrumental in this study. Intriguingly, on day 0, no behavioral disparities were noted between the OP50-treated group and FAVU. However, after 5 days of exposure, a significant mitigation of muscular degeneration caused by β-amyloid peptide was observed in terms of thrashing and pharyngeal pumping. Although the chemotaxis assay did not yield significant results, a conspicuous trend indicated that FAVU could protect the cognitive abilities of C. elegans, enabling them to recognize attractants and repellents (Figs. 1K, 1L, 1M, and 1N). This underscores the protective attributes inherent in upper antler velvet extracts, extending beyond safeguarding motor neurons to mitigating impairments in neuromuscular function, sensory neurons, and cognitive capabilities. These alterations suggest that the administration of antler velvet holds promise in alleviating symptoms induced by disease, particularly by modulating the hyperimmune response triggered by the accumulation of β-amyloid peptide in C. elegans. In a parallel context, seeking a more profound understanding of the immunoregulatory impact of antler velvet extracts on vertebrate animals, we conducted experiments employing immunosuppressed mice.
CPA, a widely used chemotherapy and immunosuppressive agent, serves as an alkylating agent to suppress rejection reactions in organ and bone marrow transplants and for treating malignant tumors. However, owing to CPA’s nonselective toxicity, it can impact normal cells, resulting in side effects such as anemia, alopecia, and thrombocytopenia. The potential long-term use-associated concerns prompted an investigation into whether antler velvet’s upper region could mitigate CPA-induced toxicity in mice. The experimental design is shown in Fig. 2A. Notably, CPA treatment led to a significant decrease in body weight gain. By the end of the experiment, the CPA group exhibited a body weight reduction of 11.8 ± 2.0% compared with the normal (CONT) group. Conversely, the FAVU low and high treatment groups demonstrated a recovery in body weight of 2.93 ± 0.75% and 2.89 ± 0.46%, respectively (Fig. 2B). Daily feed and water intake were also significantly reduced in the CPA treatment group, with no notable differences observed between groups (Fig. 2C).
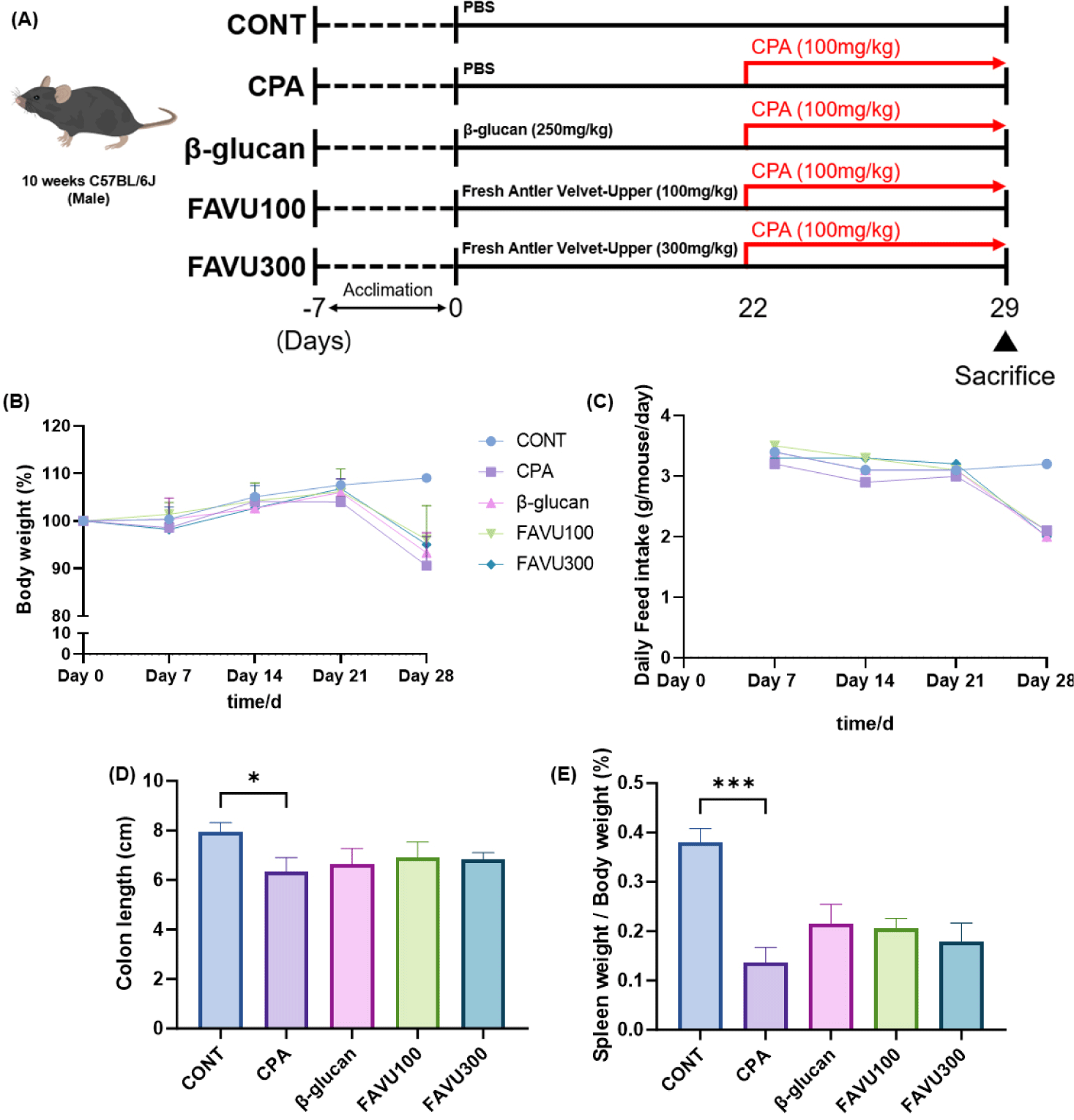
Previous research suggests that a compromised immune system may render individuals more susceptible to infections or inflammatory conditions affecting the colon. Inflammatory responses within the colon can lead to alterations in tissue structure, potentially resulting in a reduction in colon length [32–34]. Additionally, the correlation between immune decline and spleen weight is often associated with the spleen’s role in the immune system. Diminished immune activity can influence the spleen’s response, potentially causing changes in its size or weight. The spleen is integral to filtering blood, eliminating damaged blood cells, and participating in immune responses by generating white blood cells and antibodies. Variations in immune function may impact the demand on the spleen, influencing its size or weight. The monitoring of spleen weight can serve as an indicator of alterations in immune activity or overall immune system health [35,36].
In alignment with this understanding, the administration of CPA to mice in this experiment resulted in a 12% reduction in colon length compared with the normal group, along with a 41.6% decrease in spleen weight. Although FAVU treatment did not yield statistically significant changes, it exhibited a clear tendency toward recovery. FAVU low and high treatment groups displayed increased colon length by 5.4 ± 0.7% and 3.5 ± 0.5%, respectively, compared with the CPA group. Regarding spleen weight, the FAVU low and high treatment groups exhibited an 11.1 ± 0.8% and 8.3% ± 5.7% increase, respectively, compared with the CPA group (Figs. 2D and 2E). Notably, the marginal differences between the FAVU low and high treatment groups suggest that FAVU has the potential to restore the compromised immune system induced by CPA treatment, with even lower concentrations demonstrating a similar recovery effect as higher doses of FAVU.
While the treatment with FAVU did not yield a statistically significant difference in the observed results within this experiment, several factors may account for this outcome. First, the duration of FAVU treatment was comparatively shorter than the conventional timelines associated with general health supplements and dietary regimens [37,38], which typically extend to 12 months or more as of 2007, particularly in the case of health supplements such as vitamins [38,39]. Furthermore, in the course of this experiment, antler velvet extract underwent pretreatment before immunosuppression; however, the overall experimental duration was somewhat extended. This raises the prospect that an alternative response might manifest when administering antler velvet extract subsequent to inducing immunosuppression with CPA.
The relationship between immune suppression and inflammation is intricate and bidirectional. A compromised immune system can lead to inadequate control of inflammatory responses, resulting in chronic infections or prolonged stimuli that trigger inflammation. Conversely, sustained inflammation can detrimentally impact the immune system, potentially causing immune cell exhaustion and contributing to autoimmune disorders [40,41]. Given our previous examination of CPA toxicity, we anticipated an inflammatory response in mice. Therefore, we assessed inflammatory markers in serum and observed an increase in AST, ALT, and GGT levels in the CPA-treated group (Figs. 3A, 3B, and 3C).
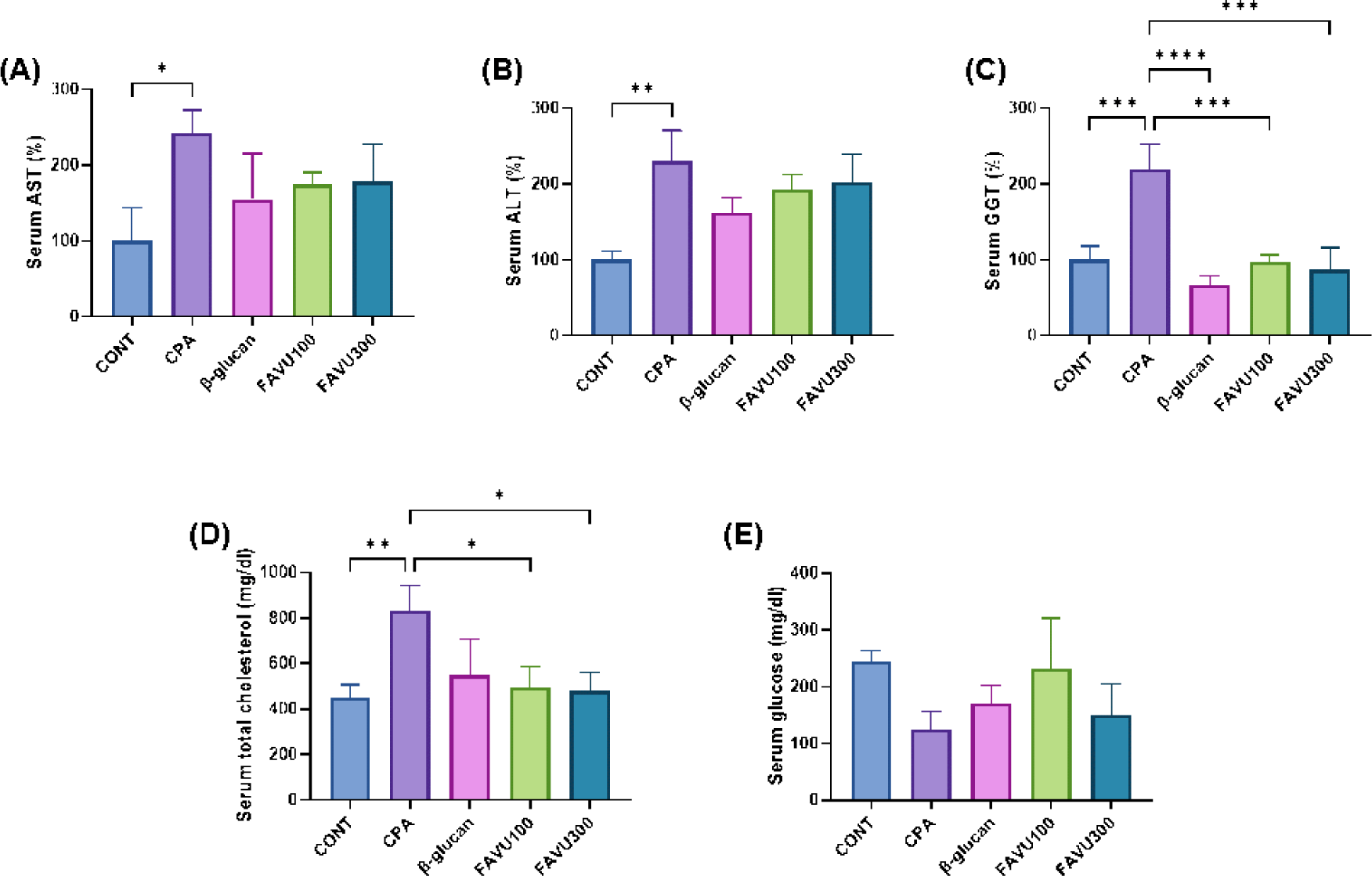
The interplay between AST, ALT, and GGT is often explored in the context of liver function and health. AST and ALT, primarily found in liver cells, exhibit elevated levels in the bloodstream, indicative of liver damage or inflammation. While ALT is more liver-specific, AST is also present in other tissues. GGT, an enzyme found in the liver and bile ducts, has increased levels associated with liver or biliary diseases. Elevated AST and ALT may suggest hepatocellular damage, whereas heightened GGT levels can indicate issues with the bile ducts [42,43]. Although statistical significance was not achieved, low and high doses of FAVU appeared effective in preventing liver damage by restoring inflammatory markers to control levels.
Furthermore, we observed CPA-induced lipid and glucose metabolic disorders in mice. The CPA-treated group displayed abnormally high levels of total cholesterol alongside reduced glucose levels. The interplay between inflammation, cholesterol, and glucose balance is intricate and interconnected. Chronic inflammation can contribute to dyslipidemia, which is characterized by elevated levels of cholesterol and triglycerides. Inflammatory signals may influence the liver’s production of cholesterol and impair the clearance of lipids from the bloodstream. Additionally, inflammation has been associated with insulin resistance, contributing to glucose imbalance and the development of type 2 diabetes. Conversely, elevated cholesterol and abnormal glucose metabolism can themselves trigger inflammatory responses [44–46]. This bidirectional relationship underscores the importance of addressing both inflammation and metabolic imbalances to mitigate the risk of cardiovascular diseases and metabolic disorders. Notably, in our results, FAVU significantly restored total cholesterol and glucose levels, even comparable to the normal control group (Figs. 3D and 3E). This outcome suggests that antler velvet extract has the potential to prevent immune dysregulation induced by CPA treatment by reducing systemic inflammation and restoring energy metabolism.
Subsequently, to assess changes in the gut microbiota composition following the administration of CPA and deer antler velvet extract, fecal samples were collected from each experimental group before sacrifice. gDNA was extracted from the fecal samples, and next-generation sequencing was conducted to analyze the microbial community composition. According to previous studies, there is a bidirectional interaction between inflammation and changes in the gut microbiota, where alterations in the gut microbiota can influence immune responses and inflammation, and conversely, inflammatory states can impact the composition of the gut microbiota [47]. Dysbiosis, or an imbalance in the microbial community, has been linked to increased inflammation, potentially contributing to the development or exacerbation of inflammatory conditions. Proinflammatory responses may, in turn, shape the environment in the gut, favoring the growth of certain microbial species while suppressing others. Understanding this intricate interplay between inflammation and gut microbiota composition is crucial for unraveling the mechanisms underlying various health conditions and exploring potential therapeutic interventions targeting the gut microbiome to modulate inflammatory responses [47–49]. From this result, there was no certain difference between groups in alpha diversity, the richness of each bacterial species, whereas beta diversity, which measures the differences in species compositions between the groups, indicated that FAVU treatment proliferates group-specific bacteria (Figs. 4A and 4B).
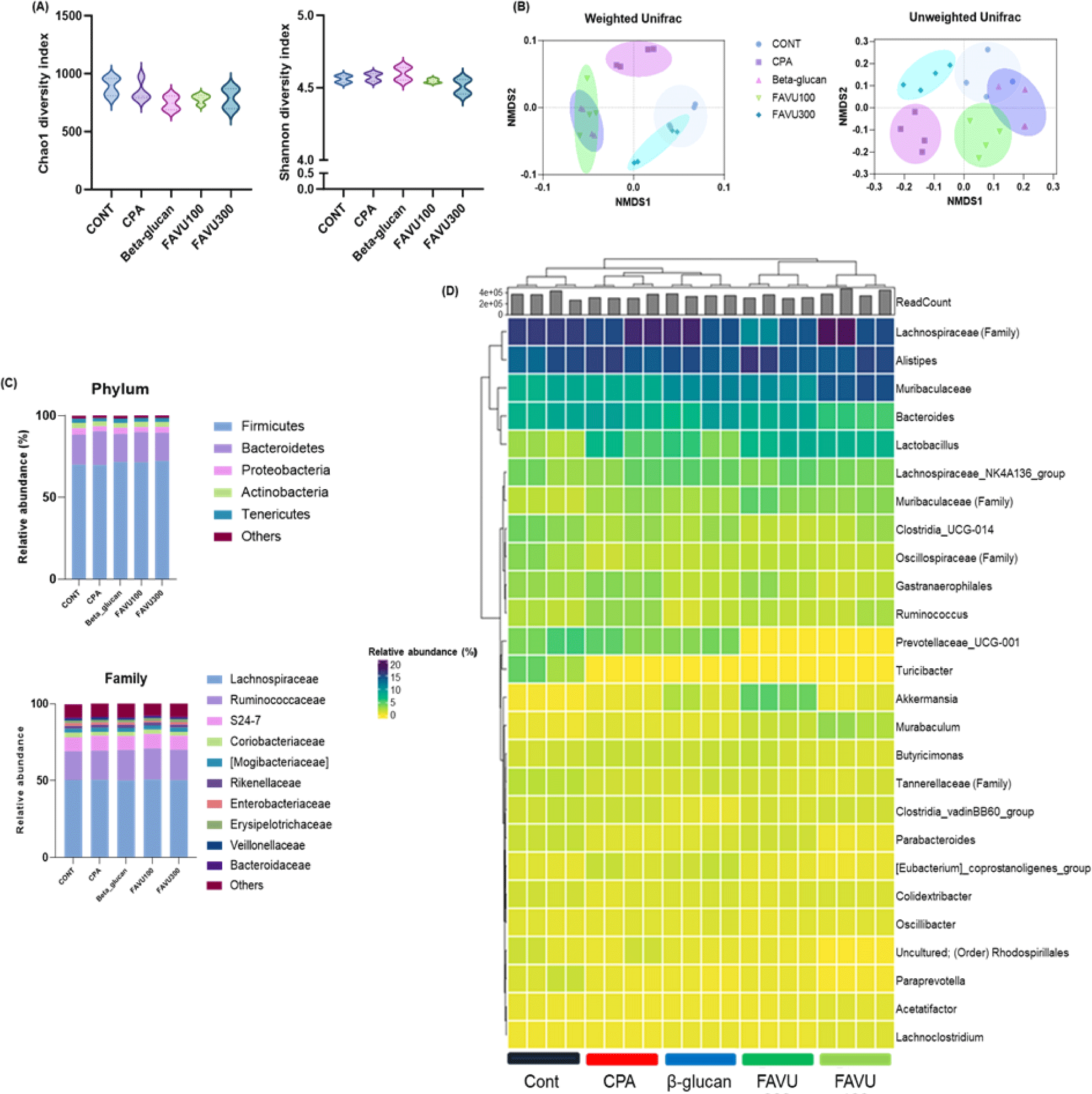
Therefore, examining the specific compositional changes induced by FAVU treatment compared with the CPA group, the administration of deer antler velvet extract demonstrated a general inclination toward an increase in commensal bacteria. This effect was particularly evident in the notable elevation of specific commensals, including Lactobacillus (lactic acid bacteria) [50,51], Akkermansia [52,53], and Lachnospiraceae [51], the established probiotics (Figs. 4C and 4D). These intriguing findings strongly suggest that the administration of deer antler velvet extract not only contributes to the restoration of gut microbiota composition but also actively fosters the growth of commensal bacteria with well-documented probiotic functions. Consequently, the implications are significant, pointing toward FAVU treatment as a promising avenue to counteract CPA-induced immune dysregulation and inflammation by positively shaping the landscape of commensal bacteria. Moreover, this outcome underscores the potential of antler velvet extract as a potent agent to encourage the proliferation of beneficial commensal bacteria, particularly in the context of diseases.
Finally, in the comprehensive exploration of antler velvet extract’s immunomodulatory capabilities, we engaged in a NK cell killing activity assay. This involved the strategic coculturing of YAC-1 lymphoma and Raw264.7 mast cells with NK cells previously exposed to antler velvet extract. Through this coculture study, our objective was to unravel the intricate interactions between NK cells and a commonly utilized target cell line in immunological research. This approach sought to emulate in vitro conditions that closely mimic the natural environment of the immune system [54, 55]. The results, as shown in Fig. 5, exhibited a statistically significant 33.8% ± 3.1% increase in the cytotoxic response of NK cells when they were preincubated with antler velvet extract (Fig. 5A). To delve deeper into the nuances of the antler velvet extract’s impact, particularly its potential cytotoxicity or influence on cell culture conditions, we meticulously compared YAC-1 LDH scores to those of a quiescent Raw264.7 cell line [56, 57] (Fig. 5B). Impressively, the findings underscored the dual nature of antler velvet extract’s immune-regulatory effects. Antler velvet extract not only augmented the cytotoxic sensitivity of NK cells toward the target cells but also maintained specificity toward the normal cell line; in this case, the macrophages were notably held at baseline levels, revealing the sophisticated and targeted immunomodulatory potential of antler velvet extract.
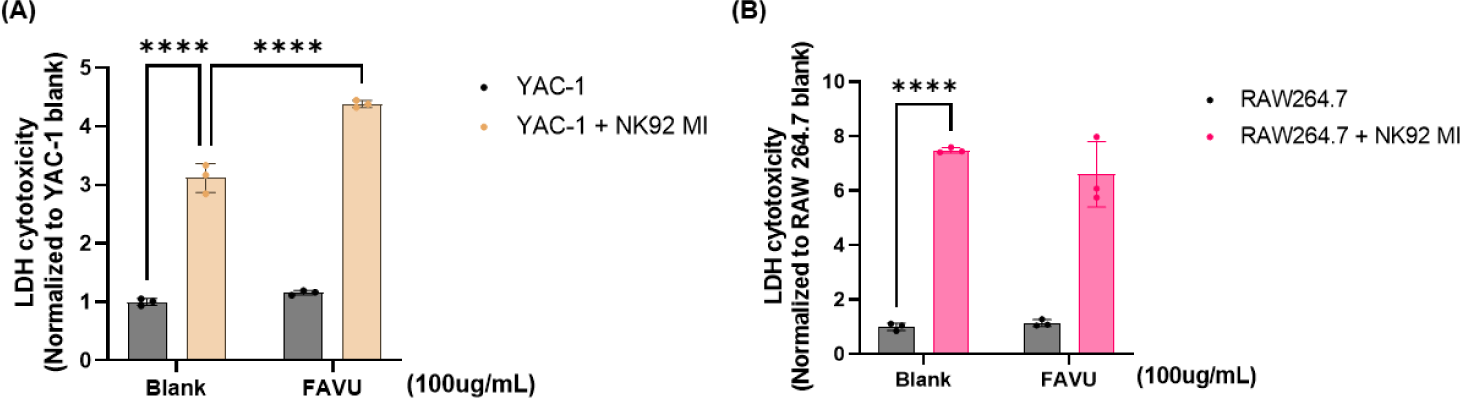
CONCLUSION
The multifaceted study into the effects of antler velvet extract on C. eleganslifespan and associated physiological parameters has provided compelling evidence of its potential therapeutic applications. The meticulous exploration of distinct antler velvet extracts, coupled with an emphasis on the upper regions of antler velvet, revealed a consistent and notable increase in C. eleganslifespan. This outcome underscores the host-beneficial nature of antler velvet extracts, emphasizing their safety profile. Beyond lifespan extension, this investigation explores the muscle and cognitive enhancements induced by antler velvet extracts. The results, particularly regarding the protective efficacy of upper antler velvet extracts against motor deficits and cognitive decline, emphasize their promising utility in fostering general well-being and potentially attenuating age-related neurodegenerative disorders.
Furthermore, the study ventured into the realm of translational research by examining the potential of antler velvet extract to mitigate the toxicity induced by CPA in a mouse model. The results demonstrated a restorative effect on body weight reduction, immune system-related metrics, and inflammatory markers, suggesting the capacity of antler velvet, particularly the upper region, to counteract the adverse effects of chemotherapy. Additionally, the study provided insights into the modulation of gut microbiota composition, revealing the potential of antler velvet extract, specifically FAVU, to foster the growth of commensal bacteria. The robust immunomodulatory capabilities of antler velvet extract, as evidenced by the enhanced NK cell immune response, further substantiate its potential as a comprehensive therapeutic agent. Overall, this scholarly inquiry offers a nuanced understanding of the diverse benefits of antler velvet extract, encouraging continued research to unravel its full potential for human health and well-being.
Nevertheless, it is crucial to acknowledge certain limitations that warrant consideration. First, the study primarily relies on experimental outcomes obtained from C. elegansand mouse models. While these models offer valuable insights into physiological responses, their translational relevance to human systems may vary, and caution should be exercised when extrapolating findings directly to clinical scenarios.
Moreover, the study predominantly focuses on the upper region of antler velvet extract, specifically FAVU, neglecting potential variations in efficacy across different antler regions. The decision to concentrate on FAVU is based on observed trends, but a more comprehensive exploration of various antler regions could contribute to a more nuanced understanding of the differential effects.
Furthermore, the study primarily utilizes in vitro and in vivo assays, providing mechanistic insights into the immunomodulatory and therapeutic potential of antler velvet extract. However, the complex interplay of factors within the human body, including genetic, environmental, and lifestyle variables, may introduce additional nuances not fully captured in controlled experimental settings.